Weyburn, CO2 Injection and Carbon Sequestration
Posted by Dave Cohen on December 14, 2005 - 11:46pm
Tip of the hat to
Engineer Poet for introducing me to this interesting and important story.
Recently, a little publicized meeting called the EOR Carbon Management Workshop, was held at the Petroleum Museum in Midland, Texas.
Recently, a little publicized meeting called the EOR Carbon Management Workshop, was held at the Petroleum Museum in Midland, Texas.
Michael Moore, director of the workshop, noted that the developing technology of capturing carbon dioxide (CO2) emissions, sequestering them geologically or utilizing those emissions in enhanced oil recovery has received government approval.In this post, we'll examine the claims about Weyburn's success to cover two important related subjects.
Holding up a recent announcement from U.S. Secretary of Energy Samuel Bodman about the successful sequestration of 5 million tons of CO2 into the Weyburn field in Saskatchewan, Canada, he declared, "the government says it works!"
The Weyburn Project, funded by the U.S. Department of Energy, captured CO2 from a coal gasification project in North Dakota and transported it over 200 miles to the Weyburn field for sequestration and enhanced oil recovery. The DOE estimated the field's oil recovery rate was doubled and, if the methodology was applied worldwide, would eliminate a third to a half of CO2 emissions over the next 100 years while helping recover billions more barrels of crude oil.
- CO2 injection for EOR (enhanced oil recovery)
- CO2 sequestration as a solution to climate change
To emphasize the importance of all this, here's what Qatar's representative at the Petroleum Museum workshop had to say.
CO2 Injection for EOR
As reported by the DOE, CO2 Injection into oil fields is a tertiary recovery technique first tried in Scurry County, Texas in 1972.
Examining the Weyburn EOR graphic, water injection was used first, later some new vertical wells were drilled (yellow area), followed by horizontal infill drilling (the red area) in the 90's to offset declines after production had considerably fallen off. Finally, CO2 injection was tried beginning in the year 2000. As in all tertiary recovery, this technique was used to amplify and extend the tail of an oil field's production to extract "stranded" oil in place. As TOD contributor westexas can no doubt tell you, use of tertiary recovery generally has not fundamentally altered the shape of the Hubbert Linearization for lower 48 US oil production but has marginally increased that production by extending the tail of the linearization curve into the future. As the DOE reports (link above), "Today, less than 700,000 barrels per day of oil is produced in the United States by EOR processes (compared to about 6 million barrels per day of total domestic production). Thermal techniques [another tertiary EOR strategy] account for over 50 percent of U.S. EOR production, primarily being applied in California; gas injection [CO2 in this case] accounts for nearly 50 percent." For Weyburn, CO2 was determined to be the "solvent of choice" and is only used in those parts of the oil field where this is the case. See Role of Enhanced Oil Recovery in Carbon Sequestration--The Weyburn Monitoring Project, a case study (pdf).
CO2 Capture and Storage (CCS) aka Carbon Sequestration
Weyburn is an ideal experiment. Not only do such carbon capture processes not exist at all current and planned industrial production sources (again, for example, new coal-fired power plants in the US or China) but also, even if they did, there are no programs for transporting the CO2 to places where geological sequestration could take place if the infrastruture were in place to do so. These are the problems the world faces in implementing universal carbon capture and storage. You will want to read Robert Socolow's Can We Bury Global Warming? from Scientific American (July 2005) for more details (unfortunately, not freely available online). For our edification, here is the world's projected CO2 emissions growth out to 2020.
Weyburn and Overall Anthropogenic Carbon Emissions
On November 15th, 2005, Samuel Bodman's office announced Successful Sequestration and Enhanced Oil Recovery Project Could Mean More Oil and Less CO2 Emissions with the subtitle "Weyburn Project" Breaks New Ground in Enhanced Oil Recovery Efforts.
As applied to Weyburn, an additional 130 million barrels of oil will produce 43.8 million mt of CO2 emissions. On the other hand, if 30.0 million mt are sequestered, only 13.8 million mt will be emitted. This is obviously greater than zero. And in fact, the Weyburn case study says as much regarding their pilot project.
1. Does the Weyburn experiment extend to new (primary or secondary) oil production, deepwater or land-based? -- No.
2. Is carbon capture (as in the Beulah coal gasification facility upstream from Weyburn) viable yet? -- Yes, technologically, but really no, not without laws mandating that CO2 emissions be penalized to justify the higher costs of capturing the carbon, costs which will be passed on to consumers. See the Socolow article in Scientific American cited above.
3. Is carbon capture of fossil fuels industrial production sites better than using a natural CO2 source? -- Sure. Here's from Cascio again.
5. Does the Weyburn process (universally applied) have a large impact on reducing total anthropogenic emissions from fossil fuels? -- Absolutely not but it does create a very small % reductions in emissions.
Finally, where does that leave us? Plainly, it leaves us with 130 million barrels of new oil (6.5 days of US consumption) and 13.8 million mt of new CO2 emissions (with a lifetime in the atmosphere of about 100 years), proving that you can not have your cake and eat it too or, to put it another way, there is no free lunch. There are three doors here, as when Monty Hall says Let's Make A Deal. Behind door #1, we can mitigate climate change by not producing the additional Weyburn oil at all, cutting demand and switching to alternative energy sources, thus reducing overall anthropogenic CO2 emissions and allowing, we hope, a reasonable future life without major recessions or (God Forbid) dieoffs. Or behind door #2, we can produce the oil with a tiny cut in the CO2 emissions growth rate in a business as usual scenario with growing demand. This staves off scarcity that would produce worldwide recessions in the near term but will certainly exacerbate climate change and energy depletion in the longer term, thus postponing the suffering until later. Behind door #3, we can implement energy alternatives now, reduce demand and produce the oil with sequestered CO2 as at Weyburn. This would be best if carbon capture and storage programs could be greatly explanded worldwide. However, successful pilot programs like Weyburn, while a small step in the right direction, are not going to cut the mustard if we're going to achieve the CO2 emissions reductions necessary to avoid catastrophic climate change.
Explained Hamal Salah al-Baker of Qatar Petroleum, "our wells are flowing and we're just now putting some on waterflood. But there are predictions of declines by 2020," at which time, he said, Qatar's oil output would be "zero."On the other hand, there are only really two strategies for mitigating climate change--switching to alternative energy sources (nuclear, wind, solar, biofuels) that emit little or no carbon into the Earth's atmosphere or sequestering carbon underground as we continue to use fossil fuels.
"If we can use CO2 (to increase oil recovery), we can prolong production 20 years," he continued, adding that his country also has a number of natural gas facilities that have produced an excess of CO2 as a byproduct.
"Because of environmental regulations, we have to get rid of it," he said.
CO2 Injection for EOR
As reported by the DOE, CO2 Injection into oil fields is a tertiary recovery technique first tried in Scurry County, Texas in 1972.
Since then, CO2 injection has been used successfully throughout the Permian Basin of West Texas and eastern New Mexico (where about half of all the CO2 floods in the world are located). The technology has been pursued to a limited extent in Kansas, Mississippi, Wyoming, Oklahoma, Colorado, Utah, Montana, Alaska, and Pennsylvania.As applied to Weyburn, this graphic makes clear the role of CO2 injection in the production lifetime of an oil field.
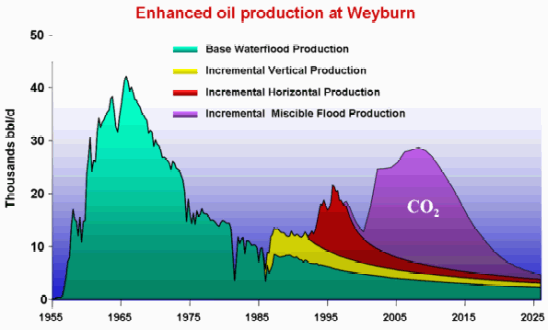
Examining the Weyburn EOR graphic, water injection was used first, later some new vertical wells were drilled (yellow area), followed by horizontal infill drilling (the red area) in the 90's to offset declines after production had considerably fallen off. Finally, CO2 injection was tried beginning in the year 2000. As in all tertiary recovery, this technique was used to amplify and extend the tail of an oil field's production to extract "stranded" oil in place. As TOD contributor westexas can no doubt tell you, use of tertiary recovery generally has not fundamentally altered the shape of the Hubbert Linearization for lower 48 US oil production but has marginally increased that production by extending the tail of the linearization curve into the future. As the DOE reports (link above), "Today, less than 700,000 barrels per day of oil is produced in the United States by EOR processes (compared to about 6 million barrels per day of total domestic production). Thermal techniques [another tertiary EOR strategy] account for over 50 percent of U.S. EOR production, primarily being applied in California; gas injection [CO2 in this case] accounts for nearly 50 percent." For Weyburn, CO2 was determined to be the "solvent of choice" and is only used in those parts of the oil field where this is the case. See Role of Enhanced Oil Recovery in Carbon Sequestration--The Weyburn Monitoring Project, a case study (pdf).
CO2 Capture and Storage (CCS) aka Carbon Sequestration
Carbon sequestration is the capture, from power plants and other facilities, and storage of carbon dioxide (CO2) and other greenhouse gases that would otherwise be emitted to the atmosphere. The gases can be captured at the point of emission and can be stored in underground reservoirs (geological sequestration), injected in deep oceans (ocean sequestration), or converted to rock-like solid materials (advanced concepts).What we are primarily interested in here is geological sequestration as used at Weyburn. As this New Scientist article states (not freely available), the possibilities for storing the carbon include
Fossil fuels will remain the mainstay of energy production well into the 21st century. Availability of these fuels to provide clean, affordable energy is essential for global prosperity and security. However, unless energy systems significantly reduce the carbon emissions to the atmosphere, increased atmospheric concentrations of CO2 due to carbon emissions are expected.
- deep unused saline reservoirs
- active wells for EOR (as at Weyburn)
- depleted oil & gas reservoirs
- deep unmineable coal seams
- CO injection to drive out coal bed methane
- other options (basalts, oil shales, cavities)
Weyburn is an ideal experiment. Not only do such carbon capture processes not exist at all current and planned industrial production sources (again, for example, new coal-fired power plants in the US or China) but also, even if they did, there are no programs for transporting the CO2 to places where geological sequestration could take place if the infrastruture were in place to do so. These are the problems the world faces in implementing universal carbon capture and storage. You will want to read Robert Socolow's Can We Bury Global Warming? from Scientific American (July 2005) for more details (unfortunately, not freely available online). For our edification, here is the world's projected CO2 emissions growth out to 2020.
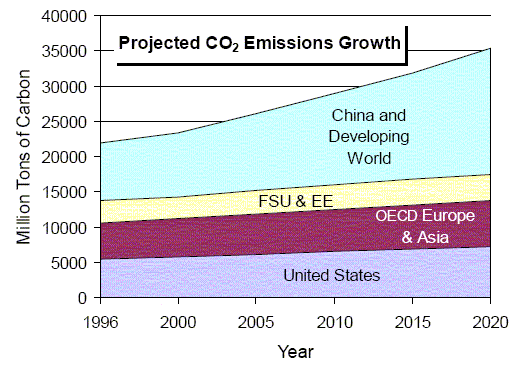
Weyburn and Overall Anthropogenic Carbon Emissions
On November 15th, 2005, Samuel Bodman's office announced Successful Sequestration and Enhanced Oil Recovery Project Could Mean More Oil and Less CO2 Emissions with the subtitle "Weyburn Project" Breaks New Ground in Enhanced Oil Recovery Efforts.
Enhanced oil recovery (EOR), the technique used in the project, has the potential to increase an oil field's ultimate oil recovery up to 60 percent and extend the oilfield's life by decades. Scientists project that, by using knowledge gained from the Weyburn Project, the Weyburn Oilfield will remain viable for another 20 years, produce an additional 130 million barrels of oil, and sequester as much as 30 million tons of CO2.Now, the obvious problem was pointed out by Jamais Cascio in his post (Engineer Poet's link) Seeing the Forest for the Trees: Sequestration and Oil Production.
I'm quite certain that you folks have already picked up on the key underlying problem. The additional barrels of oil put out carbon dioxide even while the sequestration buries it. In fact, as I show in the extended entry, the additional oil puts out more CO2 than is buried. The Weyburn sequestration model is a study in the need to pay attention to the trade-offs involved in quick-fix solutions to big problems.Cascio did some very rough back of the envelope calculations to try to prove his claim but I decided to be more precise. Here's how I did that. Based on the year 2001, the US Emissions from Petroleum were 2418.6 teragrams CO2 Equivalent. See Table 2-3. One teragram = 1 million metric tons (mt). EIA's 2001 data indicate that total oil consumed in the US that year was 7.17 billion barrels (19,449/kbd). For the US, this turns out to be 0.337 mt CO2 emissions/barrel of oil consumed.
As applied to Weyburn, an additional 130 million barrels of oil will produce 43.8 million mt of CO2 emissions. On the other hand, if 30.0 million mt are sequestered, only 13.8 million mt will be emitted. This is obviously greater than zero. And in fact, the Weyburn case study says as much regarding their pilot project.
For the produced oil, on a life-cycle basis, it has been estimated that CO2 production at end use will be two-thirds that of oil produced in a conventional fashion [meaning using CO2 injection without sequestration].According to my calculations, the ultimate CO2 emissions are reduced by 0.68% (about two-thirds)--this is in accord with their numbers, thus substantiating their claim and this analysis. Here's a small Q&A on the meaning of it all.
1. Does the Weyburn experiment extend to new (primary or secondary) oil production, deepwater or land-based? -- No.
2. Is carbon capture (as in the Beulah coal gasification facility upstream from Weyburn) viable yet? -- Yes, technologically, but really no, not without laws mandating that CO2 emissions be penalized to justify the higher costs of capturing the carbon, costs which will be passed on to consumers. See the Socolow article in Scientific American cited above.
3. Is carbon capture of fossil fuels industrial production sites better than using a natural CO2 source? -- Sure. Here's from Cascio again.
As Watthead correctly points out, the use of CO2 as a means of enhanced recovery from oil fields is not new; the difference in the Weyburn project is that the CO2 used comes from industrial output, and would previously have been released into the atmosphere (past CO2-based recovery used carbon dioxide removed from naturally-occurring underground pockets). If the choice is between sequestering some atmospheric CO2 for oil recovery versus no sequestration (but continued enhanced oil recovery), sequestration is clearly a better result. But as the DOE release points out, the use of natural pockets of carbon dioxide happens at "considerable expense" -- oil companies would almost certainly start using industrial/atmospheric CO2 regardless of the environmental benefits (such as they are) for cost reasons alone.4. Does the Weyburn process reduce future CO2 emissions even if universally applied? -- Only in those special cases where CO2 injection is appropriate for EOR at the tail end of an oil field's production. This will depend on the field's geological characteristics.
5. Does the Weyburn process (universally applied) have a large impact on reducing total anthropogenic emissions from fossil fuels? -- Absolutely not but it does create a very small % reductions in emissions.
Finally, where does that leave us? Plainly, it leaves us with 130 million barrels of new oil (6.5 days of US consumption) and 13.8 million mt of new CO2 emissions (with a lifetime in the atmosphere of about 100 years), proving that you can not have your cake and eat it too or, to put it another way, there is no free lunch. There are three doors here, as when Monty Hall says Let's Make A Deal. Behind door #1, we can mitigate climate change by not producing the additional Weyburn oil at all, cutting demand and switching to alternative energy sources, thus reducing overall anthropogenic CO2 emissions and allowing, we hope, a reasonable future life without major recessions or (God Forbid) dieoffs. Or behind door #2, we can produce the oil with a tiny cut in the CO2 emissions growth rate in a business as usual scenario with growing demand. This staves off scarcity that would produce worldwide recessions in the near term but will certainly exacerbate climate change and energy depletion in the longer term, thus postponing the suffering until later. Behind door #3, we can implement energy alternatives now, reduce demand and produce the oil with sequestered CO2 as at Weyburn. This would be best if carbon capture and storage programs could be greatly explanded worldwide. However, successful pilot programs like Weyburn, while a small step in the right direction, are not going to cut the mustard if we're going to achieve the CO2 emissions reductions necessary to avoid catastrophic climate change.
Such discussions and a good bit of modeling and experimentation are underway at Los Alamos National Labs...Los Alamos Press Release
Now, don't get me wrong - I am highly skeptical of the feasibility of such a setup, both economically and thermodynamically...
But, absent any other alternative, and given that the climate seems to have reached a tipping point - or will soon - it seems to me that the only real way to go is remediation - "terraforming" as it were.
There are some folks thinking about bioremediation, and others working on mineralogical (thin films reacting with force-fed (solar or wind pump) atmosphere at 370 ppm CO2 making solid MgCO3 or CaCO3) remediation...and that, to me seems about the only way to go...unless we can stop producing excess CO2 and kick the hydrocarbon habit...neither of which, frankly, seems very likely right now.
A fine idea. IF you have excess electromotive force with nothing to do.
That is a big IF.
I spent a day looking into a basic set of Eq. to do the sealand process. I was looking at over $250,000 to set up with wind generators, no land costs, and a spitballing of the reaction/process cost to make Methonal. 100 Liters a day production.
You might be able to count on selling Nitrogen Gas/Liquid Nitrogen, until there is mass Methonal production (oversupply of N2) or a reduced industrial need for Nitrogen (via recession) You might be able to turn an additional buck splitting water, taking the H2 and combining with your excessive N2. But that model needs cheap, excessive energy.
http://www.glencoe.com/sec/science/chemistry/mc/pow/chapter18.shtml
in a cyclic process.
There's some research I just got the goods on (direct carbon fuel cells) which is pretty much ideally suited for a cycle which does just that. I hope to be blogging that as soon as I get enough think-time to organize it.
How to get those various oxides WITHOUT resorting to methods that release CO2?
Grind up mineral deposits.
Where to get those mineral deposits, specifically some of the more exotic ones?
Ophiolites and the ocean floor.
MnO, MgO, FeO and some of the other weirdo oxides are found in abundance in those geologic settings.
-Does CO2 injection increase the overall percentage of recovered oil?
-If so, then why does it not effect the Hubbard Linearization?
-Can the CO2 become disolved in the oil (like carbonated water)? If so, the guesstimate for stored CO2 is high.
-Does CO2 injection (or any gas injection) damage the geologic structure of the rock?
-Doesn't tertiary recovery methods just mean we have a more severe depletion rate once it rolls over again?
HeadingOut, can we get a lesson on CO2 injection?
A bibliography is included.
This is an interesting story. One thing we didn't discuss in our article is sequestering CO2 in the deep ocean. This would mean pumping CO2 into the ocean where it would freeze, forming a solid that could be stored indefinitely on the bottom of the sea. The CO2 does not dissolve. This mirrors the natural process of hydrate formation, which has trapped some 1,500 trillion cubic feet of methane on the ocean floor.
Thanks for an interesting story,
/papa
Any credible energy balances out there on this process?
The main drawback would be the investment to install the equipment. The stuff process a wet corrosive stream so plain old cheap carbon steel tends to be a bad choice.
Like CaO and MgO, KOH does not occur in nature and takes a nontrivial amount of energy to separate from its natural mineral sources. Even in the LANL study, the author tells us "The calcium carbonate is then heated ...", releasing the captured CO2.
Another way to separate CO2 from flue gases would be to compress and cool them, letting the lighter N2 escape from the mix. This too is an energy-hogging process. (Note that liquid CO2 only exists under pressure -- at one atmosphere, the solid sublimes somewhere around -80C.)
As for anions, you probably really have an HCO3- <-> CO3-- equilibrium going on between your stack and stripper. And I'd expect some of the acid ions to be more stable than others. So give or take some sulfate, I'd expect at least some of that system to be consumed/degraded although perhaps a small percentage.
The big-picture problem is that the combustion process uses a train-car load of coal in an hour and produces three times that, by weight, in CO2. However you remove it, you're going to need a lot of equipment and significant quantities of chemicals, stainless steel, pumps, compressors, electric power, etc. to run all that.
And, with all said in this thread, at least it should be easier to sequester it from flue gas than try to extract the 0.002% of it from the atmosphere in general.
Basis:
This is a LOT of CO2 and a large volume of gas to try to inject into the ocean!
If you attempt to send it to the ocean via a pipeline and then try pumping it to a low depth, you will incur a huge energy consumption just as the result of the work involved in compressing the CO2 to the pressure existing in the ocean at the depth you wish to sequester it.
On the other hand, if you first attempt to liquify the CO2 and then inject it into the ocean as a liquid, you will also incur a similarly huge energy consumption due to the work required for liquifaction.
Or to put it into perspective in another way, if you were to ship the compressed CO2 via 50-ton rail cars, you would need a train of some 128 rail cars each and every day that the power plant is in operation .... and this is just one medium-size power plant!
To me, this whole thing looks like a VERY bad idea.
I am totally with you on this. The numbers just don't make any sense thermodynamically for a real fossil power plant. I doubt it will ever happen.
Another problem is even if one burned pure carbon (coke?), the exhaust would be only 20% CO2 and 80% nitrogen at a perfect fuel/air ratio. In real plants, one burns a bit lean to ensure complete combustion and to suppress NOX production, making it even more dilute.
You pump a lot of inert N2 (four times as much) to even MOVE the CO2.
While using CO2 for enhanced oil recovery is good, don't think you ever get it as a byproduct of fossil fuel energy production.
Of course, coal is TODAY more capital-intensive than nuclear, once all the pollution control hardware is added.
Put ANOTHER stake through its heart for me.
- Oxygen-blown IGCC and carbon capture/storage as part of syngas cleanup.
- Gasification and complete shift-conversion to H2, scrubbing and separating the CO2.
- Direct-carbon fuel cells, which produce nearly-pure CO2.
Pulling CO2 out of the flue gas from atmospheric combustion in air is like putting lipstick on a pig.On top of that, it boosts efficiency by roughly 20%. If carbon is co-captured with H2S and co-sequestered, I understand it can be cheaper than the standard methods.
Regardless, we're using an average of about 225 GW of coal-fired power. If it costs $1000/kW to repower old plants (assuming that we build air-separation plants, filters, scrubbers and other parts on assembly lines) that would be $225 billion total. Do that over 15 years, it's only $15 billion/year or about $50/capita/year. Cheap.
Specialized heat recovery steam generators or whatever the boilers are called have a larger heat exchange area due to the much larger gasflow with a smaller temperature difference between inflow and outflow of gas.
If you did this over 15 years and allowed for 1%/year growth in output, you'd repower 40% of the plants and multiply their output by 2.9 times each (total 116% of original output) and retire the other 60%. Coal consumption would drop about 4-5%, and it would be feasible to sequester roughly half the carbon from those plants sited near exhausted oil and gas fields and the like.
Has anyone else around here heard of the notion of seeding the Pacific southern gyre with micronutrients and letting the resulting algal bloom sequester CO2?
Several test runs have been conducted, and were a wash - the enhanced productivity caused by nutrient seeding is more than offset by a) the nighttime half of the photosynthetic cycle and b) by the impact of the dying and decomposition of the resulting algal/plankton bloom.
BTW, did anyone catch yesterday's LA Times (or day before?) on Arnie's energy dilemma: Follow the state mandated reduction in CO2 or import electricity from coal burning plants in OTHER states. Anyone care to bet on Arnie turning down the latter? I'm offering 100 to 1 odds.
Geo
But not quite. ;-)
To bubble this amount of flue gas through some sort of aqueous bio-reactor, even if only a shallow basin, say 12 ft deep, would require an enormous amount of pumping power, something on the order of 15,000 HP. Then one has all the additional energy required to separate, dewater, and process the algae, not to mention waste handling. While this scheme may be better than chemical scrubber, it's not obvious to me that there is any net gain involved.
Besides, isn't the carbon content of the CO2 transferred to the algae eventually going to be re-released as CO2 when whatever fuel derived from the algae is burned? Not to mention the CO2 released during the respiration phase of the algae. It seems like a machine that just goes around in circles and doesn't really do anything.
I remain highly skeptical.
I know a guy who has spoken to the folks doing this. The comment that he made was that doing this on a power plant scale would require phenomonal amounts of plastic tubing for the photobioreactors.
At this point there isn't much that they say publicly so it is hard to really analyize the thing in any amount of detail. At this point it remains one of those things that would be interesting if it happens to pan out.
Regarding carbon, yes the CO2 eventually ends up in the air. It just takes a more circuitous route. I guess another way of looking at it is that you effectively end up displacing some petro-diesel where everything else remains pretty much the same.
It isn't so much that the thing goes round and round in circles either - the photobioreactors require sunlight for the algae to grow.
The thing that got me thinking about this was the discussion about Weyburn and all of the pitfalls and problems of more conventional sequestration.
To give you an idea of where they stand with this, they got a little writeup back in Sept:
http://www.masshightech.com/displayarticledetail.asp?art_id=69603&search=
Now, in any bio-reactor the upward flow of air cannot be too great, for the simple reason that it takes time for the biomass growing on the surfaces of teh media to absorb the gases as they come in contact with it. Thus, a typical gas flux through the media is in the low CFM/sq-ft range. Just to illustrate the requried size, let us be generous and use a gas flux of say 30 CFM/square foot (this give an apparent velocity of less than 1/2 ft/sec, which represents a reasonably gentle rise through the tower ... it could be more and it could be less, but this is a decent ball park number).
So, if our 300 mega-watt power plant has a flue gas flow rate of 545,000 CFM, then the required surface area of the bio-reactor chamber would be about 18,100 sq-ft. If the bio-reactor were circular, that would be equivalent to a vessel or chamber 480 ft in diameter (or more likely, several smaller vessels with equivalent total area). As it would have to be at least 12 ft deep (probably much more), this represents some humongous equipment, particularly when the chamber is filled with plastic media. And this doesn't even take into account all the equipment to handle and process the algae that you grow.
I still have a very hard time picturing this concept as being even marginally practical on a large scale. It may be useful in garnering an academic-based start-up company some government development money, and it may be useful in keeping some graduate students busy, but I don't think it has a prayer in the real world for large scale power plant applications.
That is still a lot of vessel, and in fact I think that considering the facxt that the C02 concentration in flue gas is typically between 10 and 14% by volume, depending on amount of excess air, the air flux of 30 CFM/sq-ft is probably overly generous, and in reality one might have to use a flux of less than half that in order to get good absorption. In which case, the required vessel area would double, leaving us with a vessel of approx 215 ft.
The point I am trying to make with all these back-of-envelop calculations is that CO2 sequestration of power plant flue gas would require a truly massive amount of capital equipment, plus would entail a significant energy drain, once one takes into account the handling, transport, and processing of huge amounts of material. This, by the way, is a problem endemic with most pollution control schemes: you have to deal with a very large volume of near worthless material.
http://mobjectivist.blogspot.com/2005/12/canadian-production-model-weyburn.html
Isn't it overwhelmingly obvious that saving energy is a huge amount less costly (in every sense of the word) than generating it to be largely tossed out the window by really stupid uses?
I am sitting here in about 15C room with my fur hat on feeling quite comfortable, and burning less wood than I did when I was sitting here without my fur hat on at 23C. And doing a lot less work stuffing wood into the stove.