The Solar Envelope: How to Heat and Cool Cities Without Fossil Fuels
Posted by Rembrandt on April 13, 2012 - 12:02pm
Architects all over the world have demonstrated the usefulness of buildings which are heated and cooled by design rather than by fossil fuel energy. What has received much less attention, however, is the possibility of applying this approach to entire urban neighbourhoods and cities.
Designing a single, often free-standing, passive solar house is quite different from planning a densely populated city where each building is heated and cooled using only natural energy sources. And yet, if we want passive solar design to be more than just a curiosity, this is exactly what we need. Modern research, which combines ancient knowledge with fast computing techniques, shows that passive solar cities are a realistic option, allowing for surprisingly high population densities.
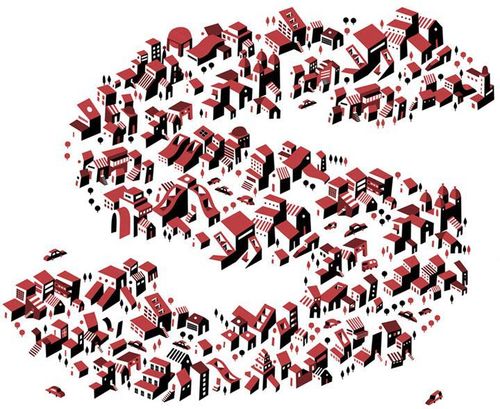
Passive solar design requires the knowledge to design and orientate buildings so that they can be heated by the sun. Coupled with other low-tech solutions such as thermal underwear and oven stoves, passive solar design could all but eliminate the use of fossil fuels and biomass for heating buildings throughout large parts of the world. Indirectly, a passive solar house can also cancel the energy requirements for cooling and ventilation (passive cooling), and for lighting during the day. Of course, passive solar buildings can be outfitted with solar water heaters and PV solar panels, further reducing the use of unsustainable energy resources.
Passive solar design does not involve any new technology. In fact, it has been around for thousands of years, and even predates the use of glass windows. For most of human history, buildings were adapted to the local climate through a consideration of their location, orientation and shape, as well as the appropriate building materials. This resulted in many vernacular building styles in different parts of the world. In contrast, most modern buildings look the same wherever they stand. They are made from the same materials, they follow forms that are driven by fashion rather than by climate, and are most often randomly located and oriented, indifferent to the path of the sun and the prevailing wind conditions.
Modern buildings rely on a massive supply of cheap fossil fuels for heating, cooling, and lighting. Take the supply of cheap fossil fuels away, and they become completely uninhabitable for most of the year: they are too cold, too hot or too dark. This radical change in architectural design was caused by both the arrival of cheap and abundant energy sources and the resultant urbanisation (See part 2). The Industrial Revolution relocated millions of people from the countryside to the cities. When most of us lived and worked on farms or in hamlets, it was fairly easy to orientate one's house towards the sun. In an urban environment, however, building orientation is generally determined by street layout, and one building can easily overshadow another. High-rise buildings further complicate solar access.
From solar oriented buildings to solar oriented cities
This does not mean that passive solar design could not be applied to entire cities. It just takes more sophisticated planning. Solar access to an individual building is determined by only four factors: latitude (the distance north or south from the equator), slope, building shape and orientation.
Solar access to a city (or any other built-up environment) is determined by seven factors: the four just mentioned, plus the height of the buildings, the width of the streets, and the orientation of the streets (See part 2). Providing ventilation in an urban environment is determined by the same factors, with the exception that latitude is replaced by prevailing wind conditions.
While most research in passive solar design during the 1970s was directed at individual buildings, one man began forty years of research into solar oriented cities: Ralph Knowles, professor emeritus at the USC's School of Architecture and author of three fascinating books on the topic (1974, 1981
, 2006
).
Knowles developed and refined a method that strikes an optimal balance between population density and solar access: the "Solar Envelope". It is a set of imaginary boundaries, enclosing a building site, that regulate development in relation to the sun's motion -- which is predictable throughout the seasons for any place on Earth.
Buildings within this imaginary container do not overshadow neighbouring buildings during critical energy-receiving periods of the day and the season, and assure solar access for both passive and active solar systems. On the one hand, the solar envelope allows architects to design with sunlight without fear that their ideas will be cancelled out by future buildings. On the other hand, the solar envelope recognizes the need for development and high population densities, by defining the largest container of space that would not cast shadows off-site at specified times of the day.
Knowles and his students have reached densities that are far above the average in European and American cities (See part 3), with the exception of high-rise centers such as Manhattan.
Modification of traditional zoning practices
The solar envelope is actually a relatively simple modification of existing zoning practices, which also set imaginary boundaries that enclose a building site -- determining the maximum height, width and depth of future buildings. The most rigid approach in conventional zoning prescribes maximum building heights, set in feet or metres, number of floors, or both. A second, more flexible approach, sets limits based on a ratio between developeable land and floor area within the building on that site. For example, a floor-to-area ratio (FAR) of 6 means that architects can develop 6 times the developeable square footage of land within the setbacks. They could cover the entire site with 6 stories, or cover only half of the site with 12 stories, for example.
Although both zoning methods offer a certain degree of solar access in a city, they are far from optimal. The main problem is that they do not design building orientation with its solar impact in mind, which can be as critical as building height. For example, a skyscraper with its broad flat sides facing east and west will cast a relatively small midday winter shadow, while one oriented with its broad flat sides facing north and south will shade a much larger area during the sunniest periods of the day (illustration above). Taking orientation into account would greatly improve solar access for surrounding buildings, without sacrificing housing density.
The geometry of the solar envelope
Compared to conventional zoning practices, the solar envelope produces a different geometry -- the limits of the envelope derive their vertical dimensions from the sun's daily and seasonal movements. Thus, while conventional zoning envelopes are shaped like a box, the solar envelope has both vertical and sloping spaces.
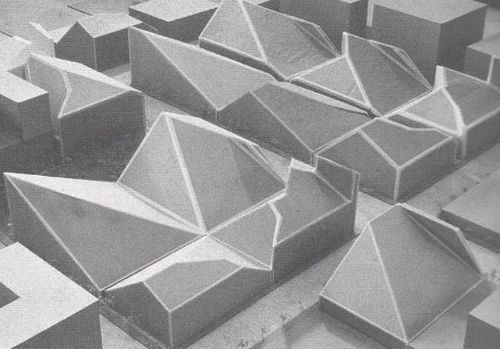
As a result, the buildings and city blocks that fill these imaginary solar envelopes are more likely to have unique shapes. One side of a building would not look like the other, nor would each side of the street. In the northern hemisphere, development would tend to be lower on the south side of a street than on the north where a major southern exposure would be preserved. Streets take on a directional character where solar orientation is clearly recognised.
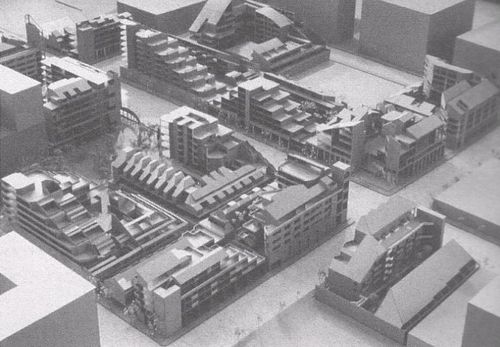
Adjacent buildings can meet each other gently, rather than abruptly, across property sidelines. Tall buildings would group together at the site's southwestern end, and those of moderate height at the northeastern end, with the shortest buildings taking up the site's midsection. Buildings on corner lots will be taller because their shadows can extend accross the street in two directions instead of one.
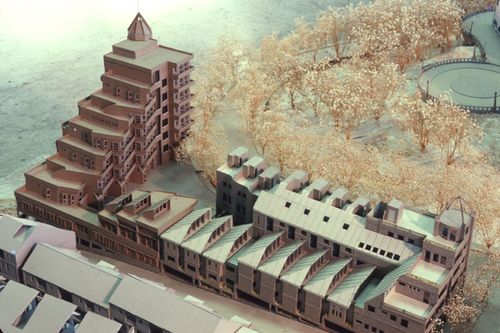
Within the solar envelope, certain architectural characteristics have great consistency. For instance, roof terraces appear where the sloping sides of the envelope intersect the rectilineair geometry of buildings. Courtyards are another crucial element, as they introduce sunlight and heat to deep interiors. Clerestories allow for the penetration of winter sun down stairways to enliven otherwise darker, lower floors. Sunscreens and porches are everywhere, keeping the sun out in summer.
Defining solar accessThe solar envelope is not only defined by the path of the sun, but also by fixed parameters set by the designer. Choosing these will determine the balance between solar access and development potential.
The most important choice is the definition of the hours during which we want to avoid casting shadows on adjacent land -- the 'cut-off times'. The longer the period of daily solar access, the smaller the developeable volume under the envelope. Obviously, setting the cut-off times as equal to the period between sunrise and sunset would not work, because in that case few or any buildings could be constructed. For passive solar design, a minimum of 4 to 6 hours per day in winter is considered practical, depending on the climate.
The duration of solar access could also be set by a minimum percentage of available energy instead of determining a minimum hours of sunshine. In that case, cut-off times would change over the course of the year. Another parameter to be set is the 'shadow fence'. It determines the minimum height to which solar access has to be assured; for instance zero, 3 or 6 metres above street level. For example, one can choose to allow shadowing of garages and shops in order to improve the density under the solar envelope.
What about existing buildings?
Solar envelopes can be designed for individual buildings or as a single envelope for a group of houses, a neighbourhood, a district or even an entire city. This is a rather straightforward process when a site is being designed from scratch, but often current buildings will complicate the generation of a solar envelope. When the solar envelope is applied in line with existing buildings, new construction would always be shaped and proportioned with reference to the old. Each new phase of development changes the surroundings and thus the context within which the next envelope is generated.
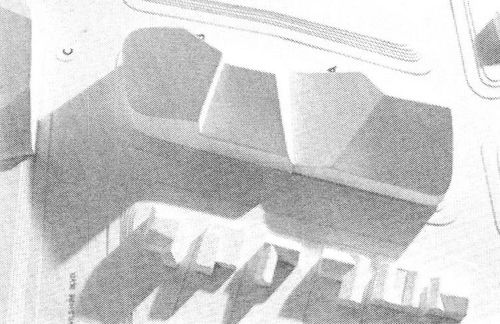
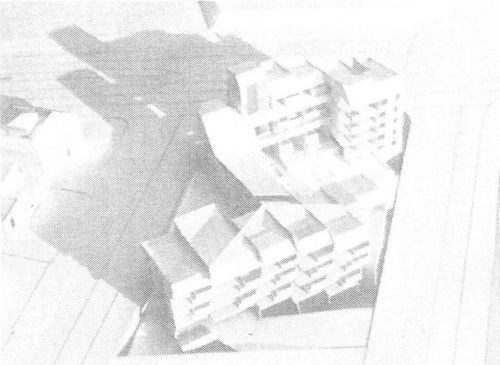
It is important to note that the solar envelope only protects neighbouring properties. It is the architect who must ensure solar access to the buildings within the envelope, tackling problems of overshadowing within the envelope itself. For larger sites, the volume of a solar envelope is therefore larger than the volume of the buildings that actually fill it, at least when solar access is assured to all dwellings on site.
Solar oriented cities in Antiquity
Knowles' research draws on ancient knowledge, most notably the solar planned cities in Ancient Greece and the solar communities of the Ancient Pueblo People in what is today the Southwestern United States. The Ancient Greeks built entire cities which were optimal for solar exposure.
In the fifth century BC, for example, a neighbourhood for about 2500 people was built in the city of Olynthus. The streets were built perpendicular to each other, running long in the east-west direction (the horizontal streets shown in the plan), so that all houses (five on each side of the street) could be built with southern exposure.A gridirion street plan oriented at the cardinal points was not new at the time, and neither is it proof of a design aimed at maximum solar exposure. But the Greeks did more. In "A Golden Thread: 2500 Years of Solar Architecture and Technology", Ken Butti and John Perlin note that all houses were consistently built around a south-facing courtyard:
"The houses that faced south on the street and south to the sun were entered through the court, straight from the street. The houses that faced north to the street and south to the sun were entered through a passageway that led from the street through the main body of the house and into the court, from which access was gained to all other spaces."In keeping with the democratic ethos of the period, the height of buildings was strictly limited so that each courtyard received an equal amount of sunshine:
"In winter, rays from the sun traveling low across the southern sky streamed across the south-facing courts, throgh the portico, and into the house - heating the main rooms. The north walls were made of adobe bricks one and a half feet thick, which kept out the cold north winds of winter."
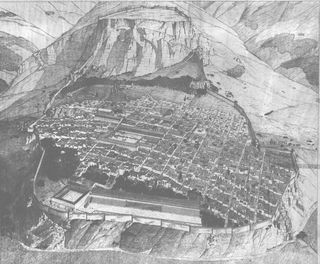
Another obvious example of Ancient Greek solar planning was Priene (illustration above), rebuilt in 350 BC and located in present-day Turkey. The city had about 4000 inhabitants living in 400 houses. Its buildings and street plan were similar to those in Olynthus, but because the city was built on the slope of a steep mountain, many of the fifteen secondary streets (running north-south) were actually stairways. The seven main avenues were terraced on an east-west axis.
Native Americans
The Ancient Pueblo People or "Anasazi" built a number of sophisticated solar oriented communities during the 11th and 12th centuries AD in what is now the Southwestern United States: Long House at Mesa Verde, Pueblo Bonito in Northern Mexico and the "sky city" of Acoma.
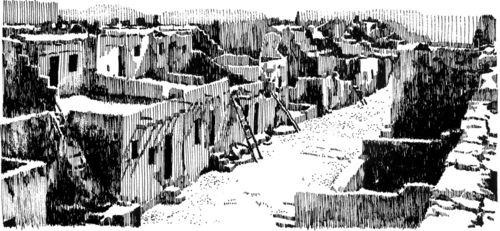
These communities followed a different building style than that of the Greeks. The Ancient Pueblo People constructed terraced buildings of up to three floors high. These were buildings that would fit perfectly in a solar envelope with slanting lines. Acoma pueblo (illustration above) is one example of these orderly, solar planned communities. It consists of three rows of houses built along streets running east and west, so that each building faces south. The streets that separate the houses have a width that allows winter shadows to cover the whole of the adjoining street, stopping just before the following row of buildings.
Heliodon
Knowles' research combines the best elements of these historical designs and incorporates modern technology that greatly facilitates the generation of a solar envelope. The heliodon, invented in the 1930s, is a contraption that creates a geometrical relationship between an architectural scale model and (a representation of) the sun. More recently, software versions of the heliodon have made the technology much more affordable, while allowing for the fast generation of even very complex solar envelopes.
On larger sites in particular, and when already existing buildings complicate the generation of a solar envelope, the available computer software saves time and can result in more building volume.
The Ancient Greeks resorted to passive solar design mainly because of acute firewood shortages, but there was an additional reason: they believed that solar heat was good for human health. When solar access in cities regained attention in the western world during the urbanisation of the nineteenth and early twentieth centuries, health was the one and only motivation.
The Industrial Revolution brought plenty of cheap energy, but also plenty of disease. Millions of people ended up in overcrowded buildings in narrow streets. These neighbourhoods were soon ravaged by lethal epidemics such as tuberculosis, cholera, smallpox and typhoid fever. Most medical experts were convinced that these illnesses were caused by a lack of fresh air and sunshine.
In his 1893 book "Healthy Hospitals", Douglas Galton wrote:
"Second only to air, is light and sunshine essential for growth and for health; and it is of Natures most powerful assistants in enabling the body to throw off those conditions which we call disease. Not only daylight, but sunlight; indeed, fresh air must be sun-warmed, sun-penetrated air. The sunshine of a December day has been recently shown to kill the spores of the anthrax bacillus."
In 1854, Richard Snow famously observed that an outbreak of cholera could be traced back to the drinking of contaminated water at a public well, proving that the most devastating disease was not caused by foul air or a lack of sunshine but by germs in drinking water. However, it would take half a century more before his theory was generally accepted. Furthermore, some ailments were indeed cured or alleviated by sunshine and fresh air. For example, rickets, a bone deforming disease endemic among children in nineteenth-century cities, was caused by a lack of sunshine.
At the turn of the century, doctors in Europe and in the US began promoting sunbathing to help prevent the disease. Interestingly, while Knowles initiated his research into the solar envelope purely for means of energy conversion, he gradually changed his priorities, aiming to improve the quality of life instead, of which he considers energy conversion to be just a part (see his latest book in particular).
Solar access in nineteenth-century cities
In order to ensure solar access and fresh air, many cities in Europe and in the United States enacted a variety of building codes between the 1850s and the 1930s. Although Knowles does not talk about this period, it is interesting to compare these earlier zoning practices to the solar envelope. Most of the building codes were related to building height and street width. Boston architect William Atkinson, who was one of the advocates of passive solar design at the time, saw the limitations of these codes and pointed out that building shape was equally important -- controlling it could actually improve development potential and solar access alike.
In his 1912 book "The Orientation of Buildings, or Planning for Sunlight", he writes:
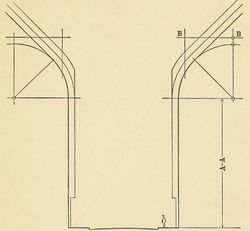
"The method of limiting the height of buildings by a horizontal plane, either at a fixed height, or at a height proportional to the width of the street, is simple in application but is not scientific, since it assumes that what is the proper height for the front wall or facade is also the proper height for the rear portions of the building."
"Whereas, as a matter of fact, the rear portions may well be allowed to rise to a greater height, in proportion to their distance back from the street line. The height of the building should be limited by a slanting line drawn from the opposite side of the street at a certain angle."
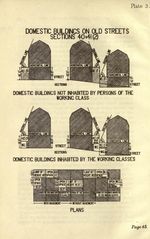
Atkinson was inspired by the 1902 building laws of Paris (an adaptation of the original building codes made by Haussmann), which not only contained rules about street width and building height, but also introduced a code regulating building shape. The illustration above (taken from Atkinson's book) shows that the facade of a Paris building (the vertical A-A line) could not be higher than 20 metres, while the attics above (of which the height was also determined by street width but could not surpass 10 metres) were curved.
This made it more likely for sunlight to penetrate the lower parts of the building on the other side of the street, while maximizing housing density. England's capital had somewhat similar building codes since the 1894 London Building Act, though prescribing slanting roofs instead of curved ones (illustration on the left). The tiered skyscrapers that appeared in the US following the 1916 Standard Zoning Enabling Act also regulated building shape, although the sheer height of the buildings was unfavourable for solar access.
The Barcelona Eixample
The most interesting example of nineteenth-century solar city planning is the Barcelona "Eixample" (Catalan for "enlargement" or "expansion"), designed by Ildefons Cerdà i Sunyer. The Barcelona Eixample (home to the Sagrada Familia and other famous Gaudí monuments) can be considered the largest solar-planned neighbourhood in existence. Moreover, its history exemplifies the tension between solar access and developmental needs.
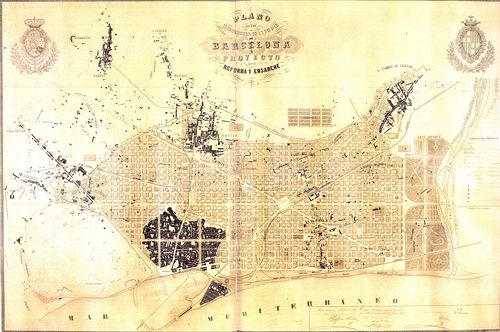
Unlike Baron George-Eugène Haussmann in Paris, Cerdà did not have to demolish Barcelona to adapt the city to the massive immigration flow of the period. Medieval Barcelona (the large dark blob on the map below) was surrounded by a large open plain with only a few small villages at the outskirts (also in black). In the 1850s, Cerdà designed a large 'checkerboard' street plan surrounding the old city centre and annexing the periferial towns. The neighbourhood, which today has an area of 7.46 km2, consists of streets 20 metres wide (65.5 ft), intersected by a few boulevards 50 metres wide (164 ft), and very large city blocks measuring 113 x 113 metres (370 x 370 ft).
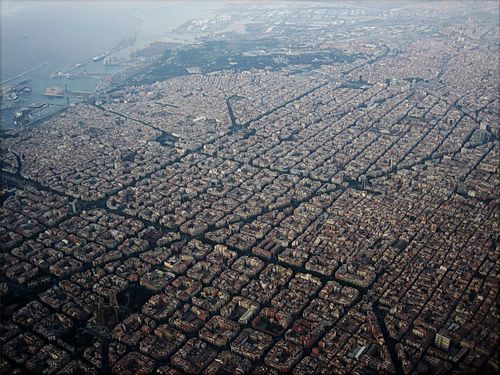
Cerdà intended to maximize solar access (and ventilation) to every apartment in four ways. Firstly, he limited building height to 16 metres (52.5 ft) for streets 20 metres wide. Furthermore, he mandated that city blocks could only be built up on two instead of four sides, either parallel to each other or in the form of an L (illustration on the right, below). This enabled the creation of large interior spaces and introduced sunlight and fresh air at both sides of each building.
Thirdly, all city blocks have truncated corners, further improving solar access. Lastly, he decided not to lay the street grid on the cardinal points, but diagonal to it. As we will see shortly, this gave all apartments access to sunlight during the day, while offering all streets shadow throughout the day.
Only the truncated corners and the orientation of the streets survived one hundred and fifty years of history. Cerdà's plan received much criticism at the time. The main reproach was that the design wasted too much valuable building space and thus money.
Within years, all four sides of every octagonal city block were built up. Even most of the smaller interior spaces that resulted from this were filled, albeit with low buildings so that solar access at the rear facades remained more or less intact. Gradually, the laws regarding building height were relaxed, from the original 16 metres (52 ft) to almost 30 metres (98 ft).
However, as can be seen in the diagram on the right, solar access was retained on all floors of the buildings on the other side of the street by placing the top floors somewhat further back -- in fact, producing a terraced building similar in shape to those of the Native Americans. In this way, developers looking for financial profit actually improved housing density without negatively impacting solar access.
The terraced upper buildings and the truncated corners in Barcelona, as well as the curved roofs in Paris and the slanted roofs in London, can all be considered embryonic steps towards Knowles' solar envelope. However, he improved the concept substantially in two ways. Firstly, he applies the idea of the sloping line to the whole building, not just the roof or the upper floors. Secondly, his slanting lines coincide precisely with the rays of the sun, which was not necessarily the case in these earlier examples. To add to this, the earlier building codes produced monotonous architectural forms and street views, while the buildings under the solar envelope can be diverse, depending on their specific location and surroundings.
The solar envelope and street orientation
The size and shape of a solar envelope is influenced by the orientation of the streets. In the US, city layout is usually based on orderly subdivisions that have geometricised the land. Typically, throughout the midwest and the west, streets run in line with the cardinal points so that rectangular blocks extend in the east-west and north-south direction of the Jeffersonian grid (or US Land Ordinance, enforced in 1785).
In the southern US, as well as in large parts of Latin America, a similar grid appears, with the difference that it is oriented diagonally at the cardinal points, with streets extending northeast-southwest and northwest-southeast. These grids were laid out according to the Law of the Indies, a manual for the construction and administration of colonial communities compiled by the King of Spain in 1573.
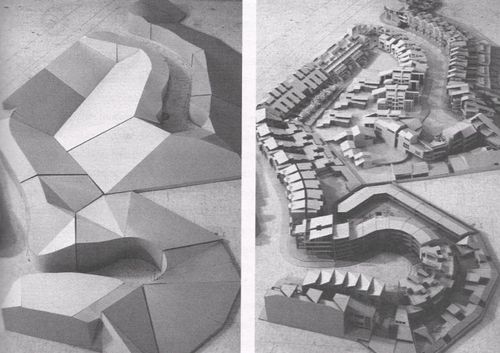
In Europe there are relatively few gridirion city lay-outs to be found. The existence of older city fabrics, resulting from centuries of unregulated growth during the middle ages and the Renaissance, constrained experiments with urban planning. Many European cities were 'modernised' at the turn of the twentieth century, by laying out wide streets and boulevards that cut through the older parts of the city - Paris being the most (in)famous example - but basically randomness still rules. The solar envelope can be applied to all possible street layouts, even if they're chaotic, with differing results.
William Atkinson: avoid east-west, north-south
In "The Orientation of Buildings, or Planning for Sunlight", William Atkinson devotes a chapter to the importance of street orientation for solar planning. He argues that the Jeffersonian grid should be avoided in order to provide optimal solar access for cities. Instead, he follows Cerdà and backs the Spanish grid:
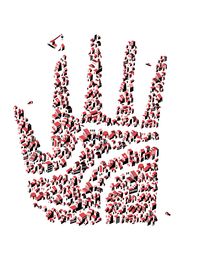
"When streets are laid out at right angles to each other according to the checkerboard plan, the best distribution of sunlight is obtained when one series of streets runs northeast-southwest and the other northwest-southeast. It is unfortunate that in so many cases where the 'checkerboard' plan has been adopted, the streets have been laid out north-south and east-west, which is the worst arrangement possible."
Atkinson agrees that "if we were to base our judgment wholly on the amount of sunlight received by windows, we should conclude that the best position for [a] building is with its long axis placed east and west". However, he saw an important disadvantage with this orientation: it involves an area of "complete shadow" on the north side of the building, during one half of the year (autumn to spring), while in the case of a building with its diagonal upon the meridian all sides receive sunlight throughout the year. The same goes for the streets:
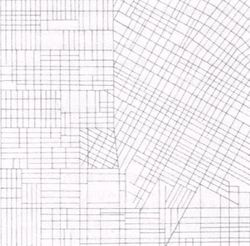
In an east-west street the surface of the street receives no sunlight at all during six months of the year, and the buildings on the south side of the street are in perpetual shadow. In contrast, when the streets are oriented diagonal upon the cardinal points, the buildings shade the surface of the ground much less".
Ralph Knowles: it depends
Knowles did most of his research in Los Angeles, which makes it easy to compare both grid patterns -- LA consists of both an older Spanish grid and a newer Jeffersonian grid (illustration on the right). Knowles acknowledges that the Jeffersonian grid is not an ideal solution:
"Streets that run east-west in a built-up area will tend to be shadowed during all of a winter day. The streets thus remain dark and cold. By contrast, streets that run north-south are lighted and warmed during the midday. In summer, streets that run north-south will be shadowed in the morning and the afternoon, but will receive the full force of the midday sun."
"From the viewpoint of solar orientation, the Jeffersonian grid leaves something to be desired. Its east west streets are too dark and cold in winter, its north south streets too bright and hot in summer. In Los Angeles, the older Spanish grid seems to have advantages regarding street qualities of light and heat. During the winter, every street receives direct light and heat from the sun somewhere between 9 am and 3 pm, the six hours of greatest radiation. Every street has the advantage of some shadow during most of the summer day."
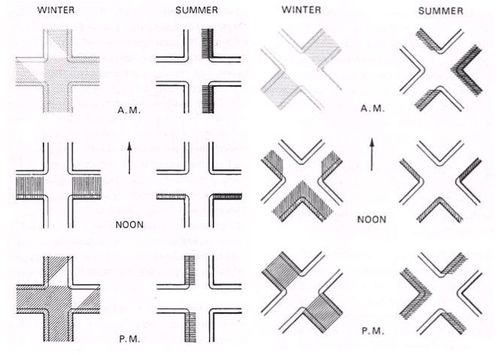
"Generally, the most height, and hence volume, are attainable at either of the two block orientations within the Jeffersonian grid, and the least volume is attainable at about the angular orientation of the Spanish grid. The street's gain in sunlight thus appears to be the developer's lose. Other design values can dictate grid orientation but, for volume alone, the Jeffersonian grid has the advantages."
Within the Jeffersonian grid, sites oriented in the east-west direction allow a greater volume of development under the solar envelope than sites running north-south. Knowles calculated that, given the same site shape and proportions of 1:3, a building site oriented long on an east-west axis will generate 40 percent more volume and 400 percent more south face over the north-south orientation.
In short, the grid layout best suited for both maximum solar access and maximum building density is one with rectangular blocks running long in the east-west direction - as was the case in the Ancient Greek solar communities and in Acoma Pueblo.
Combining the best of both grids
One problem remains, though. Within our cities, the orientation of houses is toward the street rather than toward the sun. If a street runs east-west, only the buildings on the north side of the street will have south exposure at their street front (providing that the buildings on the south side of the street do not shadow them). The buildings on the south side of the street have south exposure only at their rear facades. If a street runs north-south (and a grid system having desirable east-west streets will by definition have north-south streets, too), none of the buildings will have the ideal south exposure.
To solve this problem, Knowles reverts to the solution provided by the Ancient Greeks, using courtyards and alleys that are curved out from the middle of the site. Moreover, he shows that the concept can be further improved upon. This can be seen in the Bunker Hill Project, in which a solar envelope was calculated for a large vacant lot on the Spanish grid in downtown Los Angeles. One of the building designs made to fit this solar envelope, consists of an internal and separate street composed of a sequence of large and small squares, cascading diagonally across the site from its higher edge to its lower edge.
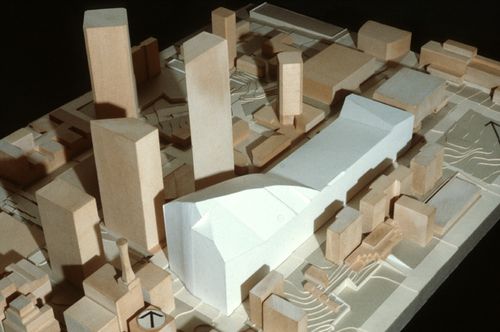
During the midday hours, the sun sees down the entire length of the interior street because it runs more nearly in a north-south direction, diagionally across the street. At 3 pm in winter, the public space is still in sunlight, as is the entire length of the interior street on its southeastern side. "Such sensitive tuning of the building's location and form", writes Knowles, "combine the best aspects of both the Spanish grid and the Jeffersonian grid."
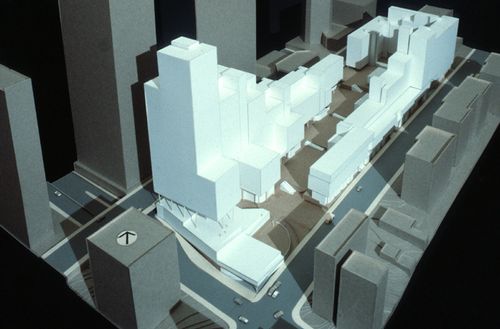
When aiming to combine the advantages of solar access and wind conditions, similar compromises can be found. Knowles:
"Sometimes complex sets of environmental forces act congruently so that, for example, desirable sun and wind may come from the same direction. In this fortunate circumstance, the building may be oriented to catch both sun and wind on its broad south face and the building may thus be open on that side. On the other hand, directional forces more usually act incongruently, making choices necessary. The sun and wind may come from different directions. Where choices exist, values must be set. For example, the sun may be recognized through a primary locational adaptation in which the whole building becomes oriented to the south. Adaptation to a west wind would then be handled with a secondary form mode in which the building's surface structure scoops in the wind for natural ventilation."

Over the years, Knowles and his students have performed multiple studies of the solar envelope's development potential in Los Angeles. These were done on sites with different land values, topographies, street orientations and neighbourhood characteristics in order to test the effectiveness of the envelope over a range of conditions.
Density is hard to define, and can be measured in different ways. One approach is to calculate the amount of dwelling units per unit of area (acre, hectare, square km or square mile), while another calculation determines the amount of people per unit of area. A third approach is to compute the average floor-to-area ratio (FAR) of the site. All these approaches of density have their shortcomings and are preferably calculated alongside each other in order to make sound comparisons. The size of apartments, the occupancy, the ratio between office space and residential building, and the amount of parking space are among the main factors that can distort density results if only one figure is shown.
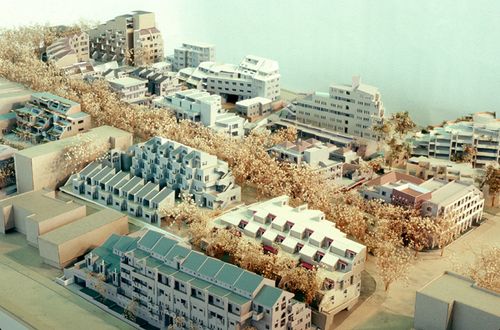
Knowles and his students have reached densities of up to 100 dwelling units per acre (100 du/ac or 247 du/ha) for residential development. If we count on an average of two people per apartment (Knowles does not give occupancy rates), this comes down to 200 people per acre. These figures were obtained on the Spanish grid, with apartments averaging 93 sq m (1000 sq ft), a minimum of 4 hours of sunshine in winter, and a 6.1 meter (20 ft) shadow fence. For mixed use development, Knowles obtains a FAR of up to 7.5.
Three to seven floors
Of course larger apartments, more demanding cut-off times and lower (or non-existing) shadow fences would reduce the development potential. On the other hand, the density would improve if the project were to be located on a Jeffersonian grid, which boosts development potential. For a site in Los Angeles measuring 150 x 205 ft with a guaranteed solar access of 6 hours, a solar envelope oriented diagonal at the cardinal points will have 1.5 times less developeable volume than a solar envelope oriented east-west, and 1.3 times less than a site oriented north-south.
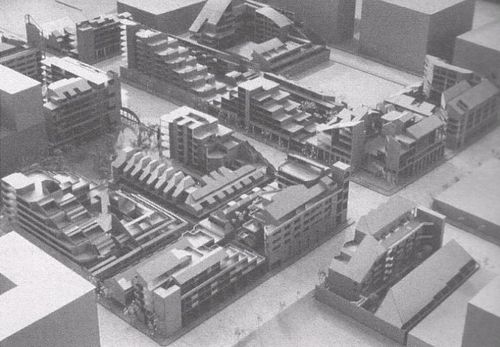
Overall, the maximum densities reached by Knowles and his students are about twenty times higher than those of the average American city (6 to 7 dwelling units per acre). This means that a city like Los Angeles (5 to 7 du/ac) could be shrunk by a factor of 15 to 20, while still assuring four hours of solar access per day to every household.
Based on the relationship between density and surface-to-volume ratio (an energy-related measure of building form), Knowles concludes that three to seven stories generally represent the best size range for urban housing in LA. These buildings - about the size of those in nineteenth-century Paris and the Barcelona Eixample - offer the greatest potential for architects to conserve energy while attaining reasonable densities.
In fact, the density figures obtained by Knowles correspond rather closely with those obtained by city blocks in Paris and the Barcelona Eixample - still the most densely populated, large cities in Europe (despite of the near absence of skyscrapers).
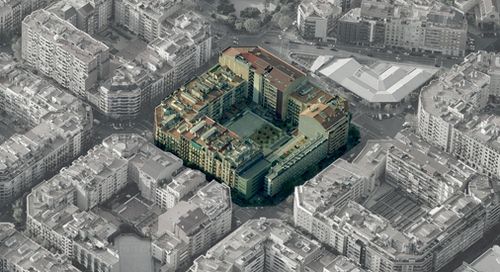
One analysis of a typical Eixample city block (how it turned out, not according to the original plans of Cerdà) found a FAR of 4.70, a dwelling unit density of 93 du/ac (230 du/ha) and a population density of 145 people per acre.

A close-up of a typical Parisian city block - with courtyards - resulted in a FAR of 5, a dwelling unit density of 120 du/ac (297 du/ha) and a population density of 602 people per acre (see the illustration above).
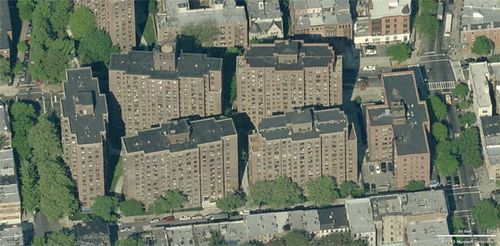
The densities under the solar envelope also compare favourably with some residential city blocks in New York City - the most densely populated city in North America - although not with others (picture above) and especially not with early twentieth century city blocks in NYC (picture below), which reached 331 dwelling units per acre. Few of those apartments received any sunshine, though.
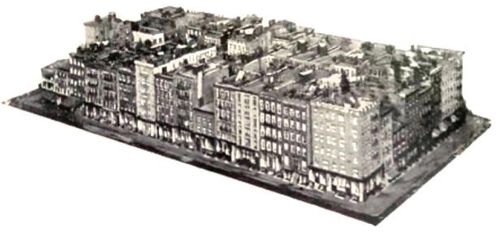
It should be noted that while Knowles achieves densities comparable to those of Paris and Barcelona city blocks, solar access is improved over these earlier examples, especially compared to Paris where street orientation (and thus building orientation) was not controlled.
Improving development potential
Even higher densities and taller buildings could be reached under the solar envelope if larger land parcels were to be assembled, in particular when city-blocks are oriented long in the east-west direction. This results from the geometric relationship between linear, plane and volume measures; a doubling of plan dimensions will square the land area and cube the envelope potential. An envelope over a 100-ft frontage site has more than twice the volume of two envelopes over adjacent 50 ft lots, because of the additional wedge of volume added when two adjacent envelopes are assembled.
However, it should be remembered that the buildings within a solar envelope will have less volume because of internal overshadowing issues, to be solved by the architect.
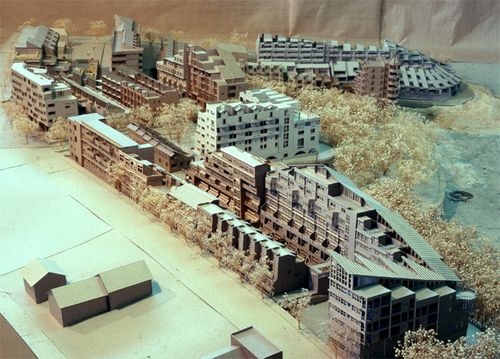
A few special conditions, such as a park or wide boulevard where longer shadows could be cast without harming a neighbouring property, also allow for taller buildings and higher densities. A building project located on a hillside close to downtown LA achieves a density of up to 128 du/ac (316 du/ha), because it is allowed to overshadow a park. In general, though, higher densities will have an adverse effect on solar access. If we want to heat and cool our cities using solar energy, density will generally be limited to about 100 dwellings per acre.
Last but not least; the above results apply to Los Angeles. They are valuable for any other city at the same latitude of 34 degrees north or south of the Equator, like Buenos Aires, Osaka, Sydney or Montevideo. At other latitudes, however, things will change, as latitude affects the height and therefore the volume of a solar envelope. If the cut-off times are held constant, the envelope height decreases as the latitude increases, and vice versa, primarily because of the critical effect of winter sun on the solar envelope's north slope. Consequently, the volume of a solar envelope increases with proximity to the equator; the volume decreases towards the north and south poles. For example, in Paris (48 degrees latitude), Barcelona (41 degrees) and New York (40 degrees) solar envelopes would be smaller than in Los Angeles (34 degrees).
Closer to the equator the position of the sun will not vary much throughout the year, which makes the traditional approach to passive solar design worthless. Instead, the prime concern in the building design would be the ability to keep out the sunlight and heat to reduce the energy consumption of artificial cooling. This might involve orienting the building to the north.
For higher latitudes, Knowles suggests a greater design emphasis on the east and west exposures (including sunscreens for sun control in summer) to improve solar access. Apart from latitude, slopes also have profound effects. A south slope in a more northern country can allow for densities that are otherwise only possible in southern countries, while a north slope does the opposite.
According to Knowles, investigations of the solar envelope have been done in places as far north as Bratislava at 48 degrees and as far south as Honolulu at 21 degrees, leading him to the conclusion, perhaps a bit overoptimistically, that "the benefits of solar zoning can be achieved around the world".
Compromise
Density is a pet subject of environmentalists, who argue that densely populated cities are the solution to lower the energy requirements for transportation. On the other hand, the solar envelope shows that above a certain treshold, density can also raise energy requirements, in particular those of heating, cooling and daylighting buildings.
This means that it would probably be wise to aim for a compromise. If we would take the highest densities reached under the solar envelope as an upper limit, we could create cities where the critical functions of buildings can be met without fossil fuels, while still retaining (more than) high enough densities to make public transportation, bicycling and walking attractive.
Kris De Decker (edited by Deva Lee)
Sources and more information:
- "Ritual House: Drawing on Nature's Rhythms for Architecture and Urban Design
", Ralph L. Knowles, 2006.
- "Sun Rhythm Form
", Ralph L. Knowles, 1981
- "Energy and Form: An Ecological Approach to Urban Growth
", Ralph L. Knowles, 1974
- "A Golden Thread: 2500 Years of Solar Architecture and Technology
", Ken Butti and John Perlin, 1981, reprinted in 2009
- "The orientation of buildings, or planning for sunlight", William Atkinson, 1912
- "Teoría general de la urbanización y aplicación de sus principios y doctrinas a la reforma y ensanche de Barcelona", Ildefons Cerdà i Sunyer, 1867
- "Ildefonso Cerdá", "Distrito del Ensanche" & "Plan Cerdá", Wikipedia Spanish
- "Walks Through Lost Paris: A Journey Into the Heart of Historic Paris
", Leonard Pitt, 2006
- "Responsive and sustainable architectural strategies for temperate regions", S.M. Mofidi, 2005 (PDF)
- "The City as a Work of Art: London, Paris, Vienna
", Donald J. Olsen, 1986
- "Spanish city planning in North America", Dora Crouch, Daniel Garr, Axel Mundigo, 1982
- "The Density Atlas", MIT (website)
- "Visualizing density" (PDF)
Its amazing how many still relay on heating oil for their homes when a heat pump is a much more energy saving and future proof system for heating.
Many factors involved with heat pumps. Things like income, upfront costs, where you live, etc. So it doesn't amaze me at all. In my area, a heat pump would run 20K to install. I heat with wood for $500 a yr.
Air source heat pumps(split reverse cycle) can be <$3,000 including instillation.
I payed 9,500 NOK (US$1,800) for a heat pump with installation. It saves me huge money on the electricity bill and I dont have to pollute by burning wood. Also works as an air conditioner for cooling during the hot summer months, though thats a luxury helping to increase the el. bill.
I'm not sure how heat pumps have anything to do with passive heating and cooling. While a well designed passive structure or group of structures may or may not need an energy sucking backup system, I don't see that as the point of the article. In fact, this first thread is indicative of how folks just don't grok passive; jumped right into the complex, grid-connected, mechanized mode it seems. Shame on you :-/ It's like bringing an electric guitar to a string quartet performance.
Guilty as charged ! Some of us live in areas that are not exactly rich in passive solar potential; our city, for example, averages just 3.1 hours of sunshine per day between November 1st and March 31st (source: http://www.livingin-canada.com/sunshine-hours-canada.html). And when winter temperatures in the Maritimes routinely dip below -20°C and winds are strong enough to strip paint off shingles (http://www.youtube.com/watch?v=QBxtu4Qk4Ks), you best have a robust and reliable heating system and, preferably, one or more back-ups to boot.
As previously mentioned, our home is not passive solar friendly, but it's located in an older, well established area where I can walk to the library within five minutes and to my bank, grocery stores and range of other services in under ten. As true with most things in life, there are trade-offs and this is one of them.
We're quickly approaching the finish line with respect to winter, and our space heating costs at a blended cost of 13.4-cents per kWh will end the year at just over $500.00.
And if we were so inclined, we could cut that in half by closing off the basement.
Long and short: if you can maximize your passive solar potential you'd be crazy not to do so, but for those of us not so blessed you look for other options, including high efficiency heat pumps. Does this tie me to the grid? Sure, but so be it. If the grid goes dark, my personal situation will be no different from that of the next guy.
Cheers,
Paul
Wow! What a great article. Thanks, Kris, and Rembrandt for posting it.
As one who lives in a passively heated and cooled home, it baffles and disgusts me that we, especially in the US, have squandered our resource endowment on buildings and cities that attempt to defy natural cycles and orientations in deference to lousy asthetics and convenience, ignoring the lessons of the past. It makes me cringe when folks show me their marginally livable showcase homes; virtually uninhabitable without high energy inputs from complex and usually distant external systems (sun and wind notwithstanding).
This ancient planning concept for urban environments is both uplifting and at the same time, depressing. I fear modern societies have squandered any opportunity to adopt such common sense design considerations on any scale that matters much. Modern western societies are far too invested in poorly planned, shortsighted development, and far too many developing societies are following suit.
Looking to the future another issue will be temperature extremes. As far as heat is concerned I note Baghdad Iraq sometimes hits 50C and I presume few residents have air conditioning. You can't think straight when it's that hot therefore it's a public health and safety issue. It can't be long before a city in the West gets that hot; outer suburbs of Melbourne Australia for example have hit 48C. Cities which rarely dip below -5C might get cold snaps of -15C with the new weird weather patterns. The trend in horticulture (fruit&veg) is to grow in controlled microclimates. We need to not only reduce the external energy demand for average thermal comfort but also for extremes. Then factor in walkable neighbourhoods, urban farming and so on.
An interesting subject and a lengthy article touch on the topic of how do we create a habitable environment that signficantly reduces our addiction to non-renewable energy?
The point is to capture as much sunlight as possible within the conditioned space or via surfaces that will otherwise harness the energy.
There are serious deficiencies with orienting streets in a northwest/southeast direction (diamond shaped versus checkerboard when viewed from the north or south).
First, units facing southwest won't begin to receive warming rays until after noon, and the units facing southeast will no longer receive sunlight for another 18 hours.
Second, northeast and northwest facing units won't receive any rays (unless everything is at one level, which does not support anywhere near 72 dwelling units per acre.
Third, any units over 3 stories will tend to shade ground level units to the North.
Fourth, heat loss is a major factor in units with a significant outside wall area to floor area ratio. The shape with the greatest volume to external area is a sphere, with a cube coming next. Shapes that drift farther and farther from the latter will be less and less efficient at retaining passive solar heat.
Fifth, southwest and southeast facing units will require extensive shading overhangs in the summer in most of the lower 48 states in the US to avoid overheating.
Some contemporary efficient designs include those of the Bedzed and related communities;
Very interesting article, and quite surprising that no more work is done on that, or that "false received ideas" such as "skyscrapers allow to increase density"(false in particular for housing buildings) are still so prevalent.
The work of Lionel March and Leslie Martin in the sixties (in Cambridge) could also be mentionned, two papers about it linked below :
http://iiscn.wordpress.com/2011/05/15/densite-etages-lumiere/
I thought this was excellent. In particular, I like the way it demonstrates the use of neglected knowledge and ignores the artificiality imposed by Industrialism.
Our own roots, after all, are organic. A methodology that begins by embracing this fact must necessarily yield an optimized solution.
We need a lot more of this approach, if we expect to survive beyond the next few centuries.
A few thoughts:
The housing stock is largely static, dramatic changes to it have huge resource costs.
The density limits discussed of 100 units per acre imply dramatically higher population density than found almost anywhere. This could put the entire U.S. population into a square ~40 miles on a side. It is clearly demonstrated that passive solar is not limited directly by space constraints.
Solar heat gain is one factor in building energy consumption. External surface to volume ratio also plays a role. Dwelling units sharing common walls have reduced heating/cooling demand, all else equal.
Initial resource and design cost would be expected to be higher for passive solar design, how long would the energy payback be for typical locales compared to BAU or compared to a best-cost-case model of energy efficiency and renewable supply which ignores the solar envelope?
Thanks Rembrandt for posting this article. Kris, you did a great job of explaining how to integrate solar design into the cityscape.
I have worked with this subject for a long time and recommend Gail Boyer Hayes' book, Solar Access Law (Ballinger Press, 1979). It has been very challenging to lobby for or create new building code standards for solar access (so our neighbors won't build more stories or plant tall trees that shade our house). When the creators of the LEED certification program considered it, lamentably they backed away from making building orientation an explicit requirement, much less creating a metric for integrating a new building into its surroundings. They were afraid that practicing professionals wouldn't be able to rise to the occasion.
One of the difficulties is effective design communication between designers and building code officials. "Spherical geometry" is not exactly the easiest for either side to articulate. To aid in 3D design at the neighborhood scale, we have developed Encitra (Energy City Transportation), a 3D cityscape modeling tool that, amongst other things, automatically calculates sun angles and shading.
Kris asked about design near the equator. The same principles apply as at higher latitudes -- minimize the east and west sides.
I've written a somewhat whimsical article on these subjects, "The 4 Donkey Method of Bioclimatic design."
Special glasses and fancy shades that could be ripped to shreds in a storm. The local solution is to build shady overhangs and verandahs then add plants.
NAOM
Excellent article. It should be required reading for local government planning staff.
Here in Australia, we have been having a solid move away from good passive design. In an attempt to get the largest floor space possible on a given block, residential developers are building McMansions which are uninhabitable in summer without air con. FAIL - big time.
For high-latitude cities where heating is more important than cooling, I think the simplest and most effective technique for passive heating is to more or less ignore the sun and build gigantic block buildings. Yes I realize I'm being iconoclastic here. The goal would be to maximize volume while minimizing surface area, so that the heat generated by the building's occupants keeps it warm no matter what the sun is doing.
I did a simple back-of-envelope calculation which suggests that a well-insulated cubical apartment block about 50 meters on a side ought to generate enough internal heat (from the electricity usage and metabolism of the people inside) that it wouldn't need to be heated. Any heat provided by the sun is a bonus. A simple heat exchanger can get rid of any excess. During the summer, the building's sheer mass will even out afternoon heat spikes, reducing the demand for air conditioning.
Such a building wouldn't need to be dreary. The deep interior spaces could be used for shared facilities (rec rooms, commercial space, laundry, libraries), and illuminated using light pipes from the roof.
Wouldn't need to be cubical either: any bulky shape will do. The important thing is to minimize surface area to volume ratio: this is exactly the opposite design goal to passive solar, which tries to spread windows toward the sun to collect energy, but can lose much of what it gains back out through the same windows. And the Big Ol' Building design works fine when it's cloudy.
Back in the 'good ol' days' people knew how to build for the locale.
- Warmer climates the houses had high-ceilings and porches along with flow-through ventilation. Windows had shutters so the windows could remain open (without critters entering).
- Cooler climes had more row-houses, shared walls, tighter windows and doors. Older cities also had real work and goods/services that could be traded for the same originating elsewhere.
- Insulation (since 1900) has made buildings more energy-efficient.
- Old days had fewer people and a balance between supply and demand. Cities were trading and production centers rather than being credit dependencies/giant open-air lunatic asylums. Today's cities will never be anything other than massive energy sinks: the costs of building are high and increasing along with the service requirements of sunk capital.
What is emerging is the change away from applying credit to the service of 'luxury' (and warehousing of undesirables). Any city will have to be more than a development or collection of houses: it will have to pay for itself.
- Newer developments are universally automobile dependent ... never pay for themselves right there.
Interesting post. The concept of solar envelope should not be used, however, to increase densities. This is because we need space for veggie gardens. As oil production declines, there will be food shortages in the cities and every m2 available for planting your own stuff to supplement food supplies will be welcome.
This is what I learned in Tanzania, in the 70s:
Sustainable Cities Master Plan
http://crudeoilpeak.info/sustainable-cities-master-plan
An interesting article that will require several rereads.
New Orleans has an "eclectic mixture of grids" and neutral grounds (medians) are a major urban feature (and much loved). Solar gain is seen more as a negative for housing, and a positive for trees and bushes.
How does one design a city for maximum foliage ? And minimum solar gain for buildings ?
And I prefer the 1 to 3 story development we have - it is much more human scale - to much of what was shown. I like walking past flowering trees & shrubs and talking with people. Narrow, often one way streets (two way if walking) - low % of area for cars > high % for people.
Best Hopes for Better Urban forms,
Alan
PS: New Orleans leads the nation in fewest miles driven by residents - 13.6 miles vs. 16.0 for New York City. So it is a sustainable urban form.
First, as always Kris loved your post--there is a lot in it.
Been quite a while since I rode the City of New Orleans down to your fine city Alan, but it had as livable feel as any I've ever visited. Loved it. Of course I was there in February--don't know what I would of thought of August ?-) Still I could envision a varied landscape of somewhat taller denser city sections interspersed throughout less compact areas as very workable and livable--the solar envelope plan has a great deal of appeal. I ended up way out at the edge of the wilderness but there was a time I thought building more livable cities was what I was going to do.
New Orleans (and Netherlands for that matter) does suffer dramatically if the ice starts sliding off Greenland like snow coming off a flattish tin roof in the spring (a northern thing).
Best hopes for keeping the ice hanging around. No doubt much greater use of passive solar near everywhere and fast would up the chances for that--at near 65° north I have to pursue other solutions myself, though even this far up solar thermal can work on the right site.
This is all very promising. Far higher densities can be supported with near full passive heating than I would have guessed before. Of course there is a considerably non-applicability in historic city centers, where no change in appearance whatsoever is allowed. But all newer buildings that are allowed to be rebuilt/demolished would apply, which is most of the market.
Getting rid of the big space heating/cooling demands is very beneficial to our energy situation; not only does it save a huge chunk of primary energy demand, it also gets rid of a big seasonal variation in energy demand (which requires fossil fuels to deal with). Without that seasonal variation, the remaining energy demand becomes much more stable over the year. Ideal for nuclear powered grids and transport. A great example of nuclear-solar synergy. But not as sexy as solar panels, of course...