Photovoltaic Contribution to UK Electricity
Posted by Chris Vernon on June 21, 2006 - 2:23pm in The Oil Drum: Europe
Just to get the perspective on this we need to review the current situation. Today we use some 400TWh [1] of electricity per year, generated from the following fuel sources:
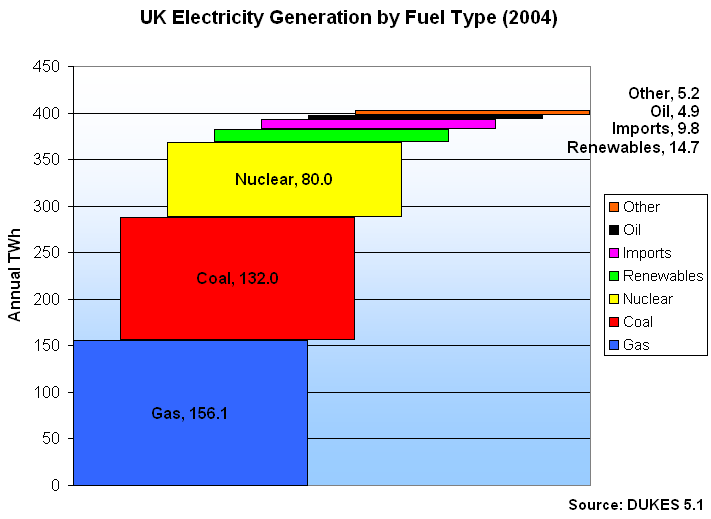
Click to enlarge
Our target for this exercise is to evaluate the feasibility or otherwise of hypothetically replacing half of that, some 78TWh with electricity from photovoltaics and therefore freeing up some 170TWh of gas. This would result in photovoltaics providing about 20% of UK electricity consumption, a similar amount to nuclear today.
Whilst the output from photovoltaics is variable I am not too concerned about integrating this supply into the grid. Although variable it is highly predictable a few hours in advance so a combination of managing fuel burn at remaining gas and coal fired power plants and shaping demand around this variability should be relatively simple.
According to a text book [3] I have here the annual insolation in the UK is approximately 1MWh per square meter of optimally tilted but fixed panel. This then leads to 1,120,000,000 square meters or 1,120 square km of 15% efficient photovoltaic panel being required to deliver the required electricity to meet our hypothetical target. I'll use 15% as a typical polycrystalline cell and a compromise between the more efficient monocrystalline and less efficient thin film cells. Under standard AM1.5 insolation (1000 watts per square meter), such an area would have a peak capacity of 168GW.
These numbers raise a few questions, namely is there room in the country to accommodate such a deployment, could it be built and how much would it cost?
There are several ways to think about the area required; the 1,120 square km above represents 0.5% of the total land area. That figure isn't very illuminating so maybe it helps to compare it to other things. Roads take up 0.9% [4] or inland water 1.3% [5]. Alternatively the area could be considered on a per capita or per house bases. With 60 million people living in ~20 million houses the area works out as 56 square meters per house or a little under 20 square meters per person.
I would suggest the figures above are within the realms of possibility and could be met through a mixture of a dozen or so large industrial solar arrays of a few tens of square km and significant distributed deployment on buildings.
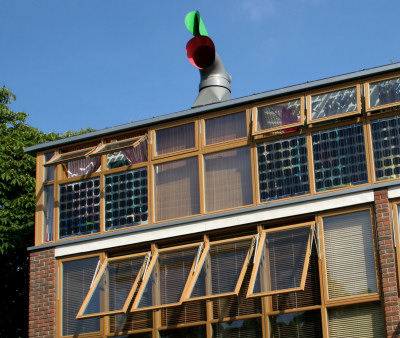
This is a photo I took last year at BedZed showing the photovoltaics in the upper windows with the dual purpose of generating electricity and providing shade. Click to enlarge.
Secondly cost. According to Wikipedia "As of early 2006 average cost per installed watt has decreased from $6.00 to $4.50" [6]. Not a great source but probably accurate enough for this purpose.
Multiplying by the required 168 peak GW installed capacity results in a total cost of $756bn or £410bn. That figure represents ~42% of UK annual GDP, some £6,800 per person or £20,500 per household. Oh dear. To persist with this just a little longer however, it is unrealistic to consider financing this all in one year.
One way of looking at this figure would be to finance it in a similar way to other capital intensive projects. Paying £410bn over 25 years (typical useful life of the solar panel) at an interest rate of 8% reduces the annual cost to just £38.5bn (or £29bn at 5%). This figure looks a little more reasonable, but how does it compare with other sources of energy in the future, specifically the gas whose import we are attempting to mitigate?
At current prices of approximately 2p/kWh [7] the 170TWh we are attempting to mitigate would cost £3.4bn per year. So photovoltaics are currently approximately 10 times more expensive than gas. Prices did spike to 5 and even 6p/kWh last winter, looking 15 or 20 years forward it's not unreasonable to suggest imported gas could be far more expensive than it is today, a three-fold increase taking the average price up to the spikes we experienced last winter would leave the current photovoltaic price less than four times as expensive.
Whilst gas prices are only going up, improved panel efficiency, manufacturing technique improvements and the economies of scale involved in manufacturing and deploying so much photovoltaic infrastructure are sure to reduce costs. To become cost competitive against this expensive imported gas the cost would need to fall to close to $1 per peak watt.
According to a graph at Solarbuzz [8] the costs of system installs have fallen at a rate of approximately 7% per year from 1996 to 2002 in Japan at least, yet retail prices of panels from 2002 to today haven't been falling so fast. In fact recently they have been rising:
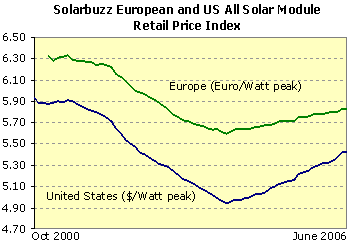
Source: Solarbuzz
If optimistically prices fell by 10% per year it would take 20 years to get down to $1 per peak watt.
The final point on feasibility is manufacturing volume. Globally photovoltaic manufacturing has been growing at 20-25% over the last 20 years. In 2001 350MW were sold, rising to 557MW in 2003 and 927MW in 2004 [9], perhaps a growth rate of at least 30% is a reasonably expectation. We are talking about installing 168,000MW though or 6,720MW per year for 25 years. Global manufacturing capacity is unlikely to reach that level for another 6 years let alone indigenous manufacturing capacity. Indigenous manufacturing would bring secondary benefits of spending the money internally, stimulating the national economy rather than sending the money abroad, ballooning the trade deficit and the increased security of supply over reliance on imported gas involves.
With these assumptions, significant photovoltaic contribution to the electricity supply appears competitive in the medium term against imported gas and obviously in the long term imported gas won't be available at all. However it seems impossible to mitigate any meaningful amount of North Sea depletion over the next decade or so with photovoltaics.
I didn't know how the numbers would fall out of this when I started and whilst they aren't much more than order of magnitude accurate I expect the outcome is reasonable.
In conclusion I think there is the physical space in the country for such a photovoltaic deployment yet they are unable to generate a significant proportion of today's current power consumption due to high cost and limited manufacturing capacity. However that does not mean photovoltaics will never contribute a significant proportion of UK electrical energy.
The numbers are heading in the right direction. In a low energy future, perhaps consuming less than a third of the primary energy we do now and photovoltaic costs falling dramatically (and perhaps moving away from crystalline technology, towards thin film evolutions and onto organic cells) photovoltaics could provide a significant contribution later this century.
It's worth noting that Spain and Italy are also heavily reliant on imported gas, so too would benefit from a partial gas to photovoltaic switch. Their annual insolation is 35-100% more then the UK depending on location, improving the above calculations by that amount.
[1] DUKES 5.1
[2] DUKES 4.1.1
[3] Renewable Energy, Godfrey Boyle, 2004
[4] SABRE
[5] Wikipedia UK
[7] National Grid
[8] Solarbuzz Japan
[9] Solarbuzz Growth
As much as I would like to use PV, I am not sure on the energy EROI involved in making them and how do you make sure you have enough available energy in 25 years time to make the next batch.
Another problem would be land - the panels should be near power lines and access roads and equipment and technological distances between solar batteries will most likely at least double the land requirements.
In conclusion I think it is obvious that to initiate such a program at the current state of the technology would be madness. 38 bln per year is the price tag of good 20-25 nuclear reactors that would replace our whole NG usage just for a couple of years.
This money will offset the upkeep costs and a proportion will have to go towards the purchase of the 'next generation' in 25 years time.
However I think the direction of the question is not so much; "Can we afford to do this sort of thing"
but more
"Can we afford NOT to do this sort of thing.."
If the area Chris describes was made up of (primarily) roofing on houses. If the householder was given tax incentives to install PV / CHP / Small Wind - then all of these really big numbers become divided by millions at a stroke, and then begin to make a difference.
Solar in isolation is not the answer (just like Gas or nuclear...) but increasing the mix is (IMHO) a valid initiative.
Chris's calculation shows that the cost of the solar energy derived this way would be some 20p/kwth. I just added that this cost will likely never come down (absent a technology breakthrough) because of the maintainance and replacement costs. Whether concentrated in large solar farms or subsidised by us taxpayers individual panels on the rooftops doesn't really matter - someone will be paying for it.
Now I think the real question we should ask the people is are they willing to pay 20p/kwth and more? I suspect that the answer will be "hell no". People would accept some small scale solar plants, but once the bills grow to unbearable heights the pressure will grow towards the cheaper sources and there is the chance that solar will be compromised completely. I think something similar is happening in Germany and Denmark which have already gone well down this path.
The bottom line is that it is not the time yet for this - subsidised small scale to stimulate the development of the technology - yes; large scale plants or "rooftop" programs - I don't think so.
However even then other electricity generation techniques may end up being significantly cheaper permanently relegating PV to niche applications.
Nevertheless I support photovaltaics as much as I support nuclear fusion (and maybe even more). And just like with fusion I would object to spend disproportional share of our limited resources for an unproven technology that can turn out to be a dead end.
That's a good point and maybe a fundamental limit of the contribution of a single variable energy source like wind or solar? Peak generation can't really exceed the simultaneous peak demand and probably needs to be quite a bit less for practical reasons (nuke and coal slow start, national distribution limits). Peak demand could certainly be shifted a bit in response to supply and storage through electrolysed hydrogen, although inefficient, would be better than wasting it.
which is for solar thermal traders but is most useful. In Cornwall it is 1.2MWhrs/m²/yr at ideal orientation, and falls as you go north to 0.8 in the Scottish highlands
The thing you most neglect there, Chris, is that 80% of the available energy is in the summer 6 months and 20% of the available energy is in the winter 6 months and in our climate, that is in antiphase to our demand, unlike the southern states of America where there is a summer air conditioning load. This is made worse by the behaviour of the inverters used to convert the DC to AC. These are most efficient at about 80% to 90% of rated power and fall off considerably in dim light. The panels also fall off in efficiency when the angle to the sun increases at the ends of the day and with a low sun in winter as more light is reflected of the front surface.
I have a 2.7kW rated array of Sanyo hybrid monocrystaline/polycrystaline panels in the south a few miles from Brighton feeding at 2.5kW mains connected inverter. They are 21% efficient at cell level and 17% efficient at the DC module level at 1kW/m² insolation. They face south east. Over the last 12 months they have produced 2350kWhr of AC power. This equivalent to 0.87MWhr /rated kW/year which is down from the 1.1MWhr the available energy in the south would indicate. I have had long conversations with Jan Muller the very helpful head of engineering at Jeremy Leggett's very helpful company Solar Century. He says that 0.9MW/kW/yr is the best you can expect at the best sites in the UK
The real shock for large scale application is the variability. Last week I got a record 100kWhr in one week but back in December it achieved 4kWhr in a week, a 25:1 range averaged over a week. The implications of this are trivial while solar generation is only a few percent of total power but enormous if we were to attempt over a 100GW of photovoltaic generation. We would need nearly that much conventional generating power tied up doing nothing much of the summer. In the absence of massive advances in energy storage on a tens of TWhr scale this would rule out pure photovoltaic generation on this scale. However a recent report that I can't find a reference to painted a more encouraging picture of a more modest mixed alternative energy scheme. If I recall correctly Britain is blessed in that a proper mix of solar, wind, tide and wave could supply 30% of generation with less than 5% of conventional backup.
You have also neglected the tilt in calculating the area required. Your figure of over 1000 km² assumes this is tilted up at 30°. I don't think we have that amount of correctly angled south facing hills going spare.
Either with the panels laid flat or tilted up in rows spaced to avoid
mutual shadowing this is going to need over twice this area.
With regard to the factoid that photovoltic systems do not recover the energy used in their construction, Energy Bulletin has an article that nailed the origin of this. The only study that has shown this analysed a large commercial installation that had massive civil engineering work. The energy associated with the concrete exceeded all other energy inputs and took the system into negative net energy. All other studies have shown substantial positive net energy. I don't suppose this will stop this `fact' being endlessly repeated.
There have been two developments that promise to reduce the energy payback time and they are at the opposite ends of the efficiency spectrum. Stacked heterojunctions in tracking concentrator systems have reached an efficiency of 39% at a concentration factor of 236. This means that the semiconductor area is a fraction of a percent of the collected area and little of it is needed. Cheap plastic fresnel lenses concentrate the sun. A study of such a Spanish installation had a energy payback time of 8 months. Nanosolar are building a factory near San Francisco to build 430MW rated worth solar panels per year by printing Copper-Indium-Gallium-Diselenide (CIGS) on a thin flexible substrate on continuous reels. The efficiency is only about 6% at the cell level but the process is so simple and uses so little semiconductor that it promises to be cheaper than silicon and have a much lower energy payback time.
Both systems have some drawbacks as far as the UK is concerned. The concentrators only really work in direct unclouded sun. They have very little output in diffuse sun. The flexible cells require several times the area for a given output and we do not have miles of desert doing very little else.
The rising cost of silicon cells is partly supply and demand but a contribution factor is that we are running out of scrap silicon from the electronic industry. The preparation of semiconductor grade silicon produces large quantities of silicon that does not meet that grade. In the past this has been sold off cheaply to the photovoltaic industry but usage by this has grown much faster than the usage by the electronics industry and silicon now has to be made directly for photovoltaic use.
Some problems with solar panels:
We don't make them. In fact we make virtually zero semiconductors in the UK. So we have to buy them on the open market. I have no idea where Wilkipedia gets its price from, but they are not getting cheaper as there is a rising demand. I have seen them for about £1 a watt in the UK so thats £1 per 150mW realistic output [from the figures at the top]. They were covered by Japanese patents when I was doing EE, but maybe these have expired. Fabricating in the UK will never happen as it's a damn sight more complicated, poisonous, expensive, energy intensive than our govt can grasp. Making semiconductors requires cutting edge production which we have lost from the '50s to the '80s. To start making tonnes of the stuff is just not possible. If every country went down this road, and bought from the existing suppliers, semiconductor makers would quickly become richer than ME oil Sheiks.
Practicality. I would say fit them on your roof ideally. Also why not have them track the sun on gimbals? Either way its problematic. AFAIK [no expert, but I did study semiconductors a little bit as an EE] the output will deteriorate from day 1. I have never seen an output/time curve. Unless someone can show me one I will assume it's a linear deterioration. If it was anything else, the manufacturers would be happy to give it. So for a 20 year life array, I will say you that to get full output at 10 years you need to install twice as many panels at year 1.
There is so much scope to reduce domestic consumption that it is massive compared to all other options. I can guess the electrical industry is dead against low voltage, micropower solutions [such as Ultra bright LED lighting run from a low voltage ring]. It was only last year that they tightened the ratchet on installations with the ridiculous 'part P' electrical code, stopping many competent people from installing wiring.
There is also a huge danger in the UK, which I have never seen mentioned. Any UK residents with a bit of history will understand this. A hundred years ago every house had coal fires to heat our poor insulated draughty housing stock. This worked a treat because of the massive heat output of coal. Then we had 'town gas' extracted from coal and radiant gas fires repaced coal fires. Then the 60s thru 90s, almost all housing was retrofitted badly with gas central heating [cutting into joists for pipes, digging out solid brick walls etc, radiators not quite optimal etc]. This was massively expensive, damaged house structures, lost wall space with radiators, cost a fortune for boilers, pumps, ongoing service and replacement etc and gave only 2 benefits - a timer for control and gas WAS cheaper to run.
2 generations have now grown up with this and it has zero status or novelty value nowadays, only high maintenance costs. If the price of gas stays high why would anyone want to use it anymore for domestic heating? If I was modernising an old house now - electric fires are cheap to buy, easy to install and have timers and thermostats. If the running costs vesus gas are comparable...!!
Some manufacturers have even twigged the demand out there and now make centrally managed electric heaters. If this was to become the next national fad, I could see the grid collapse regularly and electric supply would become an even more interesting game for the next 20 years.
Memo to self - get my wind tubines soon.
I have -20% over 20 years in my head from somewhere... a search turned up this:
I don't think that last comment about extending to 60-80 is right, I bet the solar duty cycle is included in the manufactures figure. Generally 20-25 years seems to be quoted but I don't think that's to total failure though, it's to something like 80% of rated output.
Photovoltaic generation has been around a long time. I remember reading a book about it as a boy 45 years ago. Most of the basic patents will have long since expired. However as in all complex industrial processes there are always a host of patents on minor aspects. Photovoltaic production is fairly simple technology compared to semiconductors. Doing it cheaply is the difficult part. It is no more poisonous and energy intensive that many other industries
We don't have to go to Japan, there is plenty of production in Germany which is now installing more capacity than the rest of the world put together, over 850MW in 2005 out of a world installation of over 1480MW from crystalline silicon and another 176MW by other means (the UK managed 4MW). European producers have about 26% of the market and the Americans 12%
We cannot produce tonnes of the stuff? According to this report in 2005 10,000 tonnes of silicon was produced for photovoltaic use (out of 30,000 tonnes of semiconductor silicon) and another 7,000 tonnes was used from stockpiles from previous years. This year production will increase 30% to 13,000 tonnes but there is nothing left in stock. This will reduce the amount of silicon available and increase the price of polysilicon still further. It has a already increased it from $30/kg to $60/kg. Fortunately cells are being made with progressively less silicon and output is expected to rise this year to 1738MW rated capacity.
However it is estimated that soon the production squeeze will have eased and by 2009 photovoltaic use will have overtaken electronics and be using 27,000 tonnes of polysilicon a year to produce 3400MW of rated generation which with other photovoltaic will bring the total for the year to 4100MW. This will bring total world installed rated capacity to about 15.6GW.
Tracking the sun ideally gives only a 38% increase over an optimally angled fixed panel at UK latitudes and given that the UK tends to be more cloudy morning and evening it is probably less than that. A multi-section tracker on a roof with sections paced to avoid self shadowing will not give more than a fixed panel covering the whole of same roof area. The only advantage is that the total area of the spaced out trackers will be less than the continuous fixed panels. I doubt the gain is worth the cost of the scanners and the reliability problems they might cause. Interestingly the book that I mentioned above and that has stuck in mind suggested building houses that float in a circular pond so that the whole house could turn to track the sun. I wondered then and I wonder still how the sewerage would connect in such a system.
I strongly suspect Chris is right when he suggests that the 20 year live of photovoltaic panels is a cautious under estimate. I have had my system for over 2 years and have monitored it closely. There has been no drop in output at all and I think I could detect a 1% drop off. Space borne systems have survived many years in a vastly more hostile environment than UK roofs.
You say that gas central heating is a problem in the UK. I don't know of many houses that fell down because of such installations. Gas heating is still a lot cheaper than electricity and likely to remain so for many years. As long as most electricity is generated from fossil fuels (and this is not going to change much in the next 15 years) the price of gas and electricity are going to go up together. Domestic combined heat and power is the way to go if you are going to use fossil fuel.
If a solar array is 80% at 20yrs that sounds reassuring. Of course as each PV junction fails what do you do? They are serially connected to give a high output voltage [eg 12V] so water damage of 1 in a chain of 10 renders 10 useless - unless you can field replace them? As an array starts failing do you replace it or add more? Comparisons to spacecraft etc is not relevant as I would think they have invested more thought in design than domestic installation companies.
Even if your cells have a long life, what of batteries and inverters to generate AC? These have a hard life and will need replacing.
A Google on 'Wrexham Sharp Solar' gives some info:
www.prometheusinstitute.org/admin/ researchnotes/uploads/PV2-0301.08_-Sharp_Solar-.pdf
highlight:
Employs 63 people [in 2004] - produces 40MW of modules made of mono and polycrystalline cells made in Japan.
We basically gave up making semiconductors in the UK in the 1980s, and are therefore hostages to the fortunes of other hi-tech countries. This was something UK govts have never admitted. One of your links, Nick:
http://www.renewableenergyaccess.com/rea/news/story?id=41508
says SGS is a manufacturer. Thats the French. So its French nuclear or solar right?
"I have had my system for over 2 years and have monitored it closely. There has been no drop in output at all and I think I could detect a 1% drop off."
Not necessarily. The end output seen is that of the inverter, which regulates the output voltage. If cells deteriorate it is simply the maximum current available which decreases. I have no knowledge how cells do deteriorate, but I know it takes a lot of them to make a useful amount of power.
"..building houses that float in a circular pond so that the whole house could turn to track the sun..."
If you were close to a lot of water a heat pump could give you year round climate control.
"You say that gas central heating is a problem in the UK. I don't know of many houses that fell down because of such
installations. Gas heating is still a lot cheaper than electricity and likely to remain so for many years. As long as most electricity is generated from fossil fuels (and this is not going to change much in the next 15 years) the price of gas and electricity are going to go up together. Domestic combined heat and power is the way to go if you are going to use fossil fuel."
I have owned 3 old terrace houses which have all had gas central heating retrofitted. All had notches cut into joists to accomodate pipes. I doubt if any followed these guidelines:
http://www.worthing.gov.uk/Planning/BuildingControl/TechnicalGuidanceNotes/19NotchingDrillingofTimbe rJoists/
I think domestic gas heating could be disastrous if the price of gas rises. The total ownership cost and hassle of gas is much higher than electric heating. If domestic gas prices narrow versus electric..switching to electric is as easy as a £10 fan heater from Argos.
cheers all
I've recently bought a house which has no gas connection. Due to the insane capital cost of connecting to the gas main (just outside in the street) plus installing Gas central heating we're quite happy to run all electric heating/cooking. Plus we've recently added air conditioning as south west England gets quite hot these days.
First I'd like to take to task all those that wish to remove the so-called perverse pricing incentives for increased consumption. We have cheaper electricity at certain times of the day and night to allow us to economically run off peak heating systems in the winter and air con during the summer. This is simply demand led pricing, we get cheaper power off peak because the power companies are trying to stimulate demand (or push some of the variable demand to off peak times eg washing machines/tumble driers).
In our situation we certainly don't get cheaper power if magically double our consumption. The cost benefits are there to incentivise us to help balance the load on the grid and allow us to heat our home affordably. Even at 4.5p/kWh the power co are still making money.
The suggestion of allowing each house cheap power up to a certain value for heating/cooking lighting and then increased power thereafter has merit, but who gets to decide what a reasonable level is. It would vary massively for different values of insulation/efficiency. Lets not forget that here in the UK we're cursed with a huge amount of 100+ year old housing stock, which isn't about to disappear anytime soon. Laughably much of our very recent housing stock is just as inefficient as the stuff built just pre and post WW2. I should know, my house is but 20 years old and is draughtier and less well insulated than my parents 1930's semi detached. Where I live it seems modern housing is built down to a price, not up to a standard.
So how do you decide what is required for a given house for its base load heating/cooking. What about size? Would you get a bigger allowance for more sq meters? You'd certainly need it for a large family home vs. a small starter flat.
The whole scheme is just ripe for political interference, with extra "base" allowance being granted if you're "poor", on benefits, an asylum seeker, labour voter etc etc. Soon those who can be squeezed for extra cash will end up subsidizing those voted in a certain way. Don't think this will happen? It already is with council tax. More central govt. cash making its way to "favoured" areas, with other areas having to increase council tax to cover costs. Unsurprisingly favoured areas happen to be mainly Labour controlled. I would not be surprised in the slightest if the opposite happened under a Conservative govt. Both sides are as bad as each other.
No, if anything the energy markets should remain free of this kind of political interference. Price should be set by the open market. The Govt. role should be limited to long term strategic policy making to encourage efficiency and encourage new gen. capacity to be built in a way which will not leave the UK venerable either economically or socially. We will not be able to compete with other economies in the next 30 to 50 years if we make the wrong decisions now. Further power outages will make UK plc a very unattractive place for business if allowed to occur, so to say that we will have to curb demand is slightly naive. Sure, efficiency can help, but to expect industry to simply switch off to help reduce carbon emissions/reduce fuel consumption is not going to happen. Industry will simply relocate elsewhere.
If we're not careful that elsewhere will be France, with its nice stable nuclear capacity.
We need a massive investment in domestic energy conservation and efficiency in the UK as it is the cheapest way of meeting future energy needs. With market mechanisms this requires a high marginal cost of energy to the end-user. To avoid undesirable effects such as poorer people freezing to death in winter, the best approach is to provide a quota of cheap energy per household (less than average use), and higher-priced energy beyond that.
Since we want individuals to find the most energy-efficient way of living (including changes in household size), this should be based per capita, without allowance for larger houses etc. A poor person with a large house can insulate it better or sell and buy a smaller more efficient building. This will favour more energy-efficient housing with micro-power.
This ties in neatly with carbon credits as a form of cheap energy support. There could be 1 credit per adult, plus 0.5 for each dependent child in the 6-16 age range. The government should distribute the carbon credits among the citizenry, who can sell them to their energy suppliers or use them to offset other energy-intensive tasks. The quotas and energy rates should be set so that a typical family taking reasonable energy conservations steps comes out revenue-neutral.