Current and Projected Costs for Biofuels from Algae and Pyrolysis
Posted by Robert Rapier on June 4, 2012 - 10:17am
A reader recently called my attention to a new and very interesting presentation from the Department of Energy’s Biomass Program:
The presentation explored the question of whether the U.S. government is spending money on the right technology pathways. Costs were presented for biofuel produced from pyrolysis, algae, Fischer-Tropsch (FT), and methanol-to-gasoline (MTG) routes.
I want to share several slides from the presentation to give an idea of what the DOE thinks about the costs for producing biofuels via the various pathways. The first slide below shows the projected cost of production of biofuels via MTG, pyrolysis, and FT for the “Nth Biorefinery Plant” — which is defined as the projected fuel cost after a number of plants have been built and the learning curve has been mastered.
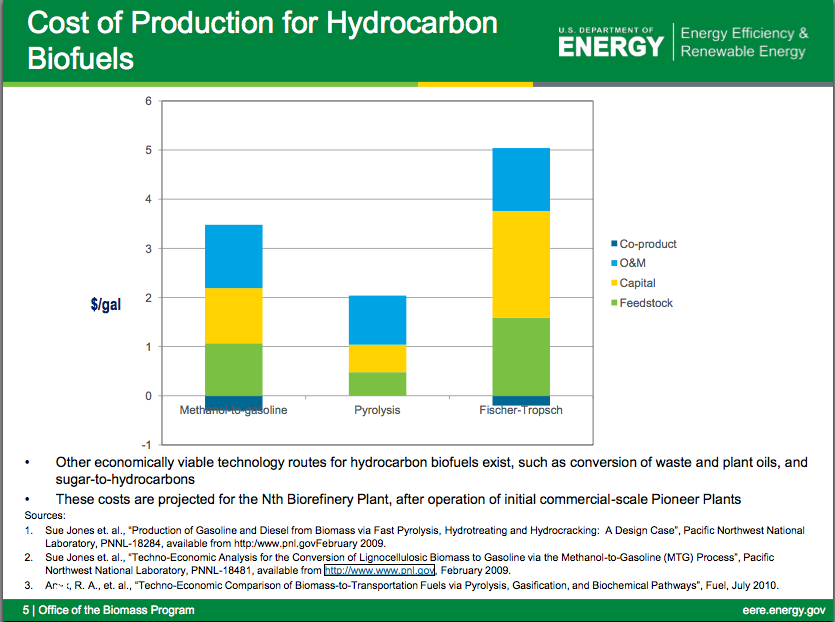
This slide projects a future best case scenario of about $3.50/gallon for the MTG route, $2/gallon for the pyrolysis route, and $5/gallon for the FT route. So if that is for the Nth plant, where do costs currently stand?
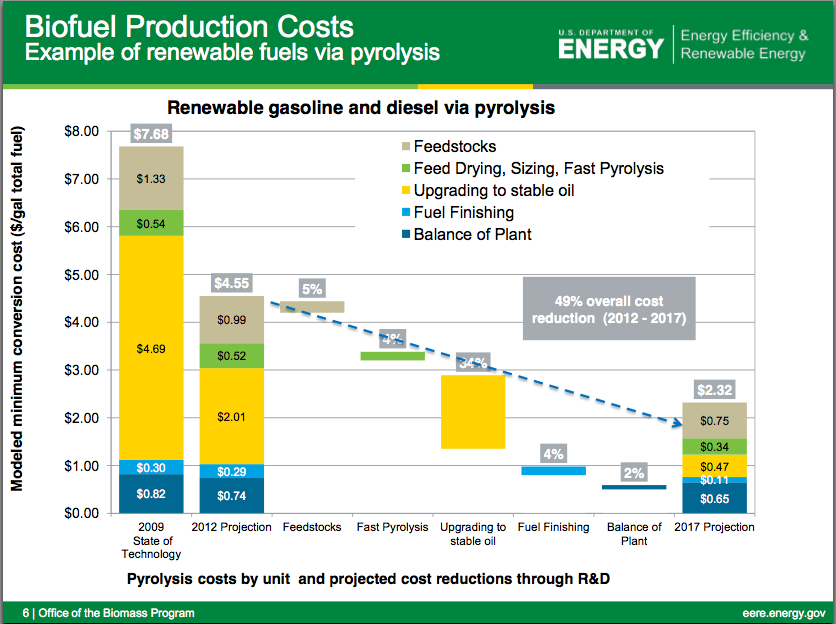
This slide shows that in 2009 they were estimating costs of production for biofuel based on pyrolysis of $7.68/gallon. By this year (2012) they projected the cost dropping to $4.55, and then over the next 5 years they project costs will fall to $2.32 (again, the Nth plant cost for pyrolysis was projected at $2.00/gallon). They project that the largest savings will come from the upgrading step.
So what do they say about fuel from algae?
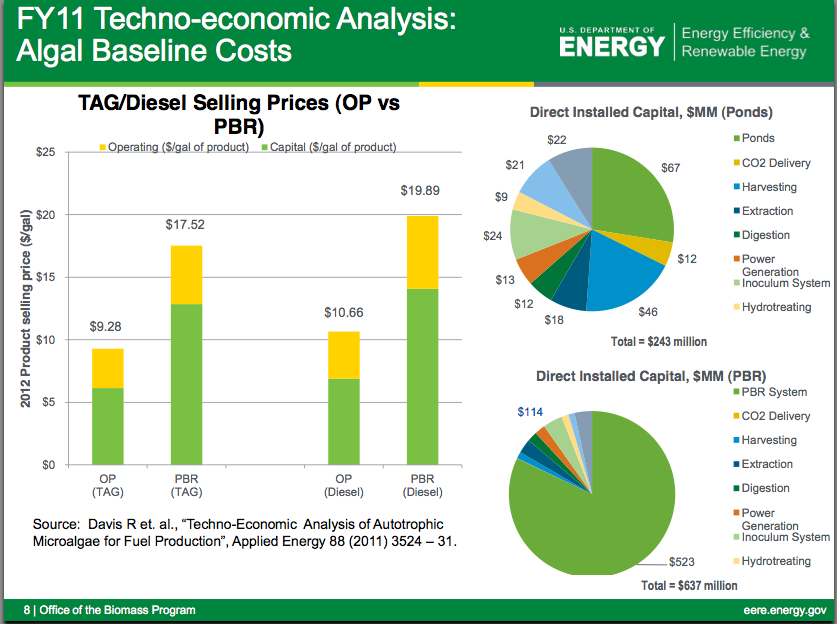
This slide shows the 2012 selling price for algal products in four categories: Triglycerides (TAG) from open ponds (OP) at $9.28/gallon and from photobioreactors (PBR) at $17.52/gallon, and then the finished diesel (which requires hydrotreating the TAG) at $10.66 from OPs and $19.89 from PBRs.
The following slide projects future algal fuel costs under a number of different scenarios:
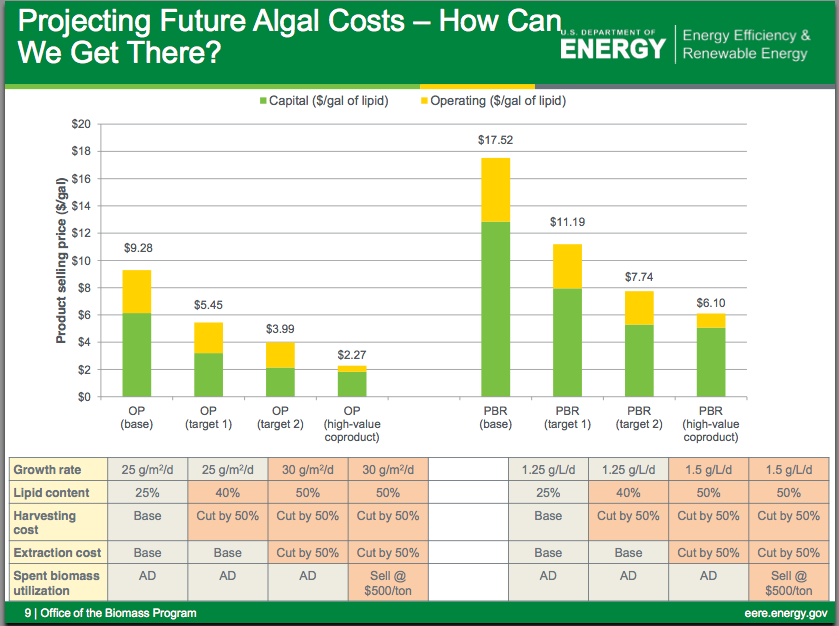
In each case they assume various improvements over the base case, with the final case for both open ponds and photobioreactors being one in which a high value coproduct is produced.
So what are we to make of these slides? First, as I have said in the past, I don’t believe photobioreactors are the future of algal fuel production. Those artist renderings of futurist algae farms such as this one are pure fantasy in my opinion:
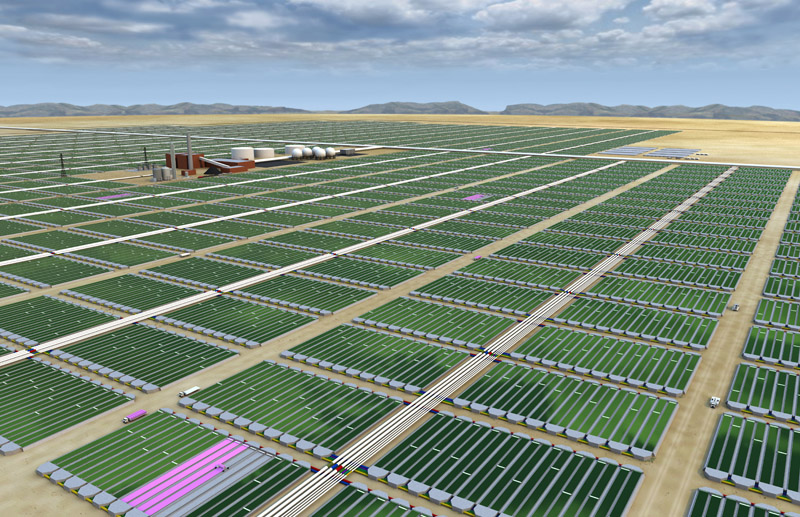
In the most optimistic case the DOE could only get the projected cost of the fuel down to $6.10. More conservative assumptions would project that the fuel derived from PBRs will still be more than $10/gallon. As algae expert John Benemann noted here in a guest essay, “The use of closed photobioreactors…is totally absurd.”
Open ponds show more promise, but algae has been grown in open ponds for many years. Some areas that are specific to fuel production might see some significant cost savings, but other areas have already had decades to work on lower costs (e.g., harvesting). I suspect that Target 1 for open ponds might be achievable ($5.45/gallon) but lower than that will be challenging.
The fermentation route that Solazyme utilizes was not covered, but it would be interesting to see how that stacks up. The cost of converting the TAG to diesel should be about the same (~$2.40/gallon), but I know that Solazyme believes that their productions costs will beat both the open ponds and PBR routes.
But I think the real story from this presentation is the DOE’s projections of the pyrolysis to fuel route. They clearly believe that this route can ultimately be competitive with petroleum. The technology currently exists to convert pyrolysis oil into transportation fuel, but it is fairly new and therefore should have room for some improvements. This is the type of route that KiOR is pursuing. A partnership between UOP Honeywell, Ensyn Corporation (those two formed a JV called Envergent) and Tesoro was awarded a DOE grant to build a demonstration facility based on pyrolysis at Tesoro’s refinery in Hawaii.
The overall ranking in terms of future costs would appear to be: pyrolysis < MTG < FT < OP algal << PBR algal.
Link to Original Article: Current and Projected Costs for Biofuels from Algae and Pyrolysis
Robert, I'm not seeing any breakdown for feedstock and transportation costs. Am I just missing something?
Jon, I am traveling at the moment and don't have time to dig into that. I think they presumed an all inclusive feedstock cost for the various options, but as I indicated in previous essays I think "cheap biomass" assumptions will be the downfall of many renewable energy projects.
because this fails to includes the depreciation arising from creating the bio mass feedstock in the first place? fertilisers etc?
This has already happened in Thailand. Biomass is often a waste product with no value. So developers plan around a very low cost. However, as demand grows so does the price.
Rice husks in Thailand were free in 2000, cost $12/ton in 2004, $25/ton in 2007, and $35/ton in 2010.
I am disappointed they didn't go further into FT. With the Auzzies coming to Canada with ISCG(In-situ Coal Gasification), I would imagine that cost of a FT bbl will drop significantly. The ISCG process creates a gas flow that is >50% CO/H2/CH4 with that stream roughly 80/20 (CO/H2)/CH4. The make up of this gas flow should help FT projects bypass expensive SMR (Steam methane reformers) and reduce capital cost. Likewise monetizing ISCG through FT makes the most sense as they can utilize ISCG's product stream almost right away, also less capital cost then building a power plant/converting CO/H2 -> CH4.
I'm a climate scientist who's actually pretty pessimistic and pragmatic about controlling greenhouse gases. But in-situ coal gasification terrifies me. Our estimates for future CO2 emissions are based on the idea that most of the coal in the world can't be economically extracted. Being able to burn it all -- while wasting half of its energy content -- could lead to some very scary climate change.
Fischer-Tropsch all you like with biomass, but leave the coal where it is.
Could that CO2 be injected back in? It's a single point source with an empty underground reservoir beneath it. Maybe it could be piped to a previous in-situ coal gasification site that is no longer active.
AFAIK, there's nothing that makes what's left over after in-situ gasification of a coal seam a good candidate for sequestration of CO2. Unminable coal seams are often mentioned as good possibilities for sequestration, but that's because coal remains there. It's porous and has an affinity for both CO2 and methane. Flooding the seam with CO2 flushes the coal bed methane (CBM) and leaves behind CO2. The extracted mix of CO2 and methane gases is processed to separate the gases, with the CO2 recycled back to the coal seam.
If the coal seam is burned away by ISCG,there's nothing left to hold onto the CO2. The formation collapses, and the fracturing of overlying rock layers creates escape paths for residual gases. Also has a tendency to let in ground water, which picks up mobilized heavy metals and can diffuse upward to pollute aquifers.
At least, that's how I understand it. Not an expert, however.
Actually another reason to go ISCG is to extract the CO2. CO2 is finding it's way into oil reservoirs across Canada, only problem is finding a constant supply. From my understanding this would bypass the CO2 emission issue. Think the Weyburn oil field CO2 flood, "carbon capture program".
"From my understanding this would bypass the CO2 emission issue".
Usually not true for EOR.
I don't think you read yesterdays post by Heading Out: Tech Talk - The Potential For Saudi EOR.
In that discussion HO explained that if CO2 is injected into an oil reservior with good permeability, the CO2 mixes with the oil and comes out the wellhead. Most is not sequestered or held in the resevior, but travels from the injection well throught the rock, picking up trapped oil and carrying it out the production pipe. Perhaps a gas-oil separation plant could recover some of this CO2. But putting CO2 into a producing oil well for Enhanced Oil Recovery purpose does not remove CO2 from the atmosphere on a permanent basis. If I am wrong perhaps someone like
ROCKMAN can correct me.
My understanding is that no particular effort is needed to separate CO2 from oil at the surface. It's only supercritical CO2 that is miscible with oil. When the oil gets to the surface and is depressurized, the CO2 "fizzes out". It's then mixed with makeup gas from the CO2 source and sent back down. The overall process does end up leaving a lot of supercritical CO2 in the formation. Substantially more, by mass, than the additional oil recovered.
I'd also like to have seen more on FT options. Not that I'm a fan of ISCG, but I've heard of alternatives to the classic FT approach used with such awful financial results in the Pearl GTL plant. The alternatives supposedly are more selective for shorter alkanes and produce liquids directly, as opposed to waxes that then have to be cracked and refined. I'd like to know if there's anything to those reports.
Hmmm I haven't really heard much about upgrading to shorter alkanes. I imagine it would reduce the need for a hydrogen cracker? Likewise what length chains are we talking about here? It would be economic suicide to upgrade to C3 or C4...
Roger how much do you know about the Pearl GTL plant? Why was it such a financial disaster? Was it just the size of and complexity of the project? I ask this as I have seen recent estimates of flowing barrel capital costs of $70,000 - $50,000 for FT facility. At those numbers and these gas prices / diesel prices economic projects exist. Problem in my mind is getting the logistics and distribution down.
What exactly were these "awful financial results" of the Pearl GTL plant? My understanding is that the Pearl GTL project was financed by Royal Dutch Shell. And according to the ownership agreement it has with state-owned Qatar Petroleum, Shell is entitled to first recover its entire $19 billion dollar USD investment cost. Then Shell will also receive a share of all subsequent profits from the Pearl plant.
The Pearl GTL plant was completed nearly on schedule - despite being one of the largest hydrocarbon projects ever attempted. The plant has now been operational and shipping finished GTL-based products for roughly one full year. The plant is designed to produce 140,000 BOE per day of very clean, high-quality GTL products like gasoil (for low-sulfur diesel), naptha for gasoline, kerosene for jet fuel, and base stock lubricants for motor oils. There is also daily production of another 120,000 BOE of LPG, condensate and ethane. So there is a total daily production potential from the plant of 260,000 BOE.
This level of output is based on 2 GTL trains, and the Pearl plant can also accommodate a 3rd such GTL train, giving Shell the option to add a future 50% increase in Pearl GTL capacity.
Shell estimates that the Pearl GTL plant, along with a smaller $2 billion dollar LNG project in Qatar, will produce over $6 billion dollars per year in profits. And Shell says that this estimate is based on a crude oil price of $70 dollars per barrel. Obviously, oil remains well above the $70/barrel rate, therefore Pearl GTL profits should be running that much higher.
Shell also estimates the lifetime production from the underlying natural gas fields supplying Pearl GTL will be the equivalent of about 3 billion barrels of oil.
http://www.hydrocarbons-technology.com/projects/pearl/
http://www.thenational.ae/thenationalconversation/industry-insights/ener...
NOTE: I am not, and have never been, associated with Royal Dutch Shell in any way whatsoever.
I think the most shocking thing about what you have posted, assume it is true, is their operating costs of $6.77. If you take 6,000,000,000/260,000/365=63.22 as an operating netback, subtract $70bbl vola! I am assuming this means they have a deal with the Saudi's to hit project payback before paying for gas?
Using the 19 billion for capex here is what I get as a payback period. Capital intensity of 73,000, using netback of 60, pay back is achieved in 1217 days or 3 years and 4 months. Course all things considered your NPV will take longer, but I imagine another year or two or double the oil price price does the trick. Looks like an attractive project if they can keep it running. Anyone else have a take on Pearl?
The plant has been hugely more expensive that expected. According to the Wikipedia entry, and remembered bits from elsewhere, in 2003 the complex was projected to cost $5 billion; by 2007, costs had escalated to $18 billion, and the project was still incomplete. It is operating today, but at less than the original design target.
The track record of that project has killed off many other GTL plans that were in the works. I would have to do more digging to learn more about what went wrong, but what I've heard is that Shell tried to scale up the FT technology that SASOL has been using, and ran into a host of scale-related problems that they hadn't anticipated.
This is pure speculation on my part, but I suspect that the design approach for the plant was determined in part by company politics at Shell. I know that years ago, there had been what might be dubbed a GTL "scale war" between Shell and a couple of upstart competitors. I've forgotten the names, but the competetitors were pushing small scale equipment, I think using microchannel reactors. Shell insisted that that approach was not viable, and that the only way to make GTL cost-effective was to go big for "economies of scale".
I've long considered "economies of scale" a kind voodoo phrase that appeals to senior managers and others who don't really know what they're talking about. Not that economies of scale don't exist; they certainly do, but they're complex and not automatic. There's no substitute for actually knowing what you're doing.
I tend to agree with you. What fiancial disaster. It would only be a finacial disaster to Shell if they had to pay a market price for the gas which they don't. I do not like this type of project for other reasons namely the CO2 footprint for the plant and therefore the products CO@ footprint. However when you consider the waxes, the production of these waxes might be most economically done in terms of CO2 with a FT step. As for fuels then some further processing is necessary usually in the form of isomerisation to improve the cold flow properties.
For gasoline production FT is not really suitable as the gasoline fraction octane number is very poor, and extensive prcessing would be necessary, such as platforming, FCC of hydrocracking. There are a number of options available. For chemicals the waxes can be cracked in a steam cracker for the production of light olefines.
There are two types of FT. Low temperature (cobalt catalyst)and high temperature (iron catalyst). In both cases the products are a distribution of molecular weight known as an Anderson Schulz Flory distribution, which is a sort of bell shaped curve, with from memory the starting point at about C4. Some attepts have been made to use zeolites to reduce the chain length but I do not know of any commercial plant. The Shell process is the LT FT and is highly optimised having an overall carbon efficiency of around 87-82%(Myers Handbook of Petroleum Refining Processes). Sasol are the experts with HT FT and produce some chemical products with this route, namely olefines, alcohol and acids. By altering the H2 to Co ration the production of lower olefines, alchols and acids can be achieved, but few have vebtured down this path. Again the products are a distribution and higher CO concentrations favour oxygenate production.
An indirect FT route is via methanol. Olefines and gasoline can be produced by this route. The methanol is decomposed to light olefines and Mobil have a process for MTG using a SAPO catalyst. I do not believe that there is currently a running plant on MTG.
In all cases the total global population of FT plants is small, very small. Personally I think it will always be a niche process and suffers from very high capital costs and complexity which perhaps confirms why there are so few plants globally. It is difficult to justify a coal process. Gasification of biomass is for dreamers in my opinion as the cost would be of the order of several times that of a GTL plant. Perhaps that is why Shell sold out there share in Choren who then went bust faster than a chest of drawers falling off a cliff. BTL is otherwise known as burning money.
OK, I did a little digging into the project's history. It's easy to understand why there's confusion about it. Searching on "Pearl GTL", the first few pages of hits that come up are nearly all either pages from Shell's web site, press releases from Shell, or "news articles" that are transparent echoes of Shell press releases. Shell is spinning the releases, pushing the line that "everything is great, the project is a huge success, comining in on schedule and budget ..". Well, yeah, as long as you're talking about the most recent reworked schedule and budget, and not the original ones. The delays and four-fold cost overrun from the originals are being stuffed into a memory hole.
I did find an article that appeared in Qatar's Gulf Times for December, 2007. It gives an interesting account of some of the problems. (Article here). The project apparently got off on the wrong foot (or footing, actually) right from the start. The complex is huge, covering nearly a square mile. But when Shell started construction, they discovered that the site survey was bad. Soil conditions on the survey, on which the foundation plans had all been based, didn't match up to what was actually encountered. The plans had to be completely reworked. Then further into the project, they found that suppliers with whom they had contracted for the thousands of giant valves and other specialty parts were overwhelmed and couldn't deliver on schedule. They had to line up new suppliers in China.
Reading between the lines, it sounds reminiscent of the foul-ups and overruns that plagued the construction of nuclear power plants in the US back in the '70s. Or more recently, the difficulties that companies encountered when they tried to ramp production too quickly in Alberta's tar sands. Logistics headaches, and plans that overtax the infrastructure's ability to deliver. In this case, however, it's possible that corruption was also a factor. Even though Shell is carrying the entire financial risk, their contract with Qatar indirectly gives them a perverse incentive to inflate costs: they pay nothing for the gas until their capital costs have been recouped. There's also the fact that the delays and overruns did scare investment away from would-be competitors, and kill some planned projects that might have gone to other companies.
In any case, Shell is now sitting in the catbird seat for GTL technology. Even with a $19G price tag, the Pearl plant will be highly profitable. Interest on capital computed at 10% adds "only" (how our scales have changed) about $37/barrel to the cost of product. And another article I found (here) indicates that they now planning a new $10G, 2 million bpd facility in Louisiana. Apparently, they're not too worried that the current bargain price of NG won't last. And they apparently prefer GTL over LNG for exporting our newfound abundance of NG.
Robert,
Interesting for both what you are and what you are not saying .)
I suspected and you have tracked/researched gasification from many years ago because it makes the most sense of all options available. I look for your posts specifically. I'm not expecting you to reveal what you are working on in detail but how is it going?
I hoping for a "Mr. Gasification" unit to be at my local hardware store someday.
I hope you have run into this gentleman;
http://www.fundamentalform.com/html/energy_from_waste.htm
Godspeed,
D
I suspected and you have tracked/researched gasification from many years ago because it makes the most sense of all options available.
As I have pointed out many times, gasification is commercially practiced today. And when countries have been cut off from oil -- like Germany during WWII and South Africa during apartheid -- both turned to gasification for their liquid fuel.
Yes, but their does seem to be room for improvements(!).
What I keep stumbling up against is a material that will take both the heat and the organic acids/chemicals without degradation. This Dobson fellow I linked is leaning toward ceramics. But, ash has the insulation qualities, temperature resistance, and self repairs...what to do(?) Dobson's latest work is toward a small CHP unit, which interests me personally, I have no commercial interests. More wood falls and rots annually that I can burn in several years here.
I wish you the best,
D
I think that where Pyrolysis will realize its breakthroughs is not in the upgrading step, but the reaction step. The improvements that Kior has already begun to realize relative to other pyrolysis technologies is that they have formulated a catalyst which produces a much more stable pyrolysis intermediate product with less oxygen entrained. This way the upgrading step is a simplier proposition that can occur in a singe stage instead of multiple stages. I believe that Kior is capable of producing fuel at the 2012 costs in your figure with their demonstration plant and at much lower costs with commercial scale plants - we shall see when their plant is built. Kior is working on fast tracking the implementation of their technology by utilizing existing refinery capital for the upgrading step. If refinery capacity is to be idled due to higher prices and lower demand, it could be put to good use upgrading pyrolysis intermediates.
You need to do your homework on Kior because as far as I am concerned it will soak up money and disappoint. It is nothing but rehashed FCC technology which in the main is not suited to the production of bio based transport fuels which are iincreasingly is becoming middle distillate centric. Feeding it with biomass will introduce a lot of oxygen which is going to cause a whole lot of issues, in the form of organic acids which will rot the hell out of any existing plant.
Think abou the scale up to run an existing unit. A small FCC is 20000 b/d or about 3000 tonnes per day of VGO equivalent. That means to operate the plant efficiently you would need to feed in the equivalent of 3 times that amount of biomass or the reduced volume as pyrolysis oil, which incidentally is acidic as hell. Maybe this is why Kior share price has tanked and they have not gotten past a pilot plant.. This is a carbon rejection process and any subsequent product will require some serious upgrading with a hydrotreater. Sorry but this technology is for the fairies. Yes we shall see- but not any time soon. Bankruptcy beckons.
Likewise BTL suffers from the same issue of vast amounts of biomass and problems with oxygen. FT is expensive even for simple gas projects. Biomass FT will so expensive it will be out of sight. Methanol to gasoline MTG is the same. Nice on paper until you doe the logistics and the size of the plant.. To give you some idea a 1.5 million tonne methanol plant will cost over $1 billion based on gas feed. Add in the cost of biomass logistics and a biomass gasifier and I would reckon you could nearly double the cost. Fairyland again
Solaslime. Another niceon paper short on delivery; great idea I think not. US Navy gets sucked in and blown out.. Suddenly cosmetics are more luctative than fuels. With sugar at $600 pmt and a thermodynamic efficiency of 25% at best I wonder why. Do your homework. Heterotrophic algae also respire which reduces the yield, and optimising the production of TAG comes at a price as well. It is called Entropy.
Then read up on NPP which is mentioned in another post below. NPP is on the decline. Not good if you intend to use biomass as a feedstock-- positively devastating if you are concerned about soil fertility and the environment.
As a person who looks at feedstocks I have yet to see any process that can be competitive with fossil based feedstocks. Much wishful thinking but as ever it is the Law of Receding Horizons. Just anothe hill to climb. blah, blah blah. Oh, and an IPO (I'm pissing off - with your money). Don't believe the hype. Look into the process, examine the thermodynamics and if they are not published then be suspicious.
Remember - there are many thing that can be done with chemistry, not all make sense.
FCC = Fluidized Catalytic Cracking (not Federal Communications Commission). Had to look that one up.
And VGO = Vacuum Gas Oil. Had to look that one up too.
Please be careful tossing around TLAs. Not all readers of TOD are as conversant with them as you may be.
I am not necessarily placing my bets on Kior as a company - they may or may not succeed. My point was to use them as an example of how the technology will incrementally improve over time. Thus, I am placing my bets on the use of catalysts to greatly improve the economics of pyrolysis. Kior's technology is the first step of a long process of improvements that will undoubtably occur with the pyrolysis technology. I believe that Kior is the first company to utilize catalysts in a commercial venture to produce motor vehicle fuels. If you study the literature, catalysis has been documented to improve the quality of the intermediate products of the pyrolysis reaction. This is clearly a large step in the right direction. I also believe, like DOE does, that pyrolysis has the best chance to produce renewable fuels at the lowest cost.
Yes, the intermediates of the pyrolysis technology are crappy stuff, but catalysis can play a role in improving the quality of those intermediate products and significantly lower the overall costs of the technology. I think that a very viable way forward is to place pyrolysis reactors in the field close to the renewable feedstocks, produce a stable intermediate liquid product and then send the intermediate product to a larger, centrally located upgrading facility (i.e., refineries). Conventional pyrolysis technolgy produces an intermediate product which is way too unstable to ship for upgrading at a centralized facility. This is where catalysis can really help the pyrolysis technology. In the end, FCC catalysts and its technology may not be the ultimate solution, but, again, Kior is moving in the right direction with what it is doing.
Good luck on delivering biomass at any meaningful scale for $45/ton.
That's what caught my eye too. Existing examples of plants that use biomass as an input show that the cost of feedstock goes up as demand increases, not down. Also I would be very interested to see how they plan to achieve a 10X reduction in fuel upgrade costs.
Agree. I always tell people to plan on the costs of commercial hay, which is around $100/ton.
And it's a lot higher where demand exceeds supply, since it gets trucked in. Limits the size/location of your plant pretty quickly.
Alice Friedemann posted an excellent piece on the Culture Change web site in 2007, "Peak Soil: Why Cellulosic Ethanol, Biofuels Are Unsustainable And A Threat To America", on the considerable logistical challenges facing cellulosic ethanol, in which she laid out all the problems with supplying a processing plant with feedstock:
http://www.culturechange.org/cms/index.php?option=com_content&task=view&...
I agree that there are significant challenges to biomass, that current U.S. agribusiness practices are highly unsustainable, and that biomass is not and never will be a silver bullet to replace all fossil fuels. That said, I think the linked piece is highly conclusory, and that biomass can be done sustainably and could make an important contribution to future energy needs. The biggest challenges are political, not technical.
It's a serious problem, to be sure, and the hay example is a good one.
Presumably biomass-to-liquid plants would have to be located very near the biomass sources. If the hay baler loads a truck which drops its load right into the hopper at the biomass reactor, you can keep transportation costs down.
The question is, how big an area of agricultural land would a biomass plant serve, and what are the transportation costs within that area?
This problem reminds me of the North Dakota lignite power plants. There's a ton of low-grade coal in North Dakota, but it costs too much to transport it to Eastern US power plants. So they built a bunch of power plants in North Dakota to burn the lignite, and sell the electricity. North Dakota is a huge fossil fuel energy exporter, but the fuel never leaves the state.
One of the theoretical advantages of flash pyrolysis to produce bio-oil is that it lends itself to a tiered logistics chain. The pyrolysis stations are small, cheap, and very local -- potentially even one per farm or small cluster of farms. The bio-oil created can't be left untreated for long, but it is dense liquid that can be efficiently transported to a regional upgrading / refining plant.
Or so the theory goes. I don't know of any places where the model has been implemented as yet. That's not to say that it hasn't been done; I haven't followed developments all that closely.
One interesting point about the upgrade / refining process: I read the DOE report that was referenced in one of the graphs above, and learned that the upgrade path was hydrotreating of the highly oxygenated bio-oil to yield a low oxygen feedstock for subsequent refining. And the hydrogen for the hydrotreating comes from -- drumroll please - natural gas!! Which goes a long way to explaining why the cost per gallon of this approach to biofuels is projected to be so much lower than others. A significant fraction of the energy content in the upgraded fuel is supplied from natural gas!
Not that there's anyting wrong with that. Biomass still provides all of the carbon and the lions share of the energy in the fuel, and the fuel is a lot more compatible with transportation infrastructure than LNG or CNG. But to fully qualify as renewable, the process would need to use hydrogen derived from additional biomass or from electrolysis using renewable electricity. That would raise ist cost somewhat.
Yeah that's a huge problem. When fossil fuels run out we'll all be so desperate for energy the cost for biomass will shoot way up.
The problem with these algae biofuel (and any biofuel) schemes is that they don't take the necessary big step back to look at the macro picture to see how well they could scale to make any meaningful difference to our overall energy predicament as we run out of FF's. I recently wrote a piece on this topic, just the scale of the problem and how totally unfeasible and dangerous a reliance on biofuels going froward will be. Last week I put my link on the Drumbeat but readers here may find it interesting as well.
The planet's total Net Primary Production has gone down, despite modern agricultural technology. The gains we have enjoyed from the Green Revolution have merely (partially) offset the declines in NPP due to ecological degradation. And the thing is, most of the gains from the Green Revolution come from fossil fuel inputs, so when we subtract those out as we run out of FF's, global NPP will drop substantially and then the share of NPP devoted to humanity (25% right now if you include the degradation + the harvest) will shoot up, even just to maintain current food production. If we're going to try to replace fossil fuels with biofuels then we've basically entered an ecological collapse; it can't be done. As Robert says, it's pure fantasy.
http://markbc.net/world-energy-use-and-ecological-productivity-an-order-...
Gregor
I read your posting - thanks. Even better was the Thermodynamics for Economists. Your reference to purveyors of perpetual motion was highly amusing. It saddens me that Economists are held in such high esteem; I wish they would stick to economics. It saddens me even more that some academics are purveying all sorts of bio solutions for nothing less than montary gain and inflated egos.
Much of what NREL has published remains to be tested in scale up and that is where reality will bite. The logistics are frequently ignored and will be the killer. Every step requires and energy input that saps the final net energy available.
A few months back I listedned to a gentelman from Boeing talk of the routes to biojet fule and how it would be soon viable as an alternative to fossil jet fuel. He cited projections from Bloomberg. Let us say that there was an interesting exchange of views and he came off worse. Even better was the BP Biofuels nunce who waxed lyrical about their miscanthus project in Florida. When I pointed out that his yield predictions for ethanol had yet to be achieved in practise he went apoplectic. Even more so when he presented micanthus yields that also have yet to be acheived. All this with no fertilizer requirements and in petuity.
Airbus also presented, and when I pointed out that their yield predictions for Jatropha were about 10 x reality they had to accept that they had made an error, in public. I really wonder about some of these people. Clever they are not.
I seem to recollect that when I first came to this site seven years ago, the shared assumption was that (to paraphrase Kenneth Deffeyes) if oil ever reached $40/barrel then Farmer McDonald would have his day in the sun and sell his biofuels to the Arabs. Well that day seems to have come and gone. In spite of miraculous claims for switchgrass, hemp seed oil, 3rd and 4th gen biofuels, algaes that spit oil, etc. etc. we are no closer to a non-fossil ICE fuel then we were before. Wasn't there a concept bandied about here to explain this phenomena? "The Law of Receding Horizons" perhaps? That liquid fuels built with/upon the current oil-soaked industrial infrastructure would always be more expensive than petroleum and therefore unavailable without specific government subsidy. Oh, then there was the issue of rainforests, and human hunger?
What has changed to allow these schemes to succeed now? Why not when oil was at $80? Or $147? Now that demand destruction is sending the price back down to $90 will that be the magic price point?
Just a general comment about these types of oil drum postings: it would be greatly appreciated by me (and I suspect some others)if a little more interpretation of the slides were offered in the text. I was struck by this post and a previous post about EOR in Saudi fields that much of the post is simply a series of slides with very little help for the reader. Yesterday, I tried to understand carbonate wormholes, water production vs. water injection (how are they different?) on a slide, and so forth. On this post I do not know many of the terms. Yesterday some of the slides themselves were sometimes pretty fuzzy- even when you enlarged them and I could not read data at all. Maybe the oil drum is evolving into more of a technical journal- and if that is the goal I understand completely and won't mention this again- but I don't think it would take too much effort to explain a little. The amount of text in this post is very minimal. Thanks.
by the way- I say all this because I really appreciate the work you guys do and I was interested in Robert's post. I hope you don't take this the wrong way- best wishes.
I am afraid that is because the underlying assumption (however gussied up by pretty graphs) are unreasonable. Take for example claims for algae; there has not been to date a viable algae biofuel project. It remains a minor academic exercise (having failed at NREL several decades ago) full of technical promises for genetic miracles that have never panned out. Corn ethanol has proven a failure as a cost-effective replacement for conventional high-eroei fossil fuels. It mostly remains a fuel additive. You are confused because that is the intention of such academic schemes--to extract subsidies and funding, not energy.
This is precisely the experience the oil & gas industry had with enhanced recovery techniques in the early 1980s. In the event, costs were invariably proportional to the price of oil. While numerous methods - surfactant flooding, caustic flooding, in situ combustion - were proposed and researched, only large scale CO2 injection and steam flooding proved economically viable. I've not heard of "The Law of Receding Horizons", but it's name is self-explanatory. Likely only the best of the biofuel schema will make it.
Possibly the problem was that nobody noticed that Farmer McDonald's major expenditures are on fossil fuel for his tractors and fertilizer (which is basically fossil fuel plus the Haber process), so that as the price of his biofuels went up, the cost to make 'em went up too.
You can play whatever financial games you like, but in the end, money plays second fiddle to energy. If your business model gives you an energy return on energy input that's less than 1, you're going to have a rough time making a profit.
Yes, we need an EROeI analysis, not $/gallon. That metric has led to a lot of false hope. Its like using the waterline distance of the Titanic to determine the best location to be in the future: "The stern of the Titanic is rising! If we all cluster at the back railing it will be fine."
The OP is assuming everyone here understands what each of the technologies he lists is.
Well I for one don't--and there are probably others in the same boat.
Could someone clarify what the technologies are that he is talking about?
Algae - Grow bioengineered pond scum that produces oil. Harvest pond scum, squeeze out vegetable oil. Convert to diesel and/or burn directly.
PBR - Photo Bio Reactor - Grow bioengineered pond scum in big glass tubes. Keeps out wild pond scum that kicks it's ass and eats it's lunch.
Fischer-Tropsch - Make gasoline and diesel out of nearly any carbon source using heat, pressure, and catalysts. Energy intensive, capital intensive, produces lots of CO2.
Methanol to Gasoline - Alternative to F-T process for making gasoline and diesel. Uses natural gas feedstock, developed by Mobile corp.
Pyrolysis - Heat up nearly any organic material, get CO and H2. Use as feedstock for F-T or M2G process to get liquid fuel, or burn directly on site.
Fast Pyrolysis - Stuff the organic material through the hot zone in a couple of seconds, get bio-oil. Bio-oil is a different chemical than regular old crude, and turns to gunk within months. It needs to be processed in order to burn in a standard engine.
All of the above processes have issues that need to be resolved before widespread rollout.
I am concerned that the charge toward these approaches will result in strip mining soil nutrients and minerals to produce biomass. This is something every grower deals with, and if we don't respect the limits we could end up creating large regions with very low biological productivity. Nothing a few hundred thousand years of weathering and wild plants won't cure, but that's not going to do us any good now.
GOWHN said,"I am concerned . . . in strip mining soil nutrients and minerals to produce biomass.
I am not so much. I don't believe we have the will or the crude for such silliness. These various schemes would have happened years ago when crude prices climbed past $40/barrel.
The PDF of the slides of actual gov't policy wonk's presentation list a lot of topics where it is likely the wonk expressed an opinion by his/her tone of voice. We don't have that tone recorded, and don't know which way they might be moving on any particular topic.
A standard policy topic is choose 'the right technology'. I have read this before and have always taken it to mean 'study the problem until the market has decided and issue a backdated study report'. For a few months back in the early days of Obama administration, I thought maybe these people will be different, but now I'm more inclined to believe that there actually are no winners, so it doesn't much matter what they choose.
"I don't believe we have the will or the crude for such silliness"
- Alas we are currently strip mining the soil and the fossil aquifers to grow corn for ethanol, on a large enough scale to do some real damage. And this is being done because our tax money is coerced from us to fund this boondoggle. Perhaps less of these kinds of things will be done as the crude gets scarce enough, but it may get worse before it gets "better" in that sense.
Not anymore!
http://www.nytimes.com/2012/01/07/opinion/one-bad-energy-subsidy-expires...
The major ethanol subsidy was not the cents per gallon subsidy that expired--but rather the continuing mandate:
http://www.heritage.org/research/reports/2012/01/ethanol-subsidies-expir...
No question that corn as grown commercially depends on regular applications of fertilizer. However, the leading contenders for cellulosic biomass tend to be perennials that develop deep root systems. Some, such as miscanthus, thrive on marginal soils and require no irrigation. If cut in winter when nutrients have descended into the roots, they require little or no fertilizer.
Other contenders,such as black locust trees, host nitrogen-fixing bacteria and fungi. They enhance soil fertility.
The main problem with biomass in general is scale. There isn't enough marginal land available to supply more than about a quarter of current liquid fuel needs from biomass -- even under best case scenarios. However, if we electrify most ground transportation, we could produce enough biofuel to operate diesel heavy equipment (road graders, power shovels, etc) and supply jet fuel for a reasonable airline industry.
You are still going to pay $100 per ton to get a farmer to deliver that theoretical perennial cellulosic biomass crop.
For a real perennial deep-rooted biomass crop, it is worth studying the challenges in the wood chip market. I burned several megawatt-hours worth of trees that were cut down last weekend because it would cost more time and effort than I could ever hope to get processing or delivering it anywhere.
Just what process do you propose for jet fuel, because so far the only process has been veg oils, and the cost is not even slightly competitive with normal jet fuels.
I have yet to see any scalable process for jet fuel. Sure there a processes but they are not not even close to being affordable. That means that flying will be for the wealthy. i.e. a much smaller airline industry and no airfreight.
I do not buy into you miscanthus solution. Take away the biomass and the soil fertility will go down and the soil organic carbon will plummet. I don't care how deep rooted they are it will be a monoculture and environmental disaster.
Personally, I'm not a purist when it comes to fossil fuel replacement. If we can dial our petroleum usage down to only the uses for which it's irreplaceable --- by way of example only, suppose that's aircraft fuel, plastics, and pharmaceuticals --- it will last a good long time. It will cost quite a bit, due to loss of economy of scale and the need for extra cracking and synthesis to get the right atomic weights, but I don't see a need to make a plan for 100% replacement when we haven't started on the first 1%.
Don't let the perfect be the enemy of the good.
Perhaps you know more about jet fuel than I do. My understanding is that it's basically kerosene. Like diesel, it can be blended from the fractions that are distilled after cracking the wax from FT synthesis.
If the FT route turns out to be inefficient for biomass, I would think that the hydrotreating of bio-oil from flash pyrolysis would yield fractions that could be blended for a suitable jet fuel. "Suitable", in any case, is a flexible term. If economics require it, then fuel tanks and burners can be redesigned, and flight operations constrained if necessary to accommodate fuel characteristics that are less than ideal. We're talking long term future, not the next ten years.
BTW, It's not my miscanthus solution, and I'm not asking you or anyone else to buy into it. To the extent that I have any favored camp, it would be the "cheap electricity from gen-4 nuclear driving synthesis from CO2 and H2" camp. But mostly, I just want to help people understand the options.
I am not so optimistic. There will come a panic point where these schemes will be pressed into action regardless of the consequences. It will be the "look what I have achieved" with the unsaid "I will be gone by the time the consequences hit". After all how much stewardship of our current resources is taking place? Think that will change when the BAU club starts panicking about where their oil is coming from?
NAOM
I think the BAU club is already starting to panic. "Drill, baby, drill" is not the sentiment of someone who feels secure and confident in their energy supply. Things will only get worse until they fail. The question is where the failure point is; I remember that people argued over the viability of tar sands - well, that one works. Deepwater is old news. Gas-to-liquid may turn out to work in places with lots of natural gas for a short while. After that, it's coal-to-liquids, and then things like shale-to-liquids (which I am pretty certain is way, way too poor to support BAU).
I think we need new chart with the best data for a comparison, as time has shown that some of the old EROEI charts are pretty off. Biofuels also fit on the chart, but depending on the feedstock they vary wildly (sugarcane vs corn for ethanol, for example, with sugarcane being much superior). This article and the accompanying charts are actually pretty confusing, IMHO. Pyrolysis? Of what? Forests? Grasses that are farmed? Corn?
In any case, I think biofuels should be considered non-renewable at industrial scales. The "BAU club" is right now running through all the options as fast as possible, but at some point the game will end and BAU will be over (not just modified, but ended). The questions are "when?" and "what then?", but in any case the endpoint is gradually starting to come into view.
You and Goodol'whn sound about right.
Biofuels is from photosynthesis wherever you can extract oily stuff from the product.
It is difficult and costly enough in both financial and energy terms to get food and paper out of photosynthesis sufficient for the 'advanced' world, let alone for the up comers. There is room only for niche or ‘lifeboat’ technologies for small scale fuel production using ‘wastes’, in my view.
Sounds like an attempt to seriously trash the planet on our way out. Don't underestimate though the capacity of these guys to do just that.
I don't know. We are currently allowing countries to chop down masses of rain forests to plant Palm trees for extracting Palm Oil. When market forces rule, there seems to be no limit to humans willingness to ruin our planet. Biofuels, although they will be vital for the future to provide any form of oil for essential usage, we really need to change our dependence on oil and never for one second think we can replace current fossil oil consumption with "grown oils" instead. To do so will be the sure death of the planet within a very short timespan. Its essential that our remaining "free oil" is used to build the infrastructure changes to reduce globalism and reduce energy usage per person on the planet as well as a major downscaling of the human population to a more sustainable one.
As long as this goal is not on the agenda, but a world where growth and current energy consumption is king, humans will attempt at seriously killing off the planet until it slashes back at us with force (most likely in the form of resource conflicts before we recognize climate changes - but most likely a mix of these).
To be a bit more optimistic about it, we can hope the bad effects of our transition into bio fuels will be so visible to the public that we decide that we have to think differently about energy consumption.
Wonky TOD citation
"A bit energy wonky but interesting: The Oil Drum has a breakdown of a recent Department of Energy presentation on current cost of various renewable fuels and how inexpensive DoE thinks those analyzed might get over the next five years."
http://www.treehugger.com/renewable-energy/how-cheap-will-algae-biofuel-...
wow!!!
A total misreading of what is going on in the outside world.
This is an example of why I love TOD and respect RR and his postings.
Thanks RR and TOD!
Here's a new one: Peak phosphorous
It's not so new. It's been talked about here for years.
The really scary thing is that organic sources are depleting as well. Phosphorus is normally tied up in top soil and forests, but much has washed to the sea with erosion and over-harvesting of the world's forests. The natural systems, anadromous fish and their predators, that used to return this precious mineral back to the land from the ocean are all but extinct world-wide. Very sad.
Peak people? Peak politicians?
The assumption seems to be that hydrocarbon fuel should be an ambient liquid. If you consider natural gas as an internal combustion fuel it comes way out ahead for low cost.
Working in megajoules of thermal energy we have a litre of petro-diesel with 35 MJ. A US gallon is 3.8 litres so a gallon of standard alternative fuel needs 133 MJ energy content. Equating an mmbtu with a gigajoule or 1000 MJ the low gas price of $2 is 0.2c per MJ. Therefore natgas with equivalent thermal energy to a gallon of diesel has a wholesale cost of 133 X 0.2c = 27c.
Forget biofuel and use natgas as a petroleum replacement. Generate baseload electricity with nukes not gas fired plant. With pyrolysis I'd also like to see estimates of
1) what fraction of 90 mbpd we can expect to make
2) the amount of fuel used by the harvesting machines
3) what happens after drought, wildfire and expensive NPK nutrients.
Unfortunately there is just not that much natural gas in the U.S.--even though there are temporary surpluses now.
"If half of this supply becomes a reserve (225 Tcf), the U.S. has approximately 11.5 years of potential future gas supply at present consumption rates. When proved reserves of 273 Tcf are included, there is an additional 11.5 years of supply for a total of almost 23 years. It is worth noting that proved reserves include proved undeveloped reserves which may or may not be produced depending on economics, so even 23 years of supply is tenuous."
http://www.theoildrum.com/node/8914
Well, gasoline only barely qualifies as "ambient liquid". But the existing distribution system is designed to deal with liquids, and it would fairly expensive to replace with another form.
Even if you were prepared to rebuild every car and gas station in the world, all the specific alternatives have major drawbacks when you go beyond megajoules per dollar:
Compressed natural gas: bulky (low energy density, MJ/liter)
Liquefied natural gas (methane): need cryogenic storage
Liquefied petroleum gas (propane, butane): not enough available
To paraphrase Churchill, liquid hydrocarbon fuel is the worst form of energy for vehicles, except for all the others that have been tried.
DME is fine at ambient temperatures, easily transported and stored and classified as non-toxic.
It can be burnt in a diesel engine with only minor modifications.
China is rapidly increasing production.
Methanol is also relatively easy to handle, although corrosive and toxic, and can be used directly in fuel cells.
Hydrogen can be stored in CF tanks and transported mixed in with the NG in the same pipelines as is to be done in Hawaii, then separated out at point of use, at least initially until it is checked out whether it is going to be used enough to make building a network worthwhile.
Hyundai, Toyota, Mercedes and just about every other manufacturer is to start producing hydrogen fuel cell vehicles by 2015, to follow up on their already established use in fork lift trucks.
At 72mpge for the small Hyundai SUV thanks to the higher efficiencies of fuel cells compared to combustion engines losses from converting NG to hydrogen are cancelled out, and you have a far more effective energy medium.
There are very substantial challenges in moving to other fuels, but it is hardly hopeless.
Also commuting can be done with electric vehicles, perhaps if the economy does not allow it much more modest ones than the typical car today, but nevertheless something with enough wheels and a roof to get around in.
The insured cost of a 22 kwh battery is &7,500, around $548 kwh, so something like the Twizy with a 4kwh battery would certainly get people around at reasonable cost, even if it is far from the SUV they would prefer.
'Other fuels' can certainly keep the heavier traffic which can't run on batteries about, and whilst biomass IMO is not going to cover mass transport, it can run agricultural equipment on the farms where it could be produced locally.
As a one-time algae grower, IMHO everything in algae's favour as a fuel source is against it:
Its too expensive to use artificial light to make it grow so you use sunlight in vast ponds. But you cannot make them deeper than a metre or you get anaerobic growth in he bottom that consumes a lot of your energy and is basically dead water depth you have paid for. So the area requirement is huge for any sensible output. Lots of capex, and lots of ongoing maintenance of pipes, auto valves, pumps by the dozen etc. Big electricity bills.
If the algae concentration gets too high it block slight penetration and inhibits growth, so you have to balance density with depth.
Algae requires nutrients - not just sugar but dear old NPK in the right ratios - growth is limited by the essential nutrient in short supply. Train loads of such nutrients will be required every day to get tanker loads of product out.
The water plus maybe 1% algae is a very diffuse thing to deal with - the pumping and handling costs involved in concentrating that to recover useful biomass with minimum water content will be enormous, let alone the costs in the final algae to oil stages.
Evaporation from these ponds will see salinity climb to Mars. Dilute with more water to control salinity and you send nutrients to waste. What water from where?
Algae likes lots of sun. Sunny places are (or soon will be)deserts. Dust drop in the ponds can mess with nutrient balance and mess with growth of the algae you want. Being open to the sky any and all sorts of algae will inhabit the ponds, and temperature swings will create inversions and blooms of all sorts of undesirable organisms - I've seen ponds turn from green to red in an hour. Outa control!
Next!
Great comments from someone who's actually been there. This is one of those renewable energy things that looks great on paper until you talk to someone on the ground.
This is probably the best point of a good post. The billion-dollar question for biomass energy seems to be the energy cost of material handling. In another thread, folks were discussing the price of moving bales of hay around. Here it's even worse: you have to pump your algae around in circles constantly while it grows, and you have to pump 99 times its mass in useless water as well.
Imagine if the coal industry ran this way. We fill up coal trains with a mixture of 1 part coal to 99 parts waste rock, then drive them around the country in a big loop, continuously, until the power plant they're passing by happens to need some coal.
Algae requires nutrients - not just sugar but dear old NPK in the right ratios...
A bit limited on time, but wanted to comment on this. I never cease to be amazed by the number of people who claim "The great thing about algae is that it requires no fertilizer." Yeah, right.
The water plus maybe 1% algae is a very diffuse thing to deal with...
The way I sometimes explain it to people is to presume you have a lot of soggy, shredded newspapers in a lake. There's your fuel. You just have to harvest it, dry it out, and use it. People would immediately understand that this is not much of a fuel source.
Evaporation from these ponds will see salinity climb to Mars.
I have queried Sapphire Energy about exactly how they plan to deal with this in their algae farms in the desert:
http://www.smartplanet.com/blog/intelligent-energy/sapphire-energy-raise...
I have never gotten an answer.
Yet another issue for algae that is seldom mentioned, but sometimes acknowledged in cryptic circumlocutions:
Algae requires CO2 dissolved in water at concentrations higher than is normally there in Nature. CO2 is the first nutrient of autotrophic life, before N, P, or K. That's the reason for locating algae farms near to coal fired power plants. OTOH, maybe sunlight is the first nutrient. But at some point it becomes pointless to add further items to nigwil's list.
One reason for false belief that NPK are not needed is that in badly designed experiments, adding extra NPK does not improve NPP. The real reason for the result is that CO2 is the limiting resource in the experimental arrangement.
Here's my opinion on algae farming. There are a huge number of technical challenges you have to deal with if you want to grow algae on land:
* Maximizing the amount of chlorophyll exposed to sunlight
* Managing water delivery and controlling evaporation
* Managing nutrient levels
* Maximizing gas exchange (while minimizing water loss)
* Defending against harmful external organisms
* Concentrating and dessicating the produced biomass
Fortunately, there's a machine out there that solves all these problems, and lets you grow algae anywhere you want, effortlessly. It looks like this:
http://upload.wikimedia.org/wikipedia/commons/thumb/6/67/TallWildGrass.j...
The best part is, manufacturing costs for this system are zero. It literally self-replicates!
Grass contains a similar level of mineral ash as coal, about 7% by weight. When you burn it, nitrogen and phosphorus are burned away while potassium is retained in the ash. I don't know which of the secondary minerals are retained. Also the ash is in the form of oxides, which are not as bio-available as the same minerals recycled by decomposition. So even if you distribute the ash back onto the fields, using grass for fuel is not a closed cycle. It does help if the grass is harvested in winter when the plant naturally stores nutrients in the roots but you still lose some.
The other main issue is the fact that ashes do not support the microbial population that depends on decaying vegetation. Does it matter? Probably not on any one plot of land. But on a wide scale, we don't really know. We do know that fertile soil needs a microbial population. But we don't even know what types of bugs are in there, or what they do.
I have a suspicion that it does matter. But it's been hard slogging trying to track down a definitive answer. If anyone has good references about what happens to nutrients in vegetation when it is burned, I would appreciate it.
Burned, pyrolized, or digested? Each is different. An anaerobic digester will leave essentially 100% of nutrients in the outflow liquid. Slow, low temperature pyrolysis I believe leaves most nutrients other than nitrogen in the char. (Not really sure on what happens to the P, but I think it's left in the char.) Flash pyrolysis leaves very little; most everything goes into the bio-oil. But it can, in principle, be recovered from the upgrading process.
It isn't actually necessary to return 100% of harvested nutrients to the soil. There's a natural rate at which atmospheric nitrogen is fixed by microflora in healthy soils. Also a natural rate at which inorganic soil components (mostly sand and clays) are dissolved to replenish P, K, S, and other trace elements. That's what deep root systems and symbiotic fungi are good for.
Yes indeed. At this point I believe a digester is the only truly closed loop method of making biofuel. Bummer that it take big tanks and produces gaseous fuel.
Burning the biomass directly is far simpler and produces more heat. But if it depletes the soil then it's no solution at all. Still researching that point.
My post wasn't meant to suggest that growing grass is trivial, but that whatever the problems, they're worse with algae.
Yes, land plants need phosphorus, but so do algae. Yes, land plants have to worry about bioavailability of nutrients, but so do algae.
The difference is, land plants grow in an environment full of mineral micronutrients and symbiotic organisms which transform rock minerals into forms the plant can use. With algae farming, anything that's not algae or water is a potentially dangerous contaminant, and all macro- and micronutrients must be supplied and monitored by the grower.
Thanks to hundreds of millions of years of evolution, and thousands of years of human experience, most land plants can be grown almost on autopilot. If you want to grow algae outside its natural habitat, you're on your own.
Overall, I agree about the dubious viability of biofuels derived from algae. But I think that citing the nutrient requirements of algae as evidence of non-viability is a bum rap.
Sure, algae require NPK and trace nutrients like any other living organism, but the elements don't magically vanish into the aether; they're still present in the residue of harvested algae after the oily liquids have been extracted. The point is that those elements are not incorporated into the oily liquids; the latter are pure C-H-O molecules. All that's needed to keep the system going is recycle the residue from extraction back into the pond water, and supply CO2 and sunlight.
That's after you've managed to engineer a strain that is highly productive for oil, yet robust enough to out-compete wild strains that will inevitably get into the pond. Or maybe one that is resistant to an herbicide that will kill off the wild strains.
Anyone like to estimate how long it will take genes to jump?
NAOM
Goodolwhn
Decades ago our University needed to stop us putting P32 in the waste for the incinerator. It concentrated in the ash and gave the furnace man an illegal dose of radiation.
I just turned up the following: could be useful even though it applies to sewage sludge, (which is mostly biomass?). The NOx burn off but a lot of mineral is left behind, including it seems the phosphates.
http://www.aidic.it/cet/10/21/127.pdf
And another one here:
http://www2.lwr.kth.se/forskningsprojekt/Polishproject/JPS11p19.pdf
Btw - 'miscanthus' Elephant Grass leaves most of its soil nutrients behind in the perennial rhizome mat while the vegetation dries out in the winter. This does make the crop more useful for biomass except you still need to use fairly reasonable arable ground for the crop. In this booklet (link below) the crop yield is quoted as "coal equivalent" of about 4 tonne "coal" per 10 tonne of dried crop, or something under 6 tonne "coal" average per hectare. Harvestable dry-stem yields reach a plateau after 3-5 years, usually reaching from 12 to 16 t/ha (around 5.6 t/acre). Here in UK we have possibly about 6M hectares maximum of cultivable arable land - this works out about 10 of our persons per hectare. In energy terms if we used all the ground we might get 35Mt of 'coal equivalent’. For comparison, for example, in 2006 the UK imported ~50Mt of coal for power generation. And miscanthus is about as good as it gets.
http://www.naturalengland.org.uk/Images/miscanthus-guide_tcm6-4263.pdf
Excellent! Thanks for the links, I will read them all.
Re Sewage sludge ash.
One of the linked papers that include incinerated sewage sludge ash shows low potassium content in SSA; (Utilization of Incineration Waste Ash Residues in Portland Cement Clinker, Charles Hoi King Lam et al, 2010, Table 2). I assume that prior sewage water treatment removes most of the relatively very soluble potassium.
A discussion of historical removal of soil nutrients from soils (export), with some numbers, is in 'On the Great Plains': Geoff Cunfer, 2005, partly available in google preview. You can see Table 8.2 page 215 for removal of N by crops including various hay crops. American pioneer farming essentially mined soil nutrients until yields dropped. Obviously potassium and phosphate and other mineral are also removed by cropping. The figures that I have for P & K are for grains themselves (not straw or hay), but without doubt removal of hay crops off-farm and not returning minerals via manure or NPK fertilizer is an extreme form of nutrient 'strip mining'!
Forgive me RR if you have covered this before.
Do you have an opinion or assessment of any electricity-to-fuel techniques? If off-peak electricity is free, water electrolysis is cheap and so are pure oxygen and hydrogen.
These people think they'll solve Germany's transportation and winter fuel problems with renewable methane:
http://www.solar-fuel.net/
And these guys think they have cracked the "gasification" nut for synthetic fuels by avoiding the solid phase altogether:
http://www.dotyenergy.com/
Any chance they're right? You're the expert!
I should also have mentioned the Canadian methanol people : http://www.bluefuelenergy.com/index.html :)
X
I heard something similar from Audi last week at ASPO 12. The Audi chap said his process was 54% thermodynmaic efficient to the synthtic NG step which is wrong. We had an interesting exchange. The reality is much less, and will be similar for methanol. Yet more energy is expended in the DME step and a whole bunch more in the gasoline suynthesis. Every step increases entropy and reduces the final yield. There is no free lunch.
In simple terms it does not make sense. I am short of time at present but I will try and make some numbers up, to give you an idea if you are interested.
Solar fuel net link is busted.
I saw that it was down too, but it's good again for me now. The page mentions Audi, you may actually have spoken to some of the people responsible at the ASPO conference.
The chemistry behind this is the Sabatier reaction which is nothing new. Several good sources here
http://www.digipac.ca/chemical/mtom/contents/chapter3/sabatier2.htm
www.co2club.it/agenda/Presentations/S4FE%202009%20TOSTI.pdf
rsta.royalsocietypublishing.org/content/368/1923/3343.full.pdf if this link does not work type synthetic methane synthesis form CO2 into google and look for Turning CO2 into fuel.
This is the same process that Nasa is looking at to reduce CO2 to methane on Mars. In theory it should work fine and the reaction is slightly exothermic. But life is never that simple and a large amount of reltively pure CO2 will be required and the yield on methane is best when hydorgeon is in excess. The reaction is equilbrium controlled and is a variant of steam methane reforming run in reverse. By varying the hydrogen ratio it is possible to form a syngas mixture ( H2 + CO) which can be used for methanol sunthesis and in theory, at least, other hydrocarbons.
This is not the perfect perpetual motion machine, far from it. The production of hydrogen essentially provides ALL of the energy conversion step, though as stated the methane formation step is slightly exothermic. Remember the first law of thermodynamics - energy cannot be created or destroyed. Aslo bear in mind the second law of thermodynamics - entropy will tend to a maximum. This means that the sum of the energy inputs, electricity, will be greater than the sum of the energy in the output, in this case the combustion energy of the methane.
The real isuue is the CO2 source. This needs to be high purity to avoid catalyst poisoning and ideally not contain large quantities of diluents which would otherwise increase the reactor size and consume energy. Flue gas might be possible but contaminants are likley to be an issue. Extracting Co2 form the atmosphere might sound a good idea but would be extremely costly.I would see this as a niche application. Scale will be important and I doubt if the plant can be stop-started effectively to match excess electrical demand to methane synthesis. Some form of hydrogen storage would be required in my opinion to maintain the plant operating continuously.
For the thermodynamics of the electrolysis of water try here.
http://hyperphysics.phy-astr.gsu.edu/hbase/thermo/electrol.html
the sum of the energy inputs, electricity, will be greater than the sum of the energy in the output
In other words, this step isn't 100% efficient, which we certainly would expect. Any estimate of the efficiency?
Extracting Co2 form the atmosphere might sound a good idea but would be extremely costly.
Any guess as to the cost?
Some form of hydrogen storage would be required in my opinion to maintain the plant operating continuously.
How about underground?
I wonder about nuclear navy ships synthesizing fuel with surplus power?
The methanation efficiency is reported to be very high as high as 99% and is limited only by the limiting reactant. As there is an exotherm it would imply that energy is liberated as heat and not products of the methanation reaction. For more description see here
http://www.pennenergy.com/index/power/display/0723256773/articles/pennen...
In the above example the CO2 source is flue gas from natural gas combustion. Other fuels are likely to introduce catalyst contaminants. Nickel and Ruthenium are likley to be poisoned by S, N and other metals if present. Coal would likely to be very difficult.
As in many processes the higher the concentration of material to be extracted then the easier and less energy intensive it becomes. Flue gas would have a CO2 concentration of around 15% but air CO2 would be more like 400 ppmm. To remove 1 Kg of CO2 from air would mean processing something like 2500 cubic metres of air. This would mean a very large air scrubber with a lot of ciruclating fluid extractant and a very large vessel. The energy cost would be significant to drive the fan and the extraction system. That is why a concentrated sopurce of CO2 is required.
Storing the hydrogen would not be easy.Undergrounfd storage would be a challenge due to the Houdini properties of hydrogen. It is so small it will find any leak and will be gone. The only real solution would be above gorund storage in purpose designed vessels and these will cost money and require compression facilities. This type of process is never as easy as it seems.
The energy cost would be significant to drive the fan and the extraction system. That is why a concentrated sopurce of CO2 is required.
1st, that may not follow. Such a system may be able to tolerate low efficiencies - remember, we may be getting our power from a very cheap source of surplus. We really need to quantify this, not dismiss it as too low.
2nd, air probably isn't the best source of environmentally occurring carbon - seawater is about 7.5% CO2.
Undergrounfd storage would be a challenge due to the Houdini properties of hydrogen.
It's done right now:
"Underground hydrogen storage is the practice of hydrogen storage in underground caverns[1], salt domes and depleted oil/gas fields. Large quantities of gaseous hydrogen have been stored in underground caverns by ICI for many years without any difficulties[2]. The storage of large quantities of hydrogen underground in solution-mined salt domes[3], aquifers[4] or excavated rock caverns or mines can function as grid energy storage which is essential for the hydrogen economy[5]. By using a turboexpander the electricity needs for compressed storage on 200 bar amounts to 2.1% of the energy content[6]."
http://en.wikipedia.org/wiki/Underground_hydrogen_storage
Not sure if I think that the power will be so cheap at all. If it is cheap then that will not providean adequate return on investment and pricess will go up Nuclear power was supposed to be so cheap that it need not be metered. Reality it never happened. The shear scale of the extraction process for capturing CO2 from air would be such that it would consume vast amounts of energy, one way or another.
To give a blanced view try these two articles:
www.netl.doe.gov/publications/proceedings/01/carbon.../7b1.pdf
http://www.newscientist.com/article/mg21428593.800-stripping-co2-from-ai...
I am not so sure I agree with the claims of the former, but it is an interesting approach. They tactfully decline to go into detail on the adsorber section, and i not not surprised.I think this will be the weak link.
For your seawater option I am a bit confused. Were there to be 7.5% CO2 in sea water we would not be on the same pl;ant. The seawater pH wopuld be much lower and the CO2 would be gassing off unless the air pressure was sunstantially greater. In solution the CO2 will be in equilibrium as CO2/ HCO3-/CO2= with bicarbonate making up the greatest proportion Typical lvevels are in the sub 1000 pmm range. 7.5% CO2 would imply 75000 ppm
cdiac.ornl.gov/ftp/cdiac74/chapter2.pdf
www.gemarsh.com/...content/.../SEAWATER%20pH%20&..
I used to work for ICI and at the location where the hydoirgen is stored. However this aproach is not widespread and works only because of the seal qualities of slat. It would probably work in anhydrite as well. Both exhibit platic flow under pressure. Any old cavern or mine will not do, so deplted oil filds would also be an option. This limits the pparoach to sedimentary rocks with perfect seals.
Here is a link from yopur Wikipedia link that gives some detail
http://www.ika.rwth-aachen.de/r2h/index.php/Large_Hydrogen_Underground_S...
I wasn't clear about the 7.5% - it refers to the percentage of dissolved gases. Here's the reference:
The abundance of CO2 makes seawater an attractive alternative fuel source. Scientists at the U.S. Naval Research Laboratory stated that, "although the gas forms only a small proportion of air – around 0.04 per cent – ocean water contains about 140 times that concentration".
http://www.newscientist.com/article/dn17632-how-to-turn-seawater-into-je...
The Marsh article link appears to be broken. Am I right to infer from his site that he's a climate change denier?
Thanks for pointing me to the underground H2 storage reference. It indicates that underground H2 storage is very, very feasible:
"An important point to note regarding the performance of the caprock structure is the mechanism involved in sealing the top of the underground reservoir, either a depleted field or an aquifer. This sealing occurs because of water capillary action, in which water fills all the voids of the caprock structure and must be expelled by sufficiently high pressure to overcome the capillary resistances (the threshold pressure of the caprock). Below this incremental pressure, the caprock will act as an effective barrier to the passage of any gas. This incremental or threshold pressure and the effectiveness of the sealing action is independent of the nature of the gas because it is a water-rock capillary effect. This is an important observation in that it indicates that hydrogen in underground storage will behave much like natural gas insofar as integrity against leakage is concerned. " emphasis added
The DOE article link is broken.
Excuse the typos. I am the world's worst typist
Here is another link on the CO2 concentration in aqueous systems , including sea water. I wish the USN luck because they will be processing a whole lot of water and they will have to reduce the pH to spring the CO2. This is how dealkyliser units reduce the alkylinaty of water. H ion exchange resins remove metals cations and replace with H ions which send the pH down. The CO@ is then blown out in a scrubber.
www.geochem-model.org/Publications/41-MC_98_131.pdf
Here are the other links again. They should work.
www.netl.doe.gov/publications/proceedings/01/carbon.../7b1.pdf
http://www.gemarsh.com/wp-content/uploads/SEAWATER%20pH%20&%20ANTHRO%20C...
No idea about Marsh's views.If he is a climate change denier then it is up to him. He is obviously hot under the collar about some conclusions from the Royal Society about seawater pH but the paper does not identify him as a climate change denier. That is at least my view.
I happen to think that the evidence thus far suggests a problem with CO2 emissions. I also do not believe that mankingd has the nous to do anything about it. But that is my opinion, and others on TOD may think otherwise.
Whether underground storage becomes widespread is open to debate. In some places where the geography permits yes, but universally probably not. Think of Italy. Lots of basement rock. Texas might be a different story.
I wish the USN luck because they will be processing a whole lot of water
It would be nice to quantify the costs involved, just to an order of magnitude.
Marsh's views....is obviously hot under the collar about some conclusions from the Royal Society about seawater pH
The problem: if one has a strong emotional drive to discredit climate change, then it can distort one's arguments quite badly. Life is short, and I don't have the time to read stuff from people who think evolution is a UN plot. Seawater pH is a good example: it's a central part of the climate change argument, so an anti-CC advocate is going to go to great lengths to push flaky arguments, which I don't have time to decipher.
I also do not believe that mankingd has the nous to do anything about it.
Do you mean "onus"? That suggests a moral responsibility. I'm not worried about that - I just don't want homes in Florida to disappear beneath the waves.
Think of Italy. Lots of basement rock. Texas might be a different story.
Well, if a few places don't have the geography, that's a problem, but Europe overall is storing several months of natural gas consumption right now.
I too would be keenly interested in any thoughts Robert might have on SolarFuels or WindFuels.
I note that one of the major hurdles for these approaches seems to have recently been overcome. That's the invention of efficient, low cost catalysts for water splitting. They don't involve noble metals. That opens the door for electrolysis units that can be mass produced cheaply; cheaply enough so that operation at a 40% duty cycle won't add unduly to the cost of produced hydrogen and oxygen. No such units are being made yet (AFAIK), but there no longer seems to be any compelling reason why the couldn't be.
The other big issue for these approaches is the cost of electricity. How many MWh per year are likely to be available at various price points? If synthesis requires $30/MWh to compete with oil at $80 / bbl, will it be available for sufficient hours per year for electrolysis to be viable?
I'm inclinded to doubt it. But I haven't found any model for predicting what the availability might actually be.
Synthetic fuel seems like a much better idea.
Let's say wind provided 50% of the kWhs needed by the grid, and wind was overbuilt by 50% to reduce the frequency of lulls. That would create surplus power to the tune of about 25% of demand. That might be sold to synthetic fuel producers for, say, 2 cents per kWh.
Diesel has about 40 kWhs, so at 40% efficiency the energy input would cost about $2. The hardware might get 50% utilization if we're using surplus power, and that might raise capital costs to around $1 per gallon. Operating costs might get us in the range of $3.50 per gallon, which seems pretty feasible. Not quite competitive right now, but awfully useful in the long range.
And, of course, if there's a lot of surplus power you might get it for $.01 per kWh. Actually, this demand source would probably set the price for surplus power.
All of the tech needed for this exists right now, even if it's not quite competitive with the current moderate price of "rock oil" (petr-oleum).
In some markets spot electricity prices become very strongly negative when there's a surplus. Excellent and very hopeful analysis here:
http://www.dotyenergy.com/Economics/Econ_Stabilizing_RenewableGrid.htm
I'm puzzled by the claims of Doty Wind Fuels that their fuels are carbon neutral since the CO2 sources they nominate are fossil based. A bit like a vegetarian wearing leather shoes 'I didn't kill the cow it was already dead'. I'd describe the German approach as 'otherwise curtailed' windpower not 'peak'. Unlike RR's data here the various wind fuel people seem reluctant to give detailed costs. Until then wind fuel should be regarded not as pie in the sky but petrol in the sky.
I have experimented with making grass charcoal by a Filipino method but it's messy. I've also created pure CO2 by burning wood charcoal in oxygen from water electrolysis. That could arguably be described as a sustainable source of CO2 provided all the energy inputs were non-fossil. As of now I don't see any easy or cheap petroleum replacement that can continue forever.
I'm sure I wrote "off peak" wind power, which is more or less the same as "otherwise curtailed" :)
Doty address your carbon neutrality objection in FAQs 5 and 6 here : http://www.dotyenergy.com/FAQs/Faqs.htm
They take the position that buying CO2 that would otherwise be vented is "morally" equivalent to capturing that same CO2 from the atmosphere after it has already been vented, but they're hardly married to the idea of buying it from fossil fuel combustion sources.
They intend to source reasonably pure CO2 wherever it's cheapest, just like soda drinks factories do. In the short term this may be from breweries or fuel ethanol refineries and therefore the carbon is recently diverted from the biological carbon cycle, but biogas and natural gas upgrading facilities are another likely source and if the electric synthetic fuel business ever really takes off, CO2-capturing power plants or cement factories may be the only low-cost point sources at sufficient scale.
Capturing CO2 from the atmosphere is feasible and should not be prohibitively expensive (the German solar fuel electricity-to-gas pilot plant does direct atmospheric CO2 capture), and would satisfy any objection to calling the process "carbon neutral" but honestly : a significant portion of atmospheric CO2 is fossil carbon too, until such time as nobody is burning any fossil fuels any more. No-one indignantly points out that corn or sugarcane crops grown for energy have taken up some atmospheric carbon dioxide originating from recently burned coal and petroleum. In what wise is it worse to obtain the feedstock at lower cost from a fossil source that would otherwise release it to the atmosphere?
IMHO a comparison of the biofuel approaches should be made with projects like synthesis of methan with PV or wind generated electricity etc., here first tests are running and my gut feeling as chemist is that the PV/wind fuel will be cheaper :-)
This analysis was made by Tad Patzek.
Go to all that trouble (low EROI or worse) and then you burn the stuff?
Homo sapiens dufus: In the twenty-first century they knew better but they still burned rocks to move things around. When they ran out of rocks, they extracted all the water from the aquifers and exhausted all the nutrients in the soil. Food scarCities ... no Cities.
a few thoughts:
1) that wind power density looks wrong: turbine spacing might dictate 80 acres per turbine, but the turbine only "consumes" perhaps 1/2 acre, including access roads. So, wind density should be higher than solar. Of course, if PV is placed on roofs, it really doesn't consume any land at all...
2) EVs mostly eliminate braking losses with regenerative braking, so aerodynamics become the most important factor (that's why Prius MPG is higher in the city), followed by rolling and drivetrain friction.
So, once you move to EVs (or hybrids, PHEVs or EREVs) weight is much less important...
3) vehicle efficiency is a slippery concept. From a physics point of view, ground vehicles have zero efficiency, because they simply "translate" from one place to another, which doesn't yield a real output of energy conversion or energy value. So, as we reduce energy losses (braking, aerodynamics and rolling & drivetrain friction), we can reduce vehicle energy consumption ad infinitum.
This illustrates perfectly what I have been telling people. All transport needs to be replaced with a system based on small light weight EVs moving automatically in isolated transport lanes where the power is drawn outside the EV. It has to be a closed system (call it tube-mail system ala Futurama) so that you can strip away almost all weight added by rigid cage/shell that would have been required for safety. Also because you would need a system where a pedestrian or animal is not able to get into and get killed. Furthermore it does not require a big battery (less pollution as well) since power is drawn from a grid outside on the "rails" system, perhaps solar and wind based.
One possibility would be to build them elevated although that could be costly to maintain, another would be to use existing roads but close them in such a way that people or animals can not get into the system. This would be simpler to service in case of a breakdown. Although in an elevated rail the transportation device could more easily have its own set of solar panels on either side to get additional energy, and they could be built where there are roads now for coexisting in a transition period, or for using the roads only drivable by heavier transport trucks. An elevated rail will probably require much less service than the tons and tons of asphalt we today pave roads with over and over again.
The way we create EVs now is for them to coexist on normal road and traffic and is extremely inefficient due to the things weighing 1.5 tons from batteries and a crash safe cage/body. For the future we absolutely have to think differently and we can easily reduce energy used by transportation down to 1/10th probably more.
JohnCL
Exactly. You're hired!
10X is indeed the goal, as I presented in 2007 in Stockholm [pdf, 1.2MB] in a double-hitter with Tad Patzek. Since 2007, we have designed such a system under contract with the City of Uppsala, Sweden. Stay tuned for further developments.
How does fast Pyrolysis compare with Cool Planet process ?
http://www.coolplanetbiofuels.com/technologies.html
See also: Cool Planet Rocks the Bells : Biofuels Digest
Good article, their approach seems to be well above the crowd as they try to adress the whole problem of biofuel production. Sounds like a winner but let's see what follows