Tech Talk: Ground Freezing to Stop Water Flow and Strengthen Soil
Posted by Heading Out on October 3, 2010 - 8:42am
Last time I wrote about grouting of ground in order to either strengthen it, or to stop water flowing through it. Grouting is something that is often carried out during the driving of the tunnel or shaft, so that the effects can be immediately assessed. If the water continues to flow or the cement has failed to do what was desired, then a second set of holes can be drilled, and secondary and subsequent injections can be made until the goal is achieved. It is more common than not to have to make several injections. The alternative, is to immobilize the water, or strengthen the ground before the excavation is started. While this can be attempted with grout, it is more common to use a different idea.
This is ground freezing, and it differs from grouting in many ways. One major difference is that the holes for the process are drilled before the excavation is made, and a second is that the changes to the surrounding soil and rock are temporary since, when all is finished, the ground is thawed and reverts to its original condition (or close). It has the advantage that if the soil/rock is relatively impermeable or has pockets of water not easily accessed by grout injection, if the overall temperature is lowered below freezing, then the water will freeze, even if not directly accessed. This means that the zone around the pipes can be froze, even if the pipes go down through layers with different water content.
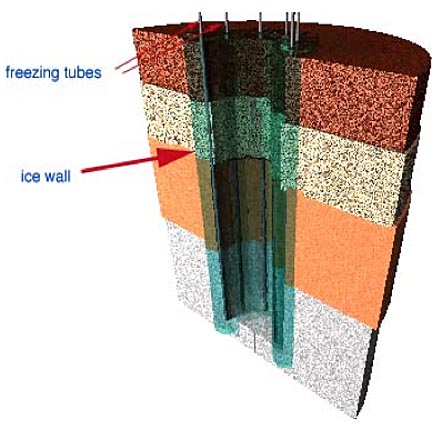
So how does it work? There are a number of different ways of going about the process, but I am only going to briefly describe a couple of them. The first is the more conventional approach, using a brine coolant, and the second is more commonly used when, for example, you’re refurbishing a road tunnel, and the roof suddenly collapses all the way to the surface. (The injection of liquid nitrogen).
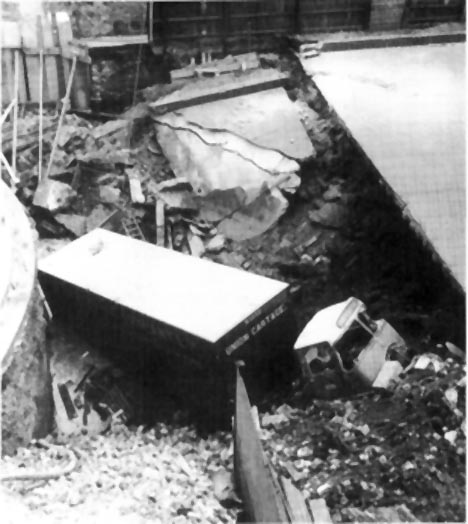
By completely freezing the gravel and other constituents of the roof and tunnel line, it was possible to restabilize and excavate through the area, putting in new supports at the same time.
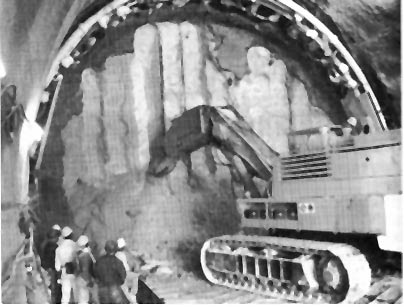
Ground freezing can effectively form a temporary roof over an excavation, even if very close to the surface. It was used, for example, during the Big Dig, to create a bridge under the railway lines in Boston, while the new tunnel lining was pushed into place beneath it, using hydraulic jacks. It was also used in Vienna, where a subway had to pass relatively closely under existing buildings.
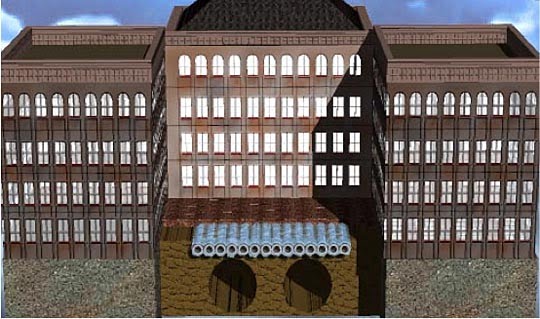
Ice wall as temporary support (image Joe Summers)
The conventional method of freezing involves inserting two sets of concentric pipes into the ground, inside pre-drilled holes. The outer pipe is sealed at the bottom end, so that as the freezing fluid (typically a chilled brine) is pumped down the inner pipe, and then flows back up the outside, it draws heat from the surrounding rock and soil, lowering the temperature until the water freezes. After circulation the brine returns to the refrigeration plant where it is re-cooled and re-circulated.
It is important to know the chemistry of the water in the ground, since with the wrong combination the water may not freeze at the expected temperature. It is also important that the outer pipe be sealed since if there is any leakage, then the brine may spill into the surrounding rock. At that point it can’t be frozen with the system, any longer, and an alternative method has to be used.
It is generally smart also, particularly when digging near the surface, to make sure that there aren’t any pipes (such as water and sewer) that can act as heat sources during the process. There can be embarrassing results if one of these (particularly the latter) is undetected, and the surrounding ground collapses as the shaft is then dug.
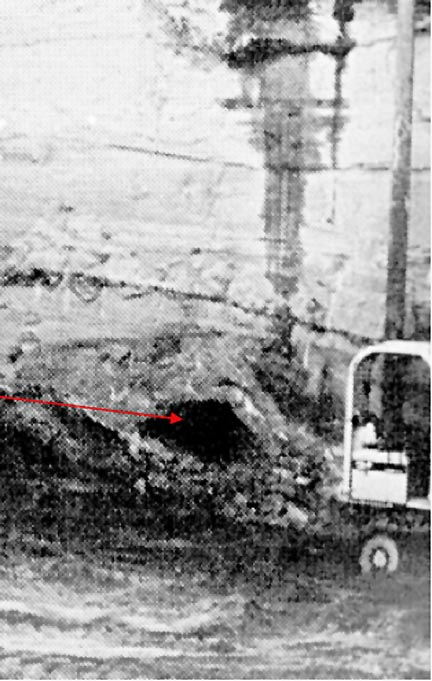
Brine operations generally take a considerable time, and there is a project schedule so that you can get an idea (depending on the depth and size of the hole) of what might be involved in such an operation. Remember that the ice wall has to be kept cold during the excavation, but that keeping the central material unfrozen makes it easier to dig out. Cement poured against a frozen wall, if properly designed, will set as planned, since the heat of hydration overcomes the surrounding heat loss.
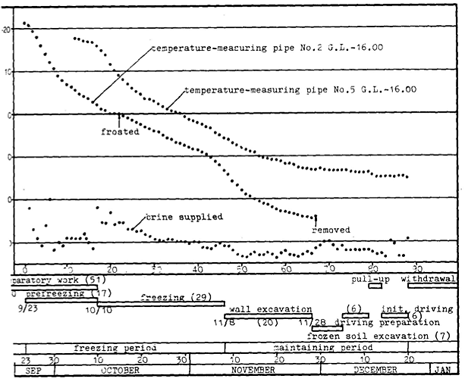
This requires, obviously, a lot of preplanning. Where there is an emergency this is not possible, and thus the use of faster freezing methods, such as the injection of liquid carbon dioxide, or nitrogen, into the ground, in order to freeze and stabilize it more rapidly.
There are two ways of making the injection. There is the dual-pipe approach where the nitrogen is in a closed circuit, and then there is the simpler process where a lance is, simplistically, pushed into the ground and the resulting gas percolates upward from the end. That is a little less precise, given that the gas moves through the ground following the path of least resistance, but it does have the advantage of being quick, and generally effective in stopping an imminent disaster. Relative to the months of a brine installation, the work takes a week or so (depending on size).
Copper freeze pipes with a standard diameter of 2” (54 mm) are installed, at an average distance of 2” (54 mm) On the inside, downpipes with diameters of ½” (10–12) mm are installed.
LIN is fed into the pipes through insulated supply lines. The LIN vaporizes, with 1 kg of LIN extracting about 200 kJ of energy from the surrounding soil, cooling and freezing it. The vaporized cold nitrogen (i.e., exhaust gas) extracts another 100 kJ from the ground. After about one week, this process forms a frozen wall with a diameter of about 1 m. This so-called “establishing phase” lasts four to seven days, and about 300 - 500 gal (1,500–2,500 l) of LIN is used to freeze 1 cubic yard (0.75 m³) of soil.
Thanks HeadingOut. Informative as always.
Between the two methods, which is usually more economical? Which is more energy intensive? On one hand, it takes a lot of energy to refrigerate all of that brine constantly but, on the other hand, it takes considerable energy to make the concrete used in grout. I guess if one knew just how much concrete was used the rest would be relatively easy to figure out. I'm going to guess that ground freezing is ~3x more energy intense.
I've not done the calculation for the energy cost of grouting, which if only done in a small area won't be that intensive, whereas freezing is more generally over a larger area, and the energy costs are part of the calculation, since you need to know the heat quantities that have to be removed, and the rate at which you can remove it. It really depends on a number of factors. For example freezing can be used to strengthen weak rock, and then you have to decide, based on factors other than just blocking the water flow, how thick to make the ice wall.
How good is the integrity of an ice wall such as shown in your first figure? Shell's in situ proposals for extracting liquid fuel from oil shale involves creating such a wall around the volume where they will heat the shale to prevent liquefied kerogene from migrating into the ground water. As I understand the technology, Shell will have to maintain the wall for a period of two to three years.
After a couple of spectacular failures it is now standard practice to check the integrity of the wall before making the excavation. Usually this is done acoustically, since the signal changes significantly when the water turns to ice. A transmitter in a central bore, and receiving instruments in boreholes around the site make it a relatively straightforward evaluation.
Once the wall is in place, provided that the surrounding water flow in the rock/soil is not great, maintenance costs are relatively low. There needs to be a certain amount of water in the rock for this to work, particularly for strengthening the rock, but when the intent is just to stop the water flowing, it becomes a function of how fast that flow is, as to whether it is economic.
I'll take a crack at it.
I believe 1 acre(208' x 208') of Piceance oil shale about 100 feet thick contains 100,000 barrels of shale oil.
Since the purpose is just to keep the groundwater from migrating, I assume the freeze wall perimeter would be
at least 100 feet from the heated area(so the heat doesn't boil the LN2.)100 feet of rock should be a fairly
good insulator.
A 2" borehole freezes a 1 meter cylinder of rock so 140 boreholes would cover 400' and a total of 560 boreholes would be needed.
If a 2" borehole 100' deep could be filled w/16.3 gallons of liquid N2(LN2) which weighs 3.5 kg per gal, then 560 boreholes would hold 32 tons of liquid nitrogen.
It probably takes around 300 KJ/kg to make LN2 so it would take 9.6 GJ. I would guess it would take a month to
thaw(32 degrees)the ice dam so 36 months would need 1152 tons of LN2 or 345.6 GJ of electricity or 1 TJ of primary energy. A barrel of oil is 5.8 GJ of energy so 1 TJ is 178 boe, not much in a 100000 barrel project (580 TJ).
OTOH, to heat up an acre of oil shale (600,000 tons) to 700 degreesF(182 TJ) and keep it there for 3 years (at 1.2 GJ/hr) would take 32 TJ.
Electrical heat up =182 TJ
Heat loss over 3 years = 32 TJ
Nitrogen over 3 years = 1 TJ
Total = 215 TJ
Primary at 38% central station = 565 TJ
Oil out = 580 TJ
A 365 acre(0.6 sq. mi.) per year operation (.1 mbpd) would take 78,500 TJ or 21.9 Twh.
Barely net energy positive with thermoelectric generation, which is why I proposed ( a long time ago)
massive local(South Pass has that capacity) windpower 21.9 Twh(which requires no water) or 8700 1 Mw wind turbines running 2500 hours per year.
get better return on increasing the volume ice walled no? cube powers vs square powers and all that
make sense to get it as big as possible?
36 months 30m of rock will do as a insulator?
if you are going to do it you need to go pretty heroic
9000 1mw turbines per 30000 barrels of oil a year
1 millon barrels a year production is 290 000 wind turbines of 1 mw class
whoa
thats > 1/4 of projected globally installed wind turbine capacity in 2010!
http://www.wwindea.org/home/index.php
well why not I suppose?
Probably true re: economies of scale with the ice wall. Though I'd imagine that, at a certain point, you reach dis-economies of scale considering that the point of the ice wall is to contain the kerogen.
Um..
if .1 mbpd = 8700 1 MW wind turbines
then
1 mbpd = 87000 1 MW wind turbines.
This is just for fun ;) but the amount of energy required is
humongous.
A really clever person could think of a way to use geothermal heat to directly heat up the shale.
takes 36 months
ie 3 years
30000/yr production capacity
or 33333.3333333333333 if you haven't got a grip on sig numbers etc was my thinking
sorry misread your post thought the 8700 fig was for the 100000 barrels
I just bookmarked this page because of your calculation :)
thanks for the enjoyable post.
I'd like to see kWh/bbl.
For one acre, you're saying, 215TJ of elec = 100,000bb of oil.
Or, 59,722,222kWh = 100,000bbl
Or, 597kWh/bbl?
For 365acres, 21.9TWh = 36500000bbl
Or 21,900,000,000kWh = 36500000bbl
Again, about 600kWh/bbl. At $0.15/kWh, about $90 per barrel just for heating and cooling the ground. Do you really advocate using the electricity, even if from turbines, for that?
Your energy input figures look about right. Your estimate for oil content of the shale may be just a little low: USGS estimates 1.5 trillion barrels; basin size is 7000 square miles, for a total of 250,000 barrels/acre, over twice your estimate. (Overly optimistic press releases or limits on extractability would reduce this.)
Also, is Shell planning on using electrical heating? Seems like coals to Newcastle: why not use fossil fuels directly? That loses you the 38% electrical conversion factor in your calculation.
Also, by only heating small patches of the shale field at a time, taking advantage of natural thermal diffusion from a patch into its neighbors, and making clever use of heat exchangers to move heat from old extraction patches to new ones, one can probably reduce the heating needs significantly.
With all three of these factors together, I think you could probably get the EROEI of this from one-to-one up to five- or ten-to-one.
Misc notes from a Shell presentation at the Colorado School of Mines...
If the ice wall is 1700 feet deep instead of 100' then the energy for nitrogen would have to 17 TJ instead of 1 TJ, which is only slightly increase electricity 7.5% from 215 TJ to 231 TJ for 100,000 barrels.
I get 1 mbpd for 843150 TJ(3650 x 231TJ) or 233.5 Twh of electricity.
1 mbpd = 2117000 TJ per year so
2117000/843150 = 2.5
This fairly IMO agrees with EROI of 3.
Google says Colorado used 51.3 Twh of electricity(2007)(produced 53.9 Twh).
http://www.swenergy.org/publications/factsheets/CO-Factsheet.pdf
I'd have to see the presentation to see how they figure
the EROI of 3 with on 51.3 Twh of electricity (184,680 TJ)
for 1 mpd 2117000 TJ. EROI of 11.4?!
Wikipedia says they'd need 12 GWe coal electricity plant for 1 mbpd (or ~50 million tons of coal?) which is 100Twh or 361011 TJ.
This is an EROI of 5.86; 2117000/361011, which still looks too high.
http://en.wikipedia.org/wiki/Shell_in_situ_conversion_process
My back of the envelope estimate of 843150 TJ or
231 Twh/yr for 1mbpd 2117000 TJ/yr looks the closest to me at 2117000/843510 = 2.5 EROI ~3.
shell IIRC the heating elements are spaced 10-15m apart for a 3 year treatment hard to imagine rock 7m away at 700° is still below 3° (whatever brines freezing point is) at 30m? maybe thats just uniformed intuition on my part?
OTOH your calculations point to the wall being a small proportion of the total energy and q 400m thick wall may allow for a bit of melting?.
5mbpd is 400,000+ 1mw windmills or twice the total global wind capacity today...ok pretty impressive but on reflection not unimaginable... I could imagine the USA running on 5mbpd of liquid fuels(+ a bit from elsewhere) with a fair amount of rejigging in the rest of the economy to electrify all the other stuff/ recycling plastics etc. Ok not possible tomorrow or with in a decade but 30-100 yrs down the road? yeah...why not
But that 1.5 trillion barrels is gone in a few centuries. its still not forever. As soon as we go down this road we have to start planning for what comes next after the shale.
where would the windmills go?
ExxonMobil’s Electrofrac Process
http://www.searchanddiscovery.net/documents/2008/08131symington/ndx_symi...
slightly different take
"making clever use of heat exchangers"
What is the heat exchage material?
What is the heat exchage material?
I dunno, supercritical steam?
Drill down, place a closed concentric pipe system like Heading Out describes above in the drill hole. Fire up a boiler at the surface and pump steam down the pipes to heat the rock. When you're done "cooking" one section of the shale field, pump water down the pipes. The hot rock will transfer heat to the water: run that water through your boiler to "top up" its temperature, and pump it back down into the next section to be cooked.
You'll need some plumbing that can stand somewhat more pressure than boiler engineers are used to and somewhat hotter temperatures than petroleum engineers are used to, but hopefully still off-the-shelf.
@goodmanj,
AMSO is doing something very similar to what you describe.
Thanks for this report. We have an invasive species, called phragmites here in New England, and I'd like to figure out how to kill it. My thought is the roots will die if frozen. I think it's worth looking into. So thanks.
I also used to do a lot of hand digging on a surgical digging crew. We used small conveyor belts. We would go under old houses on Nantucket and put in new, or repair old foundations. We could capture a chimney base with a new pad. Moving buildings, moving roads, or railroad tracks, may in the future be possible with this technology. Who'da thunk it?
I live in the Boston area, and took the commuter rail in to South Station while they were jacking the new Big Dig highway tunnel under the tracks. Quite a sight to see the tangle of brine pipes running between the railroad tracks, all covered with ice and shedding cold fog in the middle of summer!
Heading Out talks about using freezing tubes to form "ice walls", and then excavating the area between the walls. But if I remember correctly, in Boston, a less subtle approach was used: supposedly they froze the entire volume of ground that was to be excavated, and then excavated the tunnel using standard hard-rock excavation gear. Didn't even bother to remove the brine pipes: just cut right through them.
Is it possible to form a large container or working volume for shale oil extraction by using insulating high temperature cement in preference to long term ground freezing?
The formation of this zone starts with circumscribing the zone of interest using underground drilling and fragmentation (similar to shale gas fracking) around the periphery of the zone as a preamble to an insulation process.
Once the fragmentation zone is prepared, the insulation process begins. This process entails the injection of high temperature cement fortified with silica aerogel pellets or other high temperature insulating minerals into the fracture zone.
The insulating cement aggregate is pumped underground at high pressure and completely fills the spaces in an around the area of fragmentation volume on the periphery of the shale oil process zone.
This border of insulation is wide enough to provide a solid and rigid retaining structure around the shale oil process zone. This border insulates this heat retention zone to retard the flow of heat out of the heated zone even if some fractured material melts within the structure of the retaining volume.
A top and bottom of the heat storage zone can be formed in like manor. Also ground freezing can also be applied if required to exclude water from the fragmentation zone. Cement poured against a frozen wall, if properly designed, will set as planned, since the heat of hydration overcomes the surrounding heat loss.
What are the problems with is approach?
The freeze wall is to protect the aquifer, not to retain heat, interestingly the shale is a pretty good insulator, which is why the Shell ICP process takes so long (for the heat to migrate through the formation).