An Idea for Inexpensive Electricity: Concentrated Solar Power 12 Miles above the Earth
Posted by Gail the Actuary on September 2, 2011 - 9:59pm
This is a guest post by Keith Henson. Keith can be reached at hkeithhenson at gmail dot com.
A small number of volunteer engineers and scientists are working on what they think will be a low cost approach to solar energy.
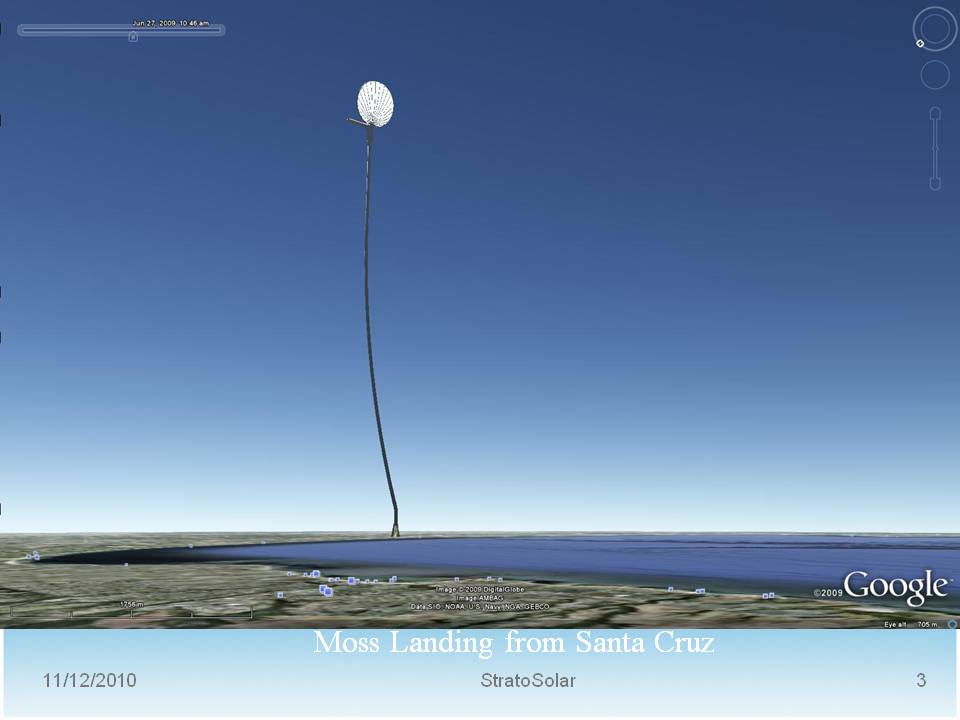
A few months ago, I wrote about Space Solar Power. The idea put forth in that post was to put solar power satellites into orbit around the earth, and beam the electricity back to the earth, using microwaves.
The concept in this article is closer to home. Sunlight for electricity production would be concentrated at 20,000 meters (12.4 miles), and come down to the surface in a hollow light pipe tethered to the ground. The light would be converted to heat at the ground, and used to drive combined cycle turbines to make electricity. They can be located almost anywhere, even places with lots of clouds. The volunteer research is being done at a company called StratoSolar.
While most of this post is about concentrated solar power (CSP) systems, the idea of using solar PV panels at 20 km is also being considered. A section at the end is devoted to the solar PV panel approach.
Why use a tethered approach, 12 miles up?
The stratosphere is a good place to collect solar energy. It is above 95% of the earth's atmosphere so there is much less of the atmosphere to absorb sunlight. There is no dust so the surfaces don't need cleaning. The winds are usually calm and there are no clouds, making the collection of solar power almost completely predictable.
The plants can be placed near loads, reducing transmission cost and losses. The solar flux models indicate this approach will work as far north as Stockholm, because of the greater solar intensity at that height. They occupy very little ground space compared to ordinary solar. The design calls for the collector surface to be pointed at the sun, so there is no cosine loss, a loss that affects ground-based solar power.
If collecting solar energy in the stratosphere works, it scales to human energy needs. It would take only 15,000 one-GW plants to replace all current sources of energy.
Based on a small multiple of the cost of common materials, electricity from the tethered CSP plants could be as low as 1.5 cents per kWh when mature.
On the down side, it will take an enormous amount of engineering and testing to determine if it will work. In addition, the power plants are a potential hazard for aircraft.
How Tethered Concentrated Solar Power Would Work
The tethered CSP design consists of a reflector made of many small (50-meter) elements arranged as an off-axis parabolic reflector. Look for one of those DISH TV antennas in your neighborhood and scale it up in your mind by a factor of 4000 in diameter, 16 million in area. Such a dish at 20 km would collect about 4 GW of sunlight from the time the sun comes up over the eastern horizon to when it goes down over the western horizon. The light curve is practically square.
The individual segments of reflective plastic would have computer-controlled rapid movement with a range of perhaps 2 degrees. The whole dish structure is kept pointed at the sun within a degree by a combination of aerodynamic vanes, fans on the scale of large wind turbines, and reaction (not torsion) on the light pipe.
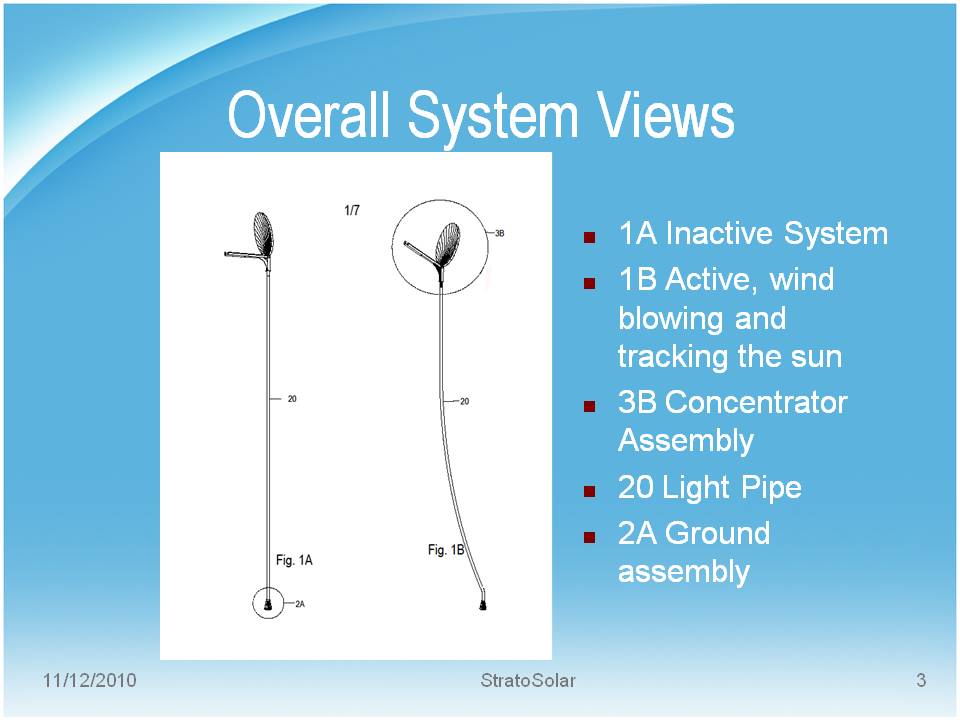
The concentrated light from the primary collector bounces off a secondary mirror and into a hollow light pipe that conducts it to the ground (see Figure 2). The calculated attenuation is around 7% for a 20-km by 50-meter light pipe lined with highly reflective plastic film similar to 3M™ 2301 Optical Lighting Film. 3M thinks they can make the film to 99.5% reflectance and, with considerable research, to 99.7% or better. The angular divergence of radiation from the sun is about 0.28 deg. This increases by approximately the square root of the concentration factor and means the light will reflect about once every 20 diameters (with no bending).
At the ground, concentrated sunlight (~6 MW per square meter) heats air to 1400 deg C at 20 bar, half of which runs an ordinary 60% efficient combined cycle power plant. The other half is directed into a buried 70,000-cubic-meter chamber half filled with firebrick (or other heat storage media). At night heated air is drawn from the heat store and used to power the turbines until dawn.
The firebrick heat store is known as a "Cowper stove." They have been used to preheat air going into blast furnaces for the past 150 years and are well understood. A cheap firebrick at 1400 K has about the same energy stored in it as an expensive lithium ion battery of the same size. For a GW scale base load, it costs around 1/10th of a cent per kWh to store heat for 24-hour power production.
As noted in the introduction, the buoyancy needed to keep the collector at 20,000 meters would be provided by metalized plastic bags filled with hydrogen inside the structure.
So the three elements in the system consist of a concentrator held up by buoyancy with optics to focus sunlight into a 20-km light pipe and a relatively conventional combined-cycle power plant on the ground.
Problems
There are serious engineering problems in designing such a power plant. The buoyancy gas must be hydrogen in metalized plastic bags. There just isn't enough helium available and it is far too expensive. We think separating the hydrogen from air by a meter of nitrogen will cope with the problem of hydrogen fires. Even a rocket fired through the structure should not ignite the hydrogen.
The light pipe is exposed to the worst of weather and wind. Lightning and electrical discharge is a concern but with more than a square foot of steel cable to conduct it to ground, it should not be a serious one. Ozone and UV at high elevations will be problems that require careful materials selection. Hail has not been a problem on the large number of plastic stadium roofs that have been in service for the last 40 years, so may not be a serious problem in this application.
Icing is a concern, mostly because of thick buildups falling off the light pipe. (The concentrator is above where icing occurs.) In addition, there is the man-made hazard of aircraft flying into them. A large aircraft flying into a light pipe would be a disaster for both the aircraft and the power plant.
Wind: the biggest engineering problem
The optimization that led to a ~1 GW size is partly due to the volume of the buoyant support structures. The volume (and lift) goes up by the cube of the linear size while the area (and therefore wind resistance) goes up as the square of the size. Additionally, the light pipe losses go up as the reflectance raised to a power proportional to the length over the diameter.
Depending on the reflectance, the hollow light pipe optimizes at 30-50 meters. That size, and the resultant maximum wind drag for a cylinder, is just too high to be practical. A rotating aerodynamic shroud around the light pipe was required to reduce the wind drag into a manageable range (the shroud cuts wind drag by a factor of 10).
The shrouds turned out to be heavy, but large enough in volume that it was practical to offset their own weight and the weight of the light pipe and hold down cables with buoyancy cells. This prevents the exponential growth in cable size seen in space elevator designs when supported only from the top.
Even with aerodynamic shrouds, the models involve huge forces, 30-50 million Newtons of wind force, and four times that much excess buoyancy to keep the light pipe from bending over more than 15 degrees in a high wind. Made from inexpensive steel wire, the hold-down cables have a cross-section area similar to that of a medium-sized suspension bridge (upwards of 16 inches in diameter).
Every couple of years there is a high-wind event in the stratosphere that reaches 50 m/s (180 km/hr) for as much as a week. The 2-km diameter collector at the top simply can't take the stress of that high a wind, so provisions have to be made to fold it into an aerodynamic shape, and the power plant needs to burn gas or oil for that time if it is to be as reliable as a conventional power plant.
Cost
A quick and dirty metric for base-load power is to take the income for ten years (~80,000 hours) times the revenue per kWh to be the allowable capital investment per kW of capacity. One cent per kWh would allow $800/kW and two cents would allow $1600/kW or $1.6 billion per GW.
The estimated cost of the CSP based system based on design plans come in at about $1.2 B per GW based on a moderate multiple of materials cost (the rough breakdown is $400 M for the power plant, $50 M for the heat store, $500 M for the light pipe and $250 M for the collector. The materials in the proposed design—aluminum, steel and plastic—are produced in huge volumes. There is considerable uncertainty in construction and lifetime issues, but if these are tractable, $1.2 B/GW capital cost would set the minimum price (no profit) for electric power at about 1.5 cents per kWh.
Ongoing maintenance costs are not yet known with any degree of certainty. An initial estimate might be 0.1 cents per kWh. The power generated would be base load, so there would perhaps be fewer maintenance problems than combined cycle. It is not clear yet how the maintenance of the concentrator and upper light pipe would be handled. If it is done by robots, the cost of the robots would be one of the maintenance costs. If it is done by humans, they would need to wear space suits.
Why should this kind of solar power come in at something like 1/6th of the lowest-estimated cost for other forms of solar energy? The 60% efficiency of combined cycle thermal plants accounts for a factor of four compared to 15% efficient solar cells, assuming similar cost per square meter. The rest of the lower cost comes from the increased solar energy due to being above over 90% of the atmosphere, being above the clouds, and not having the cosine effect of ground solar power. Moreover, 24-hour power is simple using low cost high-temperature thermal storage.
Energy Payback Time
Hydrogen is expensive in terms of energy, about 50 MWh per ton. A tethered CSP plant would take a lot of hydrogen for buoyancy, around 5,000 tons. It also leaks hydrogen, around 1-3 percent per year. To fill one up would take 250,000 MWh or 250 GWh.
That's about 10.5 days for a one GW plant. The total energy investment for plastic, aluminum and steel wire, and a combined-cycle power plant is around 5 times as much as the hydrogen so the energy payback is about two months, about a tenth of the energy payback time for PV cells in a desert.
After a year and a half of trying to find showstoppers, we feel confident that there are solutions to many of the problems identified. There is a lot of detailed engineering to do and there are problems we have identified but not solved—particularly how to construct the light pipe and the huge rotating aerodynamic shrouds. If anyone has ideas about how to construct objects of this size without wind destroying a partially completed section, please post your thoughts.
Photovoltaic variation
There is very little (almost no) experience with large tethered stratosphere structures. It is not surprising that people are reluctant to venture into this area for objects on the scale and cost of GW power plants, as required by CSP.
Can we start smaller? Not with the thermal type because the light pipe can't be made any smaller than a few tens of meters. If the pipe is a few meters, all the light is lost in the reflections.
Understanding that it isn't optimal, is it possible to put PV into the stratosphere? The power could come down at 20-30 kV in aluminum conductors inside the tethers.
There are enough advantages to consider this approach, particularly in Northern Europe and Japan. If the cost per square meter for cells supported in the stratosphere is close to the cost of a ground solar there is some, but not a not a lot of advantage, for putting PV solar collectors the in the stratosphere instead of a desert.It's a different story in places where there is persistent heavy cloud cover such as Germany and Japan. There the advantage over PV on the ground is a substantial multiple because of the high and predictable light levels.
The minimum size would be a lot smaller, in the few tens of MW.
More detail regarding these proposals is available from the StratoSolar website.
Note: The author has no financial interest in StratoSolar. His involvement with the company has been in the role of a volunteer engineer.
Keith, have you ever considered going into writing science fiction? I think you've done it again with this bit of fantasy.
Off the top of my head, I would first ask how much your 2 km reflector would weigh and how large would the balloon need to be to support it and the light pipe dangling below at an altitude of 12 km. Ever seen a picture of the launch of a high altitude research balloon? They start our with a rather small diameter at the surface, but at higher elevations, they expand greatly. I recall that at an elevation of around 5 km, one is half way thru the atmosphere and at 10 km, one is above 3/4 of the atmosphere, so your balloon would need to expand perhaps 5 times the size at the surface. Go ahead, draw a balloon on your picture and let us see what it would look like. Don't forget to add the wind loads on the balloon to the loads on the 2 km reflector and the light pipe tether.
Have fun...
E. Swanson
"Keith, have you ever considered going into writing science fiction?"
Actually I have. One story, "The Clinic Seed" is being turned into a movie script on request from a movie producer even though it has only been published on the web.
But this idea *isn't mine." It belongs to Ed Kelly who is best known as one of the main people in Transmeta.
Re your complaints, the collector is 200 meters thick. There is plenty of room inside for enough buoyancy to keep it floating at 20 km, where the air density is around 6% of sea level. Remember that 5000 tons of hydrogen generates 13 times that much lift at any altitude.
Wind drag, as discussed in the article is the biggest problem, it sets the excess buoyancy at four times the maximum wind force to keep the lean angle under 15 deg.
If you want to see the spread sheets analyzing the physics, ask, especially if you will check them for errors.
An excellent response. Few in the blogosphere appreciate the proper vetting of ideas and concepts which includes using those opposed to the idea to test and help improve it. Keep up the good work Keith.
The problem is not in the physics. You need to assume that this has been thought through. The problem, as with most similar ideas, is in the economics. Here are just a few items that I can see that makes the idea as it stands unviable:
- The proposal identifies the conversion to electricity via a "conventional" combined cycle power plant. Well it will not be conventional. In a conventional gas turbine the power plant uses relatively low cost burners to heat the compressed working fluid up to around 1500 degC before it passes into the turbine. How is this to be achieved in the proposal? It must be through a heat exchanger. This exchanger has to be very large in order to achieve the required levels of heat input. All operating at high pressure and at the very limits of allowable material temperatures. That alone will add significantly to the cost of the "conventional" combined cycle plant.
- It is proposed to include a substantial heat storage device so that the plant can be run around the clock. I have seen a number of similar proposals relating to "on the ground" solar thermal plants. They don't work. The reason they don't work, is that in order to get the thermal efficiency (in this case 60%)out of the plant you need to have your MINIMUM heat input at 1500 deg C. So the heat storage device will need to cycle between 2000???? deg C and 1500. Again material problems. The delta between high and low operation temperatures is inversely proportional to the size of the storage medium/container. So if you try to squeeze the delta T to acceptable material limits then the volume/cost blows out to unacceptable levels.
- Then there is the costs. It appears that the "conventional" combined cycle power plant forms a relatively small component of the overall cost of building and maintaining the proposed power plant. A really conventional combined cycle power plant costs (for only capex payback and maintenance, no fuel) around 4.0 c/kWHr to run. So how can the proposed plant generate power at 1.5 c/kWHr. This portion is well established technology so you are not going to get any technology or scale gains in this area.
I don't want to be completely negative as there are element of this idea that may make sense, such as the elevation of a PV array and transmission by HVDC to ground. But the proponents need to do more work on the economics rather than just concentrating on the physics.
"It is proposed to include a substantial heat storage device"
This is 150 year old technology, the same systems are used to preheat air for blast furnaces.
As you load and unload the heat from one, the hot to cold transition level moves up and down. The output temperature stays almost unchanged till the cold front has moved up from the bottom to near the top.
Can you point me to a location which shows capital expense and maintenance for a combined cycle plant costing 4 cents per kWh?
Keith
Sorry I should have explained in more detail. Of course heat storage devices work. The problem is that the device you are proposing works on the basis of specific heat ie. the the heat stored and liberated is a function of the specific heat and mass of the medium and the average delta T of the medium before and after recovery. As I am sure you are aware that combined cycle efficiency is a direct function of the GT firing temperature. Therefore in order to claim the efficiencies nominated the minimum temperature of the exhaust gas from the storage must be at around 1500 degC or above depending on the arrangements you make for heat transfer into the GT working fluid. As you say you can utilise a "once through" heat store to reduce the maximum temperature of the device. But the size of the device needs to be very large to accommodate the required heat storage ie. 12 hours x 1 GW x 1/0.6 = 72,000 GJ with an average delta T of only 750degC. Then by opting for a once through system you need to consider how you inject the heat into the storage device. The entire device needs to be heated to 1500 degC without any waste heat rejection. How do you do this ??? If you transfer the heat into the medium through a fluid then by necessity there will be substantial waste heat rejected. If you transfer the heat into the medium through direct light then you will need to figure a way to move/handle the medium at a temperature of 1500 degC.
With respect to the costings, I build these plants for a living and so am fairly familiar with the financial drivers involved. The EPC construction cost of a conventional combined cycle plant in Australia is now around $1500/kW. It will be a little lower, say $1400 in the US due to lower costs of labour. The construction finance and development cost will push this up to around $1600/kW for a US plant. The mimimum return on Capex that investors are prepared to accept is 15%. This covers depreciation, interest and profit. In the case of your proposal this number would be much higher to cover the risk. Working on 15% for a standard plant operating on base load at 8000 hours per year this translates to 2.9c/kWHr. Then you need to add the operations and maintenance. Depending on the type of plant and the operating regieme this will be between 0.5 and 1.0 c/kWHr.
Why do you need a once-through fluid loop to charge the heat storage? Recirculating it allows the remnant heat to be reclaimed. Also, the heat transfer to the incoming fluid is a function of the ΔT between it and the storage material. If this is assumed to be linear and the heat reservoir is initially at a constant temperature, the ΔT will fall exponentially as the fluid moves through it. What you'll get is a "wave" of temperature change moving through the reservoir, with the input side heating/cooling first and most rapidly. The exhaust side will change last, and most slowly.
"12 hours x 1 GW x 1/0.6 = 72,000 GJ with an average delta T of only 750degC."
I assumed 50% so for 1 GW out, the storage is 12 GWh. But I also took the delta T as 1000 deg.
For delta T of 1000 deg K (high end temp 1400 deg C) and a specific heat of 1kJ/kg per degree, it is a thousand kJ/kg or a million J/kg or a billion J/tonne. So the mass needed would be 86,400 tonnes of fire brick.
I also assumed 20 bar air as the working fluid into the turbines. The air would be heated by incoming light through a transparent window that will hold the pressure. The entire heat store, some 70,000 cubic meters half full of fire brick, will be under 20 bar pressure.
During the day, half the hot air produced will run the turbines, the other half will be sent down through the heat store. (Fan at the bottom returning 400 deg air to the top.)
At night the compressor output will go to the bottom of the heat store. Even with intercooling, 20 bar air is going to be hot. The exhaust air from the turbines will go through a heat recovery boiler and to atmosphere. Recompressing would allow using argon or CO2, but this looked to be lower in capital cost.
A while ago I worked out the levelized capital cost of 1600/kW SBSP. It varied from $15.3/MWh to $27.8/MWh depending on the discount rate with the lower being at 5% and the higher being at 15%. So 2.9 cents per kWh is about right for 15%.
Your numbers for O&M seem to be rather divergent to this http://en.wikipedia.org/wiki/Cost_of_electricity_by_source
O&M (mostly fuel I think) is about 3 times capital cost in their table. Using these numbers, 6.6 cents per kWh and discounting 3/4 for free heat, we get around 1.7 cents per kWh.
If StratoSolar costs a lot more than Ed original estimates, that's ok by me. Might make SBSP the lower cost option.
This is really interesting. I want to share my own idea about how this might work even though I'm not an engineer. If this comes out making no sense I apologize in advance.
I pictured something with continues airflow like this.

Light would come in through a window (or perhaps two windows with a vacuum between them for insulation), and heat up some rocks or fire bricks(painted black). From there the heat would be distributed throughout the system by a continues air flow. The heat would be used to make electricity by heating a boiler. The temperature of the boiler would be controlled by directing the air flow with the 'air control flap' or some similar device. Everything after the boiler functions like a traditional power plant. This whole system would have to be insulated somehow to minimize energy loss.
A few suggestions:
Ammonia is a bit toxic, but at least you know about it. Breathing nitrogen is just plain lethal. Worth considering.
It's *really* hard to get the light pipe size down. But the idea of using open cycle gas turbines at altitude is worth looking into as an alternative to PV. The engines on 777 aircraft are only a tenth of a kg/kW, and thermal engines are likely to be a substantial multiple over PV.
Not sure regeneration makes sense given the mass they would involve, but it's worth looking into it.
Thanks.
The CAES system and its regenerative heat-storage would be groundside. Compress air, run it through an insulated bed of gravel or something to capture the heat of compression, and pipe it into a big reservoir somewhere (salt domes, high-permeability aquifers on anticlines and certain rock mines seem to be popular in the proposals). On the discharge cycle, recover heat from the gravel before piping air through the turbogenerator. This may also be suitable for the gas-fired backup power system, using the same subsystems without the air storage (and possibly alternating regenerators to recover and recycle turbine waste heat).
Using regeneration to improve the OCGT efficiency at altitude is an interesting idea. Weight may be an issue (though you no longer need the excess lift to offset the drag of the ligth pipe), but given the size of the whole affair bulk would probably not be.
This is beginning to sound an awful lot like Glaser's original concept, isn't it? (Figures. My L5 Society member number was in the 1400's.)
I agree that H2 has a large buoyancy ratio. The trouble is, the mass of air per unit volume is quite small at the surface and is much less so at 20 km altitude. The problem is that your buoyancy is the result of displacing air with a less dense material, thus it is the volume of displaced air which is important, as the density of H2 is small. A cubic foot of air at MSL pressure weighs about 0.11 pounds, so a cubic foot of H2 would provide lift of approximately 0.10 pounds. At 20 km altitude, the amount of air in a cubic foot is going to be your 6% of that at msl and the lift from H2, (which would also be at reduced pressure), would be only 6% of 0.10, or roughly 0.006 pounds. Thus, to provide the lift for a ton (2000 pounds) of mass, the required volume would be roughly 333,000 cubic feet of gas bags.
Since you haven't bothered to present an estimate of the total mass aloft for the system, we are left to guess as to the size of the volume of the gas bags required. What's the total thickness is that steel cable gonna be again, 16 inches? Until you provide a mass analysis, you are just playing silly games with meaningless numbers. Hand waving and "don't worry, it'll be OK" is not allowed, as we are facing a crisis...
E. Swanson
My email is at the top of the article. Send a request and I will send you a spread sheets where the forces and masses are analyzed.
I don't remember if the spread sheets are on the web site. Maybe Ed Kelly could chime in.
If you want to help, glad to have you.
The author of the article can attach files to it, which anyone can download. (hint, hint, Gail.)
I noticed that you posted a spreadsheet further down. I don't understand what your buoyancy calculation refers to, perhaps because it's late and time for sleep.
Are you assuming the light pipe is an open tube filled with hydrogen under some pressure above atmospheric? Since the tube is anchored to he ground, the column of H2 would not directly lift the tube, instead the column of H2 would bear directly on the base of the tube. The pressure of the H2 gas acts perpendicular to the axis of the tube, with no vertical component except at the top of the tube. Where are the lifting gas bags to be located, at the top in the structure for the collectors?
E. Swanson
The spreadsheet is a bit hard to grasp because I didn't intend it for external use. But no, the light pipe does not contain hydrogen, that's all in the rotating shrouds with the excess lift in the top 5 km. The shrouds get up to 200 m wide and several times that long. I have no idea of how to construct them.
NAOM
The problem is damage from a local wind like kicked up by a thunderstorm on a partly completed shroud.
No problem if you build them inside, but then we are talking about really huge building.
Open to ideas.
Inflatables, with the inner annulus for the light pipe held open by fibers extending to the outer skin. Build them flat indoors, and the "assembly" is done in hours during windows of clear weather. Lift with hydrogen, balance pressure with ballonets.
"Inflatables,"
It's possible that might work.
Perhaps you should solve the lift problem before continuing further. Consider an air foil, such as the NACA series. They are defined with a thickness ratio, that is, the ratio between the maxium thickness and chord length. A thick NACA airfoil, for low speed use, has a thickness ratio of perhaps 25%, Thus the length is 4 times the thickness. Your 200 meter wide shrouds would need to be 800 meters (or more) in length. As you note, building such a structure would be difficult, to say the least.
Think of the size of the Graf Zeppelin, which was built in 1927 and was 236.6 meters long. The Graf Zeppelin had a gas volume of 3,707,550 ft^3 and thus had a total lifting capability of about 371,000 pounds at MSL. At your 20 km elevation, that volume could only provide a lift force of 22,250 pounds.
Perhaps you could give us an estimate of the displacement and mass of each shroud and the gross and net lift available at altitudes between 15 to 20 km...
EDIT 1: Added lift calculation
EDIT 2: Oh, BTW, the Drag Coefficient you used, 0.05, may too small, especially at higher altitudes where the air pressure is small and thus separation can occur more easily. Did you include Reynolds Number in your calculation?
E. Swanson
"Perhaps you could give us an estimate of the displacement and mass of each shroud and the gross and net lift available at altitudes between 15 to 20 km..."
Sure.
https://docs.google.com/spreadsheet/ccc?key=0ApiotdmmTJQsdDV2VkpfTlJlSS1...
The last ten 500 meter segments each take 30,000 newtons of the excess buoyancy. They are close to 4 times longer than they are thick. The figure in column X is the percentage of internal volume taken up by hydrogen to get 30,000 newtons of lift after subtracting the weight of the shroud structure.
At 200 meters, they are almost as thick as the Graf Zeppelin was long.
And yes, Reynolds number was part of the determination for the drag coefficient. 0.05 is slightly conservative and wind velocity is low in that altitude range.
Analyzing them is fairly simple, but, as you mention, building them will be a daunting task.
Keith, your spread sheet shows a nearly constant lift force for each segment of the shroud over the last 10 segments between 1.5. and 20 km altitude altitude (also a near constant but lesser value is given between 0.5. and 15 km). You apparently haven't grasped the fact that the lift force is a function of density and you show the density dropping from 0.181 to 0.089 kg/m^3 between 15.5 and 20 km altitude. For a shroud of fixed size the gross lift must decline and the net lift after subtracting the weight of the shroud must decline even faster. Also, the low drag coefficient only applies when there is near zero angle of attack, which means that the shrouds must quickly turn to follow changes in the wind direction, which would be expected to cause difficulties with the very large shrouds you propose. Another problem with the low drag coefficient is that under icing conditions, the surface of the shrouds would not be smooth, which has often resulted in crashes in aircraft as the result of loss of lift at lower altitudes...
E. Swanson
"nearly constant lift force for each segment"
Below 15 km, the lift was just enough to compensate for the steel cable and the mass of the light pipe plus shrouds. Above 15 km, a lot of extra lift provides the excess buoyancy.
The size of the shrouds more than doubles from 15-20 km (volume increases by 8) and the amount of internal volume being devoted to lift gas goes way up. The amount of steel wire being held up goes down too as the force declines from 300 million newtons to zero. It's all accounted for in the spreadsheet. (At lease I hope so. If you find something in error please call my attention to it.)
Wind direction changes tends to change slowly at this altitude. The shroud segments freely rotate to stay pointed into the wind (assuming a bearing doesn't stick).
Icing isn't a problem this high up. Lower down it is. There are well known ways to crack the ice off, but I don't know how well they will work.
What I did was definitely limited to first order analysis.
If the maximum width of the shroud has to be that much bigger than the light pipe, it looks like the problem is in the specs of the light pipe.
The light pipe should probably be made with an FRP skin lined with reflector. It should be possible to make such construction self-lifting if filled with hydrogen at a slight overpressure, and the overpressure gives it structural rigidity. If the light pipe doesn't need external lift, the shroud can be built to a close fit instead of being oversized for lift.
Also, active airflow control may cut the required size and weight (at the cost of complexity, but many parts could fail without increasing drag to unacceptable levels so the redundancy would be very large).
Lets get down on Earth again and stop dreaming, shall we?
If you have a better idea to make solar energy effective in places with a lot of clouds, please don't keep it to yourself.
It might help if you understand I started as a skeptic on this concept. If you can show a physics based reason it won't work, please do.
I tried my best and failed to find one.
http://en.wikipedia.org/wiki/Solar_energy
And if one looks at the black dots here:
http://en.wikipedia.org/wiki/File:Solar_land_area.png
That covers primary energy used by Humans.
Why is this idea better than ground based collectors? It seems to me there is no reason to worry about clouds. You put solar collectors/plants in deserts. You put up high voltage transmission lines, which can go thousands of kilometers with minimal losses. This is all old, proven technology.
I see no reason why people are racking their brains for local solutions. It_Is_Not_Necessary.
"Necessary" is an interesting word. Its syntactic form, and the way we use it, have it an absolute quality -- like, say, mass or color. We may debate about whether something is or is not necessary, but the framing is that it's got to be one or the other, and we're just trying to resolve which.
In reality there is always a relative context: necessary to / for what? Nothing is ever necessary in an absolute sense; the survival of the human race isn't necessary to the rest of life on the planet, and survival of the planet isn't necessary to the univers. So what's the relative context for saying that an energy technology -- one believed by its proponents to offer a 3:1 or better advantage over ground-based alternatives in EROI -- is not "necessary"?
There are plenty of folks around who firmly believe that the arrival of Peak Oil will spell the collapse of industrial civilization and massive die-off. Their argument boils down to an asertion that the very high EROI payback of oil is what has enabled growth to the current state, and that the energy and capital investment needed for switching to alternatives is too high for feasibility. They assert that we simply won't be able to make the transition before declining EROI triggers a collapse. I think they're wrong, but I can't be entirely certain.
Given that, isn't an approach that plausibly claimes a 3:1 advantage in EROI over other RE alternitives interesting, prima facia? Isn't it worth at least some serious evaluation to see whether its proponents might actually be on to something?
The idea under discussion fulfills the requirement of being down to earth. "Discussion about energy and our future" certainly does not appear to be a topic limited to fanciful economic speculation or dieoff scenarios.
I would also add that this is a good thing.
I think you are understating. Unless you are thinking about bypassing the 7-12 km Jetstream band, you are looking at constant 100mph gusts. The ones that flow on top of Mt Everest. I love crazy ideas but I don't think a tethered cable would work at all, the wind would rip apart any cable you put. A floating device in space would be a better bet.
"bypassing the 7-12 km Jetstream band"
No, the maximum wind forces on the light pipe from zero to 20 km are all accounted for. Big as the aerodynamic shrouds get, they are teardrop shaped and generated *relatively* little wind resistance. Normally the stratosphere is calm, but there are these rare events every couple of years. When one of them comes along the only solution I know of for this huge concentrator is to fold it into an aerodynamic shape. I have a model on my desk if you would like to see it.
But I do agree with you re space based solar power, it's a *lot* easier to analyze, though perhaps a hundred times as expensive to set up the transport system as to build a test StratoSolar plant.
But all Passenger Jets use these streams heavily, mostly to reduce drag and improve fuel efficiency. How is it that Jet Streams affect commercial airlines but won't affect your contraption. Are you making this assumption because of the cross sectional width of the cable ?
You can put a youtube video of your desk model for all to see :)
"How is it that Jet Streams affect commercial airlines but won't affect your contraption."
They do. For the light pipe design, the jet stream at ~11 km is the major source of the force on the light pipe.
Here is the chart we incorporated into our models. http://www.centennialofflight.gov/essay/Theories_of_Flight/atmosphere/TH...
Wind peaks at 90 m/s at 11 km. Force on the pipe is proportional to area x wind speed^2 x air density x the drag factor (about .05 for streamlined shapes).
Compute in blocks, say 100-500 feet and sum up to get the total. That's conservative because wind seldom blows the same way at all levels.
It's a hell of a lot of force, ~40 million Newtons, ~9 million pounds or 4,500 tons of force.
The upward force (excess buoyancy) has to be 4 times this much for the forces resolve to 15 deg.
This takes more than a square foot of steel wire to hold it down.
The largest aircraft engine,
http://en.wikipedia.org/wiki/General_Electric_GE90
can produce ~500kN of thrust. Reportedly it costs something like ~$25M. If you took some number of them (5), that would be enough thrust to move the top of the collector assembly around at some rate, depending on the wind speed and direction. With sufficient hydrogen flotation and water ballast the collector assembly could be “flown” at any altitude.
You could assemble the entire collector assembly on the surface some where, in a circular bowl-like structure, sort of like a stadium but flatter and with tie downs and inflatable roof to hold it until the right moment. If you waited for a period of calm, you could launch it and have it rise and clear the walls of the assembly building in less than a minute. You could carry sufficient cabling to tie it down, or to reach sufficient cabling on the ground at the final site. You could attach sufficient cabling to hold it down in less than an hour. Then you let it rise to operational altitude bringing the cable up with it.
Your engines are attached to a lifting body that flies back to the assembly building to take the next collector assembly to the next site. On the way back there is a lot less drag, but also no lift from the H2 flotation.
Another possibility is taking the collector down. That would also solve maintence. It may complicate the tube, but you should be able to put it up and take it down anyway, maybe doing both once generate the same number of problems than doing both each couple of years.
"Another possibility is taking the collector down"
This was considered early in the design work. Adding buoyant shrouds complicated the task of taking down the light pipe to the point we gave up on it.
This idea is so heavy, it's going to go down like a Led Zeppelin ....
Sorry 'bout that
The famous Rock band, Led Zeppelin, chose their name because they were going to "go down like a lead balloon", or as the US phrase goes, "go over like a lead balloon" - also, the name was originally spelled Lead Zeppelin, but everyone mispronounced it so they took out the "a" in Lead. That prediction was false.
I hope Harry's is too.
Interesting idea, keep up the good work.
How about a free floating version that produces synthetic fuels from the electric? Once in a while a plane load of synthetic fuel is brought down and a plane load of water and CO2 is brought up.
That's an idea I've thought about, and still rather like. Though more as a kind of "poor man's space station" than a pure solar power platform. There are a couple of issues.
"Bringing up a plane load" of whatever is not as simple as it sounds. Although there are commercial jets that can reach the stratosphere, they can't fly there at low speed. In the thin air at that altitude, they're on the edge of stalling at mach 0.7. Not exactly suitable for rendezvous with an airship.
To rendezvous, you need an aircraft that can go into a vertical climb and get into a ballistic trajectory that peaks precisely at the airship's "capture port". It sounds dicey, but with differential GPS and a programmed autopilot, it's not technically challenging. Most fighter planes could manage it if the right control software were installed. But for commercial operation, you're really talking about a new custom-designed type of cargo aircraft. New aircraft of that sort would require tens of billions to develop and certify. Unless you can score an end run around the FAA and get Scaled Composites to build you one for a few tens of millions.
The other big issue is scale. If your airship is tapping a gigawatt of solar power, you're talking about a lot more than an ocassional plane load of water and CO2 up and fuel down. It's a steady stream of 737-sized cargo planes coming arriving and leaving every few minutes. A power tether begins to look pretty attractive, in comparison.
For the bring things up side how about a rope? Or rather a 20km long steel cable? Lift cargo up with two helicopters with payload in between grab with grapple at end of cable reel up. Works for the down side also.
You are right GW is 600boe an hour!
Why lift tanks? Pump stuff up inside hoses. Carbon dioxide could even go up as a gas, inside the light pipe (it would need to be heated if lift was required).
Ed was asking about the idea of a free floating platform, using solar energy onboard to make fuel. It wouldn't have a light pipe.
I suppose a free floating stratospheric platform could dangle a big hose into the troposphere, equipped with a blower on the end to push moisture-laden air up to the platform. It would arrive as an ice fog, but it would be pretty easy to extract the ice particles and supply all the water the electrolysis units would need. If the platform made ammonia rather than hydrocarbon fuels, it wouldn't need a source for CO2.
That might actually be a nice way to gain some experience with stratospheric platforms and prove out the concept of stratospheric solar power, without having to deal with tethers and power delivery. There's certainly a large enough world market for ammonia.
What weight per square meter are you using for the PV? Are you using concentrators with 40% efficient solar cells?
Most of the post is about CSP. The PV version was mentioned at the end, but not really described. I can imagine the PV version would be interesting to move out of the way, if it suddenly got very windy.
The 10MWp reference PV design is conservative. The lightweight PV panels are 1.5kg/m2 and 11% efficient.
The greatest engineering challenge is the light pipe. So....get rid of it. That's my initial reaction.
Have you guys considered placing the powerplant in the sky? Sandia National Labs is working on a super-critical CO2 Braydon cycle turbine (link below). They claim that it's ~50% efficient (at fairly low temperatures) and 30 times as power dense as traditional turbines. They cite an example of a 20 megawatt turbine occupying a space of four cubic meters.
https://share.sandia.gov/news/resources/news_releases/brayton-cycle-turb...
The genset could be composed of superconducting magnets which are also very power dense. Just doing some back of the envelope calculations here but I reckon a 1Gw genset would occupy 30 cubic meters and half of that would be steel so you need to lift 259,050lbs of steel into the stratosphere. Air is about 13% as dense up there so 1.21kg x 0.13= .157kg/m^3. Hydrogen gas is 0.08925kg/m^3 at stp so .0116kg/m^3 at altitude. You'd need ~1.78 million m^3 of hydrogen at altitude to float your power plant.
Assuming insolation of 1200w/m^2 you'd need ~833,000m^2 of collector area which (assuming spheres) would take up ~13.52 million m^3.
Someone is going to have to check my numbers. I'm pretty tired and doing this on my phone in bed but it seems like you're in the ballpark. If you can deliver power for less than $0.04kw/h you're beating coal. Flying a heliostat at altitude doesn't seem like it should be that big of a deal for a first step.
Whoa, my numbers are WAY off. Will correct in the morning. Again, it seems like such an idea is "in the ball park". I don't see any problems with (relatively) thin cables passing through the more hostile parts of our atmosphere.
I'm a fan of supercritical CO2 cycles, but in this case I think the weight of the radiator system to dissipate the heat from CO2 at 8 MPa to air at 0.1 MPa probably favors an open-cycle gas turbine. We do have a great deal of experience with open-cycle gas turbines operating at 50,000 feet.
You may be right, but which would work out better is an open question. Strictly speaking, I have to note that it wouldn't be a "radiator" -- it would be a gas-to-gas heat exchanger. On the plus side for closed CO2, the atmosphere in the stratosphere is really cold (-50 C), and it's relative thinness is not a significant disadvantage. Thin gas = long MFP = high rate of thermal diffusion.
I've worked with Ed and Keith on this stuff. There are two main reasons that Keith favors the concentrating solar thermal cum light pipe option over the solar PV approach. One is the higher conversion efficiency, and the other is the capability for 24-hour power generation via the ground-based thermal heat store. The chief negative is that the light pipe makes the minimum feasible size very large. That 30 - 50 meter diameter is necessary to limit the number of light bounces and achieve a good transmission efficiency. Doing the power generation at altitude eliminates the light pipe and allows a much smaller system to be built, at the cost of giving up the 24-hour power generation capability. IMO, that's a good tradeoff, since there are other ways to deal with the 24-hour issue. I suspect the truth is that Keith simply likes big projects -- though of course I'd never say that to his face. Would I? (Just kidding, Keith.)
IMO, if the electricity is cheap enough, storage becomes less of an issue. At $0.01/kw/h we can afford to pump air underground and lose 30% of the energy.
I really like EP's idea of using open cycle gas turbines. Efficient? No but that doesn't really matter when using an infinite (for human purposes) source of energy such as the sun.
It's also a way to bootstrap to the size which light pipes require without having to make it in one leap.
@Engineer-Poet
Great point! I didn't even think of that! Turbines could be located at the bottom of the heliostats to keep it's center of gravity as low as possible and thus provide some stability for tracking the sun.
IMO, this seems very feasible. All the tech, save for the tether/transmission line, is off the shelf.
Joke aside- this is stealing bandwidth.
Here is some more funny future guessworks from French painter Villemard done in 1910, depicting Y2K. I love the wing-flapping fire-brigade and the low-pass bar guest ... lol.
As you'll observe - most ideas were actually in principle due at that time.
High altitude solar collectors intercept sunlight that would have shone on someone else's property. The royalties that would have to be paid to many land owners would increase the cost. One of these things shading my PV array, solar hot water collector, garden and forest would be unacceptable. Insurance would be needed in case the cable breaks and comes crashing down.
With a maximum sway angle of 15 degrees and an altitude of 20,000 meters, they would have to be spaced at least 10.8 km apart. The spacing probably should be increased to at least 20 km. to deal with failures. They should be located at least 20 km. from the nearest city. Antarctica and Arctic regions would be excluded due to low temperatures and no population. I am not sure of the surface area that exists outside of these zones, but the greater the distance from cities, the greater the cost of constructing transmission lines which you did not include in your cost estimate. The surface area of Earth is 510 million km2 with land occupying about 25%. The maximum number of 1 GW plants over land would be ~319,000 ignoring exclusion zones around populated areas.
The bit that's not called attention to is the 2km diameter of the collector, made of 50m bits. That's a big problem to keep optically useful. Don't see efficiency numbers for that not working to plan mentioned.
It also means that at 20km altitude it will cast a shadow that would be an issue on the ground (hello Mr Burns).
Insurance is less of an issue (you can make sure the unit floats away if the cable breaks).
Better bet seems to put the genset on the flying unit, do away with the light pipe, and scale it such that it can power a local area, high bandwidth, last mile connection for a city - with a superconductor wire to the ground to keep it working at night or feed excess power into the grid.
I can see many more uses for that than something best achieved with a nuke station.
If the cable breaks the solar collector would float away, but the cable would fall to the ground. There would need to be a procedure for retrieving a free floating solar collector with possibly a dangling cable before enough hydrogen leaks away to drop it down to Earth. I can think of several catastrophic failure modes:
1. An F5 tornado strikes the ground station blasting the cable not only with wind, but debris and softball sized hail traveling at 300 kilometers / hour. The cable could break near or at the base.
2. Did the engineers learn the lesson of the Tacooma Narrows Bridge? Large flexible structures are subject to resonance.
3. Because the configuration of the solar collector must be altered to be more aerodynamic when subject to high wind, there is a possibility the mechanical mechanism will fail or operators will fail to reconfigure it. If it remains in the open position, pieces of the solar collector may be ripped off by wind and come crashing down to Earth. The cable will be tilted far beyond its "maximum" 15 degree inclination before it breaks. If the cable breaks where it connects to the solar collector, it will fall to Earth likely laying across roads and power lines. A steel cable could short out two distant power lines.
4. It is shot down using a missile that ignites the hydrogen.
Basic safety precautions require it to be located away from populated areas.
Yes, I thought of that too----intercepting precious sunlight is like stealing. People with a lot of money and technological expertise are going to steal valuable sunlight before it has a chance to strike the oceans (where it will make food for fish), forests (where it will make trees and other plants grow) and people's fields (crops, rangeland) and it will even take the sunlight some poor person uses to dry his laundry.
This is a totally immoral technology that reminds me of the scene in The Terminator where all the oxygen is being sucked out of the room unless you can pay for it.
Poor people have for ages been fishermen, farmers, shepherds, woodcutters and so forth....in effect,collecting the sun light that strikes in remote places. They bring it to market towns or cities in the form of crops or products like fish. Now those people are still working at those jobs, actually they can make a living still.....but you want to basically steal that sunlight to use it for cars and computers.
This is a very shortsighted idea for collecting energy----I hope it doesn't work (I think there would be huge protests if it were tried)
People can actually live without electricity. Try it. People need water, food, air....but they do not NEED electricity. Electricity is a double edged sword----promising a cushy life and sophisticated information systems---but I fled with my two kids from a town near Fukushima and nuclear power was the reason. Electricity should not be a huge priority. Sure, a little is nice to have. But hours are spent watching silly movies, playing games, etc. It is a huge waste.
People should be talking about making do with less electricity, not being "consumed" with the idea of making more and cheaper at the expense of ecosystems including animals and poor/less technologically advanced people. Stealing from the poor/less powerful....by taking their sunlight....your idea is an ingenious but a truly hellish notion that I hope has no future!
Come to think of it, the amount of oxygen in the air will go down because trees will grow less if your plans come to fruition. Trees give us oxygen. Then megacorporations will provide precious manufactured oxygen at a premium price to those rich enough to pay, while your device funnels the sunlight to the same corporations. I guess it was only a matter of time before such an immoral idea would be dreamed up--- truly survival of the fittest. Those rich, urban, educated people will basically take steps to ensure that they get the sunlight ahead of the others.
I think your idea won't be politically acceptable outside the US and even would have problems in the US.
With all due to respect, this is a silly and hysterical rant. Do you accuse clouds and mountains of stealing sunlight? What about skyscrapers which cast shadow? As the sun moves through the sky the shadow caused by this device will also move. Nobody's house or garden will be in permanent shade. The real threat to forests is not shadows cast by space objects; it is chopping down trees for lumber, pasture, growing bio fuels, fire wood, etc. The amazon rainforest has been shrinking for decades and it has nothing to do with lack of sunlight. The same thing is true for forests in Indonesia.
"What about skyscrapers which cast shadow?" Posted by suyog
Actually, this is a major issue in many urban planning departments.
As for the overall scheme, it seems like something out of Popular Science 50 years ago. Or something the Antidoomer would have directed our attention to.
Antoinetta III
Clouds and mountains are natural, not man made. Mountains were present before the land was purchased and can be evaluated by the buyer for their effects on the property. Sunlight, as intercepted by a skyscraper, is subject to adverse possession from the land that is being shadowed. The same legal argument would apply to this tethered solar collector in the sky. True, it is unlikely that land would be subject to permanent shade, but what would be the effect of 10% shade every day? It might reduce plant growth, reduce power output from a PV system, reduce water evaporation or partially compensate for global warming in the shadowed region. Who knows?
I have more than 40 acres of land. In the summer on a sunny day my land receives about 940 MW·hr / day of sunlight. This project proposes to take some unspecified fraction of my solar energy to make electricity for their profit without my permission and with some predictable and some unknown consequences to my land. The legal issues of seeking permission, paying royalties or fighting it out in court are probably enough to bankrupt the proposal if attempted over privately owned land. If this was done over federal land, like a national forest, with support of the government, then it would have a chance.
With all due to respect, this is a silly and hysterical rant.
With no due respect - you do not understand the law and solar rights.
What about skyscrapers which cast shadow?
Solar rights are established in some of the United States and if the sunlight is blocked by a project, that has to be paid for, if the other party has solar energy capture up and operating.
OK, but do you think the shadow caused by this space object will cause forests to not grow, create a shortage of oxygen and then corporations will make mega profits by selling oxygen to rich people?
That is what makes the rant silly and hysterical.
Pi was referring to a country with corrupt leadership. If safety is cast aside in favor of a belief in infallible technology, I can imagine these collectors being spaced 3 or 4 km. apart and operated at different altitudes. During winter with a low sun angle they could intercept most of the sunlight. During summer they could intercept ~35% of the sunlight with a spacing of 3 km. Minimal spacing would take enough sunlight to alter the environment on the ground killing plants and animals.
Light-starved dystopia? Hardly. A nation that can turn even *1%* of the sunlight falling on its territory into electricity would be as close to an energy utopia as anyone could want.
For the US, converting 1% of incoming solar works out to 24 terawatts, about 6 times as much primary energy as we use today. 35% would be almost a petawatt. I don't even know what we'd *do* with a petawatt.
The proposal here points out that you can power *all of civilization* with a fleet of 15,000 of these beanstalks. Globally distributed, that's about one every 200 km. Now sure, we can imagine a society in which the ruling class lives in a gluttonous wasteful energy utopia at the expense of the shadowed poor, but you can do that with any energy technology. Your complaint is with the society, not the technology.
Meh, if they become a problem, destroying them will be easy. They will be fairly fragile in the scheme of things.
If the people with the radical thinking can blow themselves to pieces, what would stop them from blowing this $$$ investment?
With enough of these things deployed, they might offer enough wind resistance to damp out the jet stream in the tropopause. With the jet stream gone, the design job becomes much easier. ;-)
Perhaps with tight enough spacing the wind resistance of these devices in aggregate would be enough to dampen the winds in the tropopause and eliminate the jet stream. That would greatly simplify the design requirements for these devices. ;-)
Worst case shadow lasts about 45 minutes.
I think the worst case is longer than 45 minutes / day because the collector would be moving around in the sky in a conical area within 15 degrees of vertical as it adjusts to changing wind speed and direction. The location of the shadow would not be easily predicted. Because the collector is more opaque than a cloud, I expect the shadow would be darker than a typical cloud's. Even so, a shadow that lasts 45 minutes around noon would block 12% of my daily energy from my photovoltaic array. Even if they paid me a royalty large enough to expand my PV, solar hot water and passive solar systems to compensate, I am reluctant to experiment with shadowing my forest and garden. How much sunlight could be taken before causing problems with photosynthesis, temperature, wind and precipitation? The difference between summer and winter is about a 50% reduction in sunlight at my latitude.
Exactly. And it is possible to imagine some poor countries with corrupt governments selling their sunlit space in the stratosphere to rich countries, while their poor citizens literally starve in the shade. The rich countries get the cushy life, the electricity---the poor get nothing. Ecosystems need sun. You should not direct the sun preemptively to your collection unit before it strikes the earth. That is totally unfair and wrong. Especially as you will be charging people to use it!!
Clouds block the sun---but it is not the intentional hand of man that forms them for profit.
The shade caused by large buildings in cities has contributed to the fact that food cannot be grown there in large cities now, and the ways cities are so overbuilt continue to contribute to the "sustainability" problems the society faces as a whole.
Why doesn't this highly inventive scientist work on good cheap ways to take away large empty cement buildings from the landscape? Where I live, in Japan, we have a LOT of large empty cement buildings and NO good way to get rid of them because of costly oil.
And it is possible to imagine some poor countries with corrupt governments selling their sunlit space in the stratosphere to rich countries, while their poor citizens literally starve in the shade.
Isn't that how it is today with oil (e.g., Shell in Nigeria) ? Isn't that how it is today with any fossil fuel energy source?
I think you have a hyperactive imagination and no understanding of scale in this particular case. If a typical country can convert 0.1% of its sunlight into electricity that would be sufficient. If anything, this technology, if successful, would allow poor countries to improve the standard of living of their population. I don't think the "rich" countries are going to harvest solar power from the stratosphere of "poor" countries because the cost of long distance transmission cables would be prohibitive. It would be easier for each country to harvest sunlight from its own stratosphere. Even a cloudy place like Scotland could do it since there are no clouds at that altitude.
If a small number of people are adversely affected by this they could be suitably compensated. That is what we do today when we build a dam.
I am reluctant to experiment with shadowing my forest and garden. How much sunlight could be taken before causing problems with photosynthesis, temperature, wind and precipitation?
Quite a lot actually, depending on the plants. It might even screw up the flowering so you could go to a no-yield condition.
That's for a system large enough to use a light pipe. A system which uses airborne open-cycle gas turbines, or is simply semi-transparent for whatever reason, will be a much smaller issue.
At least in hot desert areas, getting a little bit of shade might be considered to be a benefit. But, then again, these areas usually aren't heavily populated, and don't have so much cloud cover to mess up ground based PV.
Well the solar collector could be placed somewhere it would not cast shade on land. Go offshore and have the solar power come down into either a electric generator ship or the fiber optic cable could come ashore miles after it reaches the ocean surface.
At the altitude of 12 miles up, the solar power will be at most three or four times what it will be on earth. (This does not guarantee that three or four times the solar power will be harvested.) Your concept, with the incredible amount of complexity and cost it would involve, could only be worth the effort if the solar power up there is 10-100 times what it is on earth. Just like EROEI, one needs a massive surplus in order to pay for the complexity. Then it would make sense to substitute several hectares of solar farms with one large dish in the air. As it turns out, it is less than 4X the intensity, so it seems to me that the dishes would still have to occupy a sizable area to produce power.
Here is a diagram I consulted:
http://home.iprimus.com.au/nielsens/Images/solsurfa.JPG
on this page:
http://home.iprimus.com.au/nielsens/solrad.html
I have checked out your website and I was quite impressed with the level of detail which has been expounded on the engineering and the costs. But I too have an engineering degree and one of the first habits inculcated into me was of finding the simplest and cheapest solution first and then adding complexity to it if it was absolutely necessary. Furthermore, the solution must have the minimum possibility and probability of failure, and if it fails, the failure must be as localized and restricted as possible. Your solution does not seem very robust.
Is it not easier, better and much cheaper to cover twice the area on the earth with solar collectors than to go 12 miles up and bring upon yourself more headaches than you care to imagine? If you are concerned with dust, solar panels installed on earth can be engineered to clean themselves at little cost:
http://techcrunch.com/2010/08/23/self-cleaning-solarpanels-could-boost-s...
I can name so many other disadvantages, but chief among them, it seems to me, is the only marginally extra energy available up there in the stratosphere.
Why bother going up into the air if, with some research and advancement, solar power on the ground can be refined and made so much more profitable? Is there a shortage of land for solar collectors? Are there not hot arid deserts around the world with hardly any life in them where solar plants can be erected? I understand that some nations are not blessed with tropical sunlight and live under constant cloud cover, but can these nations not form alliances with other nations where there is open arid space with abundant sunlight? Even this would be simpler and cheaper than floating dishes in the stratosphere or beaming solar back to earth.
Am I missing something?
But assuming that this idea does indeed work, it would be vulnerable to sabotage - BY ME!!! How I would despise such a grotesque tentacle reaching upwards, symbolizing this wretched civilization's next attempt to suck the life out of the air after it has finished sucking the life out of the earth!!! How horrible it looks, how imposing, how like a giant black parasitic weed, of which we would be the engineers. If I don't like a factory or an oil refinery, I can choose to move far away from them. But if I don't like Jack's beanstalk, how will I remove it from my sight? Perhaps the thin strands of powerline might fade from view as one moves farther from it. But think of the shadowy rays which the giant solar arrays would cast from 12 miles up. What place nearby would this hideousness not invade with its presence? I would have to live hundreds of miles away just to get away from it.
So to conclude, I hate the idea. I hate it as an engineer and I especially hate it as an aesthetician.
Something has got lost in traveling from 1977 to 2011, as all the info I can find, mentions 1366 w/sqm in space, and ~1000w/sqm best case on earth surface ?
Your figures of 342 [W/m2] seem very strange.
Just one reference :
Over the course of a year the average solar radiation arriving at the top of the Earth's atmosphere is roughly 1,366 watts per square meter[2][3] (see solar constant). The radiant power is distributed across the entire electromagnetic spectrum, although most of the power is in the visible light portion of the spectrum. The Sun's rays are attenuated as they pass through the atmosphere, thus reducing the insolation at the Earth's surface to approximately 1,000 watts per square meter for a surface perpendicular to the Sun's rays at sea level on a clear day.
Of course, the point about power ratios is valid, even more so if the Air-loss is only 366W/kW : (a loss of 23%)
That means you only need 36% more land area, on ground level, for the same peak powers (noon values).
Of course peaks are not as important as area under the curve, and the claim was the Upper atmosphere was almost rectangular, so we could guess ~11h/24h delivery factor.
On earth, those same delivery factors are never higher than 6/24, and around 20% is a more workable average.
http://www.solarpanelsplus.com/solar-panels/large-insolation-map.html
These combine to give a ballpark of ~3, for clear day values.
If we consider the earliest-deployed Solar PV is best offsetting AirCon loads, clear days are well matched.
So we have a ceiling cost factor, of ~3x that of an Earth based PV plant.
That seems a very low price ceiling, and there are a LOT of critical failure nodes in an airborne design, whilst a ground system can easily and cheaply be serviced with low capacity impacts (only the panel, or inverter, being fixed needs removal.
Then there is insurance, and the simple physics of falling-on-failure.
If/when this thing fails, it will do so in a truly spectacular way.
( and it will, what goes up, must come down..)
It would be a massive You-Tube hit!
50m diameter of plastic/Al light pipe, is likely to literally shatter into pieces, as it hits the ground, and I get around 60 kilotonnes of TNT energy released.
( or 3.88 Hiroshima bombs, if you prefer.... ) - (simple ballparks only)
I guess you could make a giant retract-a-coil, in a sacrificial emergency pull-in, but it needs to wind at terminal velocity speeds (and so have huge amounts of energy, right when you likely have none coming down the tube... )
One-off engineering projects, like this one, ALWAYS suffer cost explosions.
A solar company recently burned through 0.5 Billion, of taxpayer money, without breaking a sweat, on what was a simple fab build. (already been done before)
>> 20x that is easy to expect.
Then there is opportunity cost: A good litmus test, is what size PV FAB, could be built for the same spend ?
Remember that PV FAB, creates that additional power, every year, year after year.
Sticker prices seem to be ~$2.4B for a 1GW fab, or ~$350M/GW amortized over 7 years.
Hard to see this getting ANY venture capital ?
Whoever published the webpage (not me) meant that the solar energy falling on a flat surface perpendicular to the sun's rays is 1366 W/m2. However this power is spread out over a round hemisphere, where intensity is highest at the equator and falls off towards the poles with the angularity between the solar rays and the ground. All he did was give the entire globe an average of 342 W/m2.
As for the percentage of solar energy which is lost in the atmosphere, the StratoSolar people claim:
"The PV panels are exposed to 1.5 to 3.5X the solar energy (in kWh) of ground-based PV panels" -- http://www.stratosolar.com/
If these figures are true, then, to my mind, in order to make up for fluctuations and inefficiencies, the system must be designed to harvest and profit from let's say 2X of the amount of solar falling on the ground. That's a very marginal amount to chase after with such complexity. Much has been made on this site about the possibility that Oil with an EROEI of less than 5:1 is just not worth extracting, because so much of society's resources would be used to produce energy. The Return on Investment on this floating solar array will probably be smaller, if not negative. Furthermore, there is the very serious matter of whether there will even be enough raw material with which to manufacture the colossal quantities of solar PV's and concentrators to offset declines in fossil fuels. No one in the solar industry seems to understand that the materials needed for solar power are scarce and their currently low cost is heavily subsidized by fossil fuels. We know that fossil fuels will increase in price, but once more demands are made for rare earths, the cost of the raw material will also skyrocket. So it's likely that solar power will increase in cost over time.
1000W/m2 & 342W/m2 The difference between instant power and averaged over 24 hours.
NAOM
Or, the difference between the area of a sphere and a disc of the same diameter. Four times.
LOL! Well, thanks for your honesty. Good to know where you're coming from. Have you met Ted Kazinsky? You might find you have a lot in common.
Actually I have some sympathy for your outlook. I've always loved nature, and hated the intrusion on the landscape that power and phone lines represent. And don't even get me started about wind farms! However, I think your reaction to this particular concept is misplaced. Particularly if we talk about one of the thin tether approaches.
Artist's concepts not withstanding, the fact is that the system would be essentially invisible from the ground. From 200 meters away, you'd need binoculars or very sharp eyesight to make out the tether against the normal brightness of the daytime sky. The floating collector would loom huge if you could see it, but you couldn't. It's above most of the atmosphere where scattering of light occurs, and its bottom side is only dimly illuminated by backscattering from clouds and earth below. It would be less bright than the daytime sky in front of it. It would only be noticeable when you were within its shadow -- which somebody noted would be a maximum of 45 minutes per day, at times of the year when the sun's path from your location happened to cross the collector. And the shadow wouldn't be particularly deep. You'd still have illumination from scattered light over most of the solid angle of the sky, so it would be brighter than what you get with low-lying cloud that blocks sunlight over a much larger solid angle.
Seriously, I consider minimizing visual and environmental impact from large-scale solar power to be one of the strongest points in favor of the StratoSolar approach over ground-based solar.
Interesting, maybe you should join forces with people who are designing jet-stream turbines? I'd think there's a lot of knowledge to be shared.
Excellent fresh thinking on the subject. I'm not convinced that it would actually be maintainable, but it's worth the effort to see.
Questions:
1) What kind of a heat exchanger are you using to get to 1400 C and 20 bar?
2) 15,000 1GW plants is a whack of precision hardware. Which we already have. Existing power plants tend to be away from urban areas too. Steam is steam. Have you considered locating the collector arrays above existing coal fired plants?
3) Would the shadow even be noticeable on the ground, considering diffraction?
To question 3: there would be a "shadow" but it wouldn't be well defined. It would result in local diming, kind of like a thick stratus cloud.
I'm not sure why this would be an issue in areas of the world that are generally cloudy. You're not really "stealing" sunlight if that sunlight would otherwise be absorbed by a cloud.
You're not really "stealing" sunlight if that sunlight would otherwise be absorbed by a cloud.
And yet that is not how the law treats shadows made by Man that blocks Solar from hitting installed solar capturing devices.
http://www.statesadvancingsolar.org/policies/policy-and-regulations/sola...
I think you are mistaken about the shadow. At a distance of 30 km., a 2 km. diameter object subtends 3.8 degrees compared to Sun which subtends .5 degrees. It would completely eclipse Sun making it dark enough to see stars in daytime. It would look like a solar eclipse.
No, it would not. The diameter of a solar eclipse umbra is about 100-200 km. In a solar eclipse, not only the viewer on the ground but most of the atmosphere he can see in every direction is in darkness. So no light is scattered from that atmosphere, and the blue sky turns black.
With the beanstalk solar plant, while the viewer receives no direct sunlight, the atmosphere around him is brightly illuminated, so it will scatter blue light toward him. The viewer sees, and is illuminated by, blue sky all around him.
It's more like a cloud shadow, and nothing like an eclipse.
Right. Good explanation, Goodman.
200 km. is the approximate maximum size of the umbra during a solar eclipse. The minimum size can be zero depending on the distances from Earth to Moon and Sun during the eclipse. The best comparison would be with a total solar eclipse in which the diameter of the umbra is a few kilometers. In a total solar eclipse it becomes dark quickly upon entering the umbra as the last direct ray is blocked by Moon despite sunlight being scattered as it passes through the atmosphere in the penumbra. This solar collector is large enough to block the direct light from the corona. Clouds typically pass 25% of the sunlight. The solar collector would cast a visible shadow on clouds because it would pass no sunlight. It would be blacker than a dark rain cloud, but nothing like a puffy bright white cumulus cloud.
Nope, not a good comparison at all. The penumbra in any solar eclipse (the region of partial eclipse) is 6000 kilometers across. Twice the diameter of the moon. For several hundred kilometers surrounding the umbra proper, there is near totality, and the atmosphere is only minimally illuminated. That's why the sky gets dark enough to see the brighter stars.
There are two main factors that affect the ability of the unaided human eye to see planets and stars in a clear sky in the daytime: Rayleigh scattering of sunlight in the upper atmosphere and the dark adaption of the eye. I have personally seen (unaided eye) Venus from sunrise into the afternoon at sea level. It will not require much reduction to make the brighter planets and stars easily visible. With some of the scattered light and all of the glare of Sun blocked, the sky will be darker and the eye better adapted to see faint objects.
In regard to 1, I don't know what will work best. My current thought is a transparent window, perhaps silica or alumina and a cavity type absorber, perhaps a 1 cm grid 15 cm deep. http://www.freepatentsonline.com/4033118.pdf The window has to be kept cool to keep its strength.
For 2, yes. Four Corners where they have 3 .7 GW units would be a good example of where coal could be replaced by a StratoSolar type system.
Re shadow, the extended size of the sun would be a bigger effect than diffraction I think. At a 40 km slant distance a 2 km disk would be around 3 deg, which is considerably larger than the sun. It's not going to be dark though because of scattered light.
Interesting work on that black body collector. In column 11 line 10 of that patent, they indicate that current materials limit the upper temperature to about 1200 C. Column 2 line 10 describes the arrangement in Figure 1 where the heat is absorbed by the inner glass/quartz lining and then transferred to the working fluid by forced convection. Column 11 line 50 describes the arrangement of Figure 2 where the central cavity is filled with transparent fluid which cools the cavity wall from within.
In both cases the heat is first absorbed by the structural pressure barrier and then transferred to the working fluid. I wonder if you could achieve a higher working temperature by absorbing the light energy in the fluid rather than in the structural material. A fluid containing finely pulverized black particles could absorb light very well, the heat being efficiently transferred to the fluid due to the large surface area of the particles. Re-radiation would be mostly re-absorbed within the bulk of the fluid.
The temperature limit for the dust would be when it softens enough to accumulate on impinged surfaces. And there could be erosion issues. However without strength considerations you have a wide choice of particulate materials, perhaps a ceramic or metal oxide. And of course the particulates would have to be removed in a cyclone separator before routing through a turbine. However if these problems can be worked out, it's hard to beat black dust for heat absorption.
Of course, one can just extend the light pipe a little farther, terminate it at a mirror and heat the cavity from below. The gas will tend to be stratified and any window will remain much cooler.
Unfortunately, that would eliminate the 'black body' effect provided by the cavity. As the OP mentioned, infrared radiation increases with the fourth power of absolute temperature. At temperatures high enough to run an efficient heat engine heat loss at the absorber is a limiting factor.
The emissions spectrum of the cavity is very different from solar radiation. A selective-transmission coating on the window, or provided by an IR-opaque coolant, will cut heat loss. Keeping the window cool is much easier if convection isn't bringing it right up to the cavity temperature absent some form of heat removal.
Perhaps I misunderstood your first comment. You are proposing to heat the reciever cavities from below? In that case you would have a row of cylinders illuminated by sunlight. Am I reading that correctly?
The point of even having a bunch of cavities is to get the sunlight deep into a container before it is absorbed. This approximates the performance of a blackbody absorber. Heating the absorbers from below would negate that advantage, and result in large amounts of heat loss due to infrared radiation.
There's nothing saying that these cavities need to point upward. It could work out to be more efficient to point them all downward and illuminate from below.
In any case convection would be a minor effect. To provide sufficient cooling, the fluid would be moving through the reciever at a goodly fraction of the speed of sound.
That's precisely what I was suggesting.
I don't think you appreciate the scale of this thing. The receiver cavity opening would have to be at least as big as the light pipe, or 50 m diameter. Assuming an air density of 4x standard sea level (20x pressure, 5x absolute temperature) and 1005 J/kg-K specific heat, moving 2 GW of heat with a delta-T of 500K requires less than 4000 kg/sec of air. That's about 800 m³/sec, or a flow of about 40 cm/sec over a 50 m diameter circle.
Hmm, that's surprisingly slow. I figured they would want to have an array of smaller receivers to allow for maintenance without shutting down the whole plant.
"A fluid containing finely pulverized black particles"
It's such a good idea it's already being done. :-) I don't have a pointer to it, but one of the solar absorbers on a CSP plant uses oil behind a window with fine back particles in oil.
Kevin Parkin and I were discussing this last week. Beamed energy propulsion has the same problem.
You are absolutely right, "it's hard to beat black dust for heat absorption."
In this application it might be best to use two fluid streams. A clear fluid (such as air) would flush through the upper part of the cavity near the window. A black fluid (such as air carrying dust) would circulate through the deeper part of the cavity.
This would allow the light to be efficiently absorbed in the deeper area of the cavity while keeping the window relatively cool. It would also allow the use of a small flat quartz window instead of a shaped cavity formed of doped quartz.
How do you get the light to impinge on the working fluid. Considering the working fluid is at 40 bar.
Diamond window.
(Knocked that one out of the park, didn't I?)
It's 20 bar, and a quartz window would do just fine.
Bah. Quartz is boring. I was relishing the thought of a project where an optically perfect sheet of industrial diamond 50 meters across was just Part Number 53297.
"optically perfect sheet of industrial diamond 50 meters across"
That's a big ambitious. :-) But NASA recent spent $2 M buying Dr. Kevin Parkin a gyrotron for testing beamed energy propulsion heat absorbers. It has a 3 cm diamond window that passes 1.2 MW of 110 GHz microwaves. If I didn't drop a decimal point, that's 17 GW/m^2. Gyrotrons of this size are not exactly off the shelf, but this is number 2 or number 3 of that design.
Beamed energy propulsion is one way to get the cost to GEO down by a factor of a hundred or more.
One of the major complaints about renewable energy is that it is intermittent so you need energy storage--and good cheap energy storage methods are lacking.
But the OP has presented one. Why is no one talking about this?
Because that's not the new part. The Andasol solar thermal plant in Spain has been running with a heat storage system since 2009.
http://en.wikipedia.org/wiki/Andasol_1_solar_power_station
I think it's likely that as people proceed from the predicament we are in there will be revolutionary technologies, like this, that will eventually be implemented. I'm not trying to say that technology will save us from all hardship by any means. But I do believe that if history is any reference, the future will hold stunning new technologies and strange-to-our-eyes morphs of current technology that will finally surpass barriers that seem insurmountable now. This seems to be a good example of a powerful new turn solar could take.
I have no expertise or insight to offer on this topic, and I really have no idea how feasible it actually may be. I only wish to offer encouragement to you and anyone working on cutting-edge ideas in renewable technology. As far-fetched as it may seem to some now, these kinds of things are exactly what we need to be thinking about, and it's only a matter of time before ideas like this get off the ground, so to speak.
here's to working towards paradigm shifts in renewable energy technology!
Pie in the sky.
The CSP "light pipe" version offers an enormous cross-section to the turbulent lower atmosphere. So even with fairings, I'm visualizing it going the way of the original Tacoma Narrows Bridge (aka Gallopin' Gertie.) It might take quite a bit of very expensive supercomputer study to deal with that - has such a study been carried out?
I wonder what the world record is for tethered devices. How many miles was the tether and how large was the object that was tethered?
I dunno, but I doubt anybody has ever tried to tether anything with a tether that's 50 meters in diameter, like the light pipe. That surely takes it to a whole new level, by two or more likely three orders of magnitude. And, of course, world records of that sort are often set under ideal conditions, with the record-setting item taken down again before the conditions become less than ideal.
I believe the military and Homeland Security fly tethered aerostats up to 10 - 12 km. The tethers do include conductors for power delivery to the phased array radars and radio gear that the aerostats hold.
The full scale stability of the light pipe has not been done. But the Aero people we talk to seem to think it is highly damped and not a problem.
What I think is more of a problem is when the wind is blowing at nearly right angles to the concentrator. Like this wildly waving stop sign.
http://www.youtube.com/watch?v=O53a2yn-BI4
But the aero controls guys say a canard sort of wing out into the wind on the upwind side and some fancy control electronics will tame it. I want to see a wiggling stop sign in a wind tunnel stop waving when equipped with these controls. Don't doubt them, just want to be sure they have the controls set right.
As with the stop sign effect, I think I'd want to get some assurance on "But the Aero people we talk to seem to think it is highly damped and not a problem" before putting much real money into such a scheme. Their intuition might be fairly good with respect to local effects (and in such an enormous structure, even the aerodynamics of the Hindenburg would be merely a local effect.) Among other things the tether has to be damped in all oscillatory modes including large quasi-global ones, and for torsion, stretching, and perhaps shape-distortion, as well as the obvious "shake the rope" lateral waves.
Identifying all the possibly-relevant modes in a huge squishy structure like the faired light pipe would be quite a challenge, one that would surely need to be taken well beyond intuition. While there is certainly plenty of precedent for tethered aerostats, the usual tethers are cables having nothing like the enormous cross-section of the light-pipe and its fairings - and the devices are often taken down when storms threaten, which would not be practical with a 20km light pipe with a huge power collector at the top.
"as well as the obvious "shake the rope" lateral waves."
If the design were tapered the other way we would have serious problems such as a "Crack the whip" effect from a big earthquake. But since it gets bigger as it goes up, lateral waves damp out rather than being amplified.
I agree with you entirely that it needs serious analysis for all possible vibration modes. And that it is not practical to take it down so it must be able to take whatever stress it is subjected to.
I wouldn't think an earthquake would be much of an issue for it. The amplitudes are not very high, and the mode frequencies of this baby, are likely pretty low. Wind would be a much bigger issue. Imagine this thing in a severe thunderstorm....
Agreed. I'd like to see a thunderstorm survival design for this thing. Keep in mind that thunderstorms can have peak vertical velocities exceeding 50 m/s, extending from ground level up to the tropopause (10 km), and can pop up almost anywhere with less than an hour's warning.
"peak vertical velocities exceeding 50 m/s"
Maybe I am missing something, but I don't understand how vertical winds up or down put significant stress on the structure.
In a word: turbulence. Vertical winds would be no problem if that's all they were. I.e., if they were well-behaved laminar flows. But the high sheer between rising and falling columns of air in a thunderstorm generates strong horizontal vorticity in the boundary region. Transient horizontal velocities will hit peaks as high as the vertical velocities, and they can reverse directions within seconds.
That's not necessarily an insurmountable problem for the light pipe approach. Keith and I have talked about this a little. The turbulence in the vertical air movements manifests itself at the light pipe wall as pressure fluctions, with magnitude comparable to the stagnation pressure of 50 mps wind. If the light pipe carries an overpressure a little higher than that, it can withstand the fluctuations. They're relatively short range, and cancel each other out over any few hundred meters of the pipe.
I had initially thought that the overpressure requirements, and the need for the pipe to act as a rigid tube over distances of a few hundred meters, would make it impossibly heavy. But as it happens, Spectra is so increadibly strong that the pipe could carry even a full atmosphere of overpressure without adding excessively to its weight. So maybe the light pipe could survive thunderstorms.
Nonetheless, the uncertainty and the need to rigorously prove the design against worst-case scenarios is one of the reasons I'm more comfortable with the thin tether PV approach.
Also, there is rain and ice formation at some intermediate elevation, somewhere along the pipe and fairing. Maybe heavy enough to load the fairing enough to rupture the plastic at stress concentration points.
That's another virtue of thin tethers: they are feasible to heat for de-icing purposes.
The outer membrane and the supporting structure that transfers the aerodynamic forces to the light pipe are similar to a stadium roof. Those seldom take damage even from the most violent thunderstorms.
"I'm more comfortable with the thin tether PV approach."
In terms of it working as advertised, so am I. And something like it is on the path to the concentrated solar version regardless.
But it fails to meet my criteria of electricity cheap enough to make synthetic gasoline for a dollar a gallon.
I think your criterion is the problem there. Gasoline motor fuel is a means of converting petroleum into handy transportation energy. If you're starting from something else, gasoline is probably a bad pick for the medium.
As a for-instance, if the nation is covered with large stratospheric solar collectors, those same collectors could be used as microwave transmitters to power airliners. There goes the need for kerosene just to move airplanes around, and the silliness of converting solar electricity to mid-weight hydrocarbons.
Let's look at it from a cost benefit perspective. The system described here is an order of magnitude more complicated than a ground-based solar thermal plant: if it doesn't also cost an order of magnitude more, I'll eat my hat.
What do we gain from that complexity and cost? More power per collector area. But how much?
A traditional ground-based solar thermal power station has a capacity factor of about 25%. That's due to clouds, solar angle, and nighttime darkness; if you could eliminate the solar angle and cloud issues, nighttime darkness would still limit it to a 50% capacity factor.
Your floating sunflower *does* eliminate the solar angle and cloud issues, but it's still in the dark half the time. Thus, it can produce at most twice as much power as a ground-based system of the same collector area.
So here's the decision-making tradeoff: if we want a gigawatt of power, we can either build *one* magical floating beanstalk with 3 square kilometers of collector area, OR we can build *two* boring ground-based solar thermal plants each with 3 square kilometers of collector area.
Which of these two options is cheaper? I know which one I'd pick.
The one that includes finding a way to make human population reduction a palatable goal for everyone!
And then teaching the remaining human population to live meaningful lives without the massive waste of energy and resources which we seem to think is necessary for a satisfactory quality of life.
Perhaps we should submit the implementation of this abomination of an idea, to a binding vote by all the members of all indigenous and aboriginal peoples of the planet. Only they would be eligible to vote!
I don't want to hear any more idiotic ideas on how to produce MORE F@**G ENERGY,FOR MORE F@**G PEOPLE! doesn't anyone else get it that ideas like this are the epitome of pushing the right leverage point in the wrong direction?! /rant
My apologies to Donella Meadows.
Right on, Fred!
At a time when the "Space Program" is foundering and falling apart from an inability to obtain funding, all sorts of social programs are under threat because of lack of funds, we're cutting the education budget, etc., these "Great" ideas keep being thought up. I keep wondering how disconnected I'd have to be from reality to not see that these things have absolutely no chance at all of finding funding beyond even a small initial "toying around"? And, like Fred says, the wrongest of the wrong way to go!
I live in a world of reality, not one dreamed up by some SF author and understand that "Star Trek" isn't real. [edit]
Let's avoid personal attacks, please.
All the best,
K.
Yes---it does seem strange that noone else mentioned how the educated and wealthy are stealing the basic source of sustenance (sunlight) from the already under-pressure, already marginalized aboriginal peoples.
A horrific plan, devious, rapacious....STOP THIS before it gets off the ground, so to speak.
"A horrific plan, devious, rapacious"
In that case, you should be a big fan of space based solar power. That method diverts light that would never hit the planet into cheap electrical energy. SBSP was the subject of the last article I did for TOD.
Believe it or not, that's the option that I favor as well. I think the same could be said for many others, like StratoSolar associates Ed and Keith, whom you would deride as "techno-cornucopians".
There's no mystery about how to make human population reduction a palatable goal. It's a demonstrated and well-proven approach. It involves the liberation of women, and the creation of an economic and social environment where life offers interesting possibilities. Specifically, possibilities that don't involve raising a large brood of children to serve as captive farm labor or as expendable troops to strengthen the patriarch's position against rivals. It involves setting the expectations for child rearing to the point that the decision to have a child is seen as a costly one, undertaken only by those who are seriously committed to it.
You can find example after example where those conditions have been achieved, and birthrates have fallen below replacement levels. That's the "benign demographic transition" that we would like to see achieved on a worldwide scale. But we have get there first. Right now, there are some pretty powerful forces driving in the other direction.
One of the strongest of those forces is the doomsday thinking inspired by peak oil. Man the lifeboats! To hell with the poor! Hoard your capital! Prepare for the coming resource wars! Sew dissention and conflict among those who might otherwise challenge you! That's what's happening now, friends. You bemoan the fact that so many folks don't "get" PO? Oh, they get it. They get it too well, the movers and shakers.
As a dedicated techno-cornucopian, I care not a whit for preserving the population's ability to continue in a profligate consumption-oriented lifestyle. I do care immensely about overturning the destructive pessimism that is sending us down the road we seem to be following.
Roger, Roger, Roger that's not like you at all.
Are you really insinuating that destructive pessimism is the source of our woes? If it is lets banish it..............er where is it first, let me at 'im, don't hold me back, I wanna kick it's ass.
The source? THE source? Sorry, no such animal. Sources have sources that have other sources, ad infinitum.
The thought and behavior patterns that have me so exercised now are hardly new. They've been around for as long as people have been. Longer, actually. You can observe them at work in tribal apes and to a lesser extent in other social species. I think of it in terms of the Prisoner's Dilemma -- the eternal tension between Mars and Minerva, Ares and Athena. Competing mindsets, different game strategies in the long saga of evolution.
What's new, at least in this age, is the apparent ascendency that Mars has gained over Minerva. And yes, I do really attribute it to destructive pessimism. But I should qualify that. The pessimism I'm concerned about is not something you'd find on direct display in popular culture or in the MSM. It's more cryptic. If you're a player, and you believe that the market is about to crash, you won't run out and tell the world. You'll assert loudly that everything is just fine, while you proceed to unload your holdings onto the nearest sucker who will buy.
My feeling that destructive pessimism threatens our prospects of negotiating the coming bottleneck is not based so much on what I hear in popular culture, but what I see unfolding in Washington and the world in general. A disturbing number of the rich and powerful are behaving as if they expect the worst. If enough influential people believe that, it becomes self-fulfilling prophecy. We need to find a way to reverse that.
http://aljazeera.com/programmes/101east/2011/08/2011830104236992830.html
Indonesia: Bursting at the seams
There must be optimism somewhere here...........
Good case in point. Indonesia has never achieved the conditions required for a benign demographic transition. If they become mired in war and fomented ethnic conflict, they never will.
Upon closer inspection, that statement though oft repeated and consistently held up as an unassailable fact may not even be completely true. At the very least one may wish to remember that correlation doesn't always imply causation. http://xkcd.com/552/
http://www.populationpress.org/essays/essay-myths4.html
There most certainly are! Which is why I referred to Donella Meadow's Leverage Points: Places to Intervene in a System
http://www.thesolutionsjournal.com/node/419 in my rant up top.
My contention is that it is your goal itself that is the problem, its technological feasibility therefore being almost completely moot. I view it in the same vein as I view attempts at solving anthropogenic climate change with Geo-Engineering schemes such as seeding clouds with sulfate particles, putting up space shields or fertilizing the oceans with iron to promote algae growth. They might even work technically speaking but the very fact of their existence is a blatant example of attempting to continue with a completely failed paradigm!
I think it is past time to let go of the paradigm whereby complex technological solutions are proferred for the purpose of allowing things like growth based economic development to continue.
http://xkcd.com/556/
Cheers!
There's not a lot on which we actually disagree, Fred.
Your observations on the BDT, in my opinion, are valid; it's not something that magically follows simply by raising women's education levels. It's a complex matter of culture and expectations. Which is why I wrote "liberation of women", not "education of women". I have disagreements with V.A, but I strongly agree with her central premise. I believe that women / couples can and do regulate their fertility on the basis of expectations for the future. The perception of a high "opportunity cost" from having children is key to the BDT.
I also agree, in general, with your point about the importance of goals, and the inappropriate nature of many goals that are driving society today. But goals are not singular; they're elements within a hierarchical and interlinking system of goals and subgoals. I think you misunderstand my own goal in working to advance the StratoSolar concept. I'm not interested in advancing the cause of cheap sustainable energy for its own sake or in order to enable the continuation of BAU lifestyles. I'm interested in doing what I can to change the "facts on the ground" in a manner that helps to void the rationale for resource wars and policies aimed at preventing the rise of competitors.
Imagine that you're a paramedic on the scene where a flabby, overweight gentleman is gasping in pain from what appears to be a heart attack. From the sidelines, an onlooker chides that "well, of course he's having problems with his heart. His lifestyle is a mess. He needs a healthy diet and exercise. That heart medicine you're giving him will only allow him to continue his current wretched lifestyle." A healthy diet and exercise is all well and good, but the patient first has to survive long enough to adopt them.
Well, Ok Roger, but that's quite the defibrillator your trying to build there...I don't think the patient's health insurance is going to cover it. May just have to do some old fashioned CPR and give him some aspirin and hope for the best.
Cheers!
LOL! OK, Fred, I'll give you that point. :-)
Roger, two points:
1. Surely, you must see that population growth (aka extreme poverty) is but half the problem, if that. The societies that are at or near ZPG use more than half the world's energy with much less than half its population, and do so because of capitalism and its effects on the structure of life, not their rate of population growth. Political economy, not just mere existence, drives lifestyles.
2. One could easily argue the opposite of your point about PO and doom. Rosy, techno-cornucopian assumptions distract attention from the issues at hand. To my eye, that problem is far worse than the reactionary survivalism you mention. Our only hope is to get the public the news about impending doom. We can win the fight over how to survive that follows that. But, unless some still nonexistent technology really is going to rescue us, we are truly doomed if all we ever hear is that some future technology is going to rescue us.
What we need instead is the right blend of doom and promise, pessimism and optimism. The key is addressing our social institutions and the economic divisions they perpetuate.
"What we need instead is the right blend of doom and promise, pessimism and optimism. "
I really wonder what that blend might be?
I am fully aware that running out of cheap energy will stave a substantial fraction of the world's population. I know of two ways to solve the problem at relatively low cost (relative to the money spent on energy today) and am willing to consider any others people want to propose. I get next to no traction on these ideas.
If you have any thoughts on how to do better, please let me know.
One of the things that triggers large family size is nutritional deprivation of females in utero.
http://www.ncbi.nlm.nih.gov/pmc/articles/PMC2569844/
Fix that and (possibly) women will have smaller families.
Keith, I would repeat my objection to Roger's post: Population is only half the story, and not the one that is most immediate and pertinent within places like the USA.
Lifestyle is as important as population. Think back to the old environmental impact formula, i.e. P + A + T. That is pretty rough, but the point was clear even there: The forms by which we accomplish work within the richer societies = A + T. If we want to reach people here, we need to find ways to explain how wasteful and dangerous existing technologies are.
The task is inherently hard, the odds long. The powers-that-be are 100 percent invested in perpetuating present waste streams, with only minor, mostly gestural adjustments. They also utterly own the mass media and the political system. So, our job is to get as clear and honest and direct and respectfully realistic as possible, and then just keep trying (and waiting and hoping for our big moment).
Unless this approach is substantially less expensive, there is no reason to build it.
But take your 50% capacity factor. Being above the clouds makes the energy down the light pipe almost as predictable as the sun coming up. So we know exactly how much really cheap heat storage we need to keep it running overnight, we don't have to cope with a week of cloudy weather.
Based on the cost of plastic, aluminum, steel etc., we get around $1.2 B per GW. That corresponds to around 1.5 cent per kWh.
And the energy payback time looks to be around 2 months.
I am sure you can look up the numbers for the energy payback time and cost per kWh for ground based.
Based on the cost of materials, the cost of a Boeing 777 is about $400,000. (Actual retail price is about 1000 times greater.)
We're doing aerospace engineering here. The cost of materials is almost irrelevant: what matters is the cost of *complexity*.
Big these things are, no question about it. But complex? I don't see one being much more complicated than a building with a plastic stadium roof. They are not cheap at that, costing maybe 3 times the cost of a 777. But that's something like 1/5 of a nuclear plant of the same size.
I'll grant you this is less complex than a jet aircraft, but by less than you'd think. Off the top of my head:
* Redundant computer control systems which balance ever-shifting aerodynamic loads against optimal solar collection
* A physical frame that remains rigid despite being several km in length, width, and height
* Two thousand independent mechanical servos which control each mirror
* Massive steering propellers with huge electric motors (you proposed using something on the scale of a commercial wind turbine)
* Power plants to create electricity to power the servos and turbines
* Electrical systems to deliver power to the servos and turbines
* Hydrogen plumbing, pumps and valves to refill buoyancy cells
* Fire suppression systems
* Sensors to monitor heat loads along the light pipe to avoid thermal catastrophe if the mirror coating is damaged
* Human access systems to fix something if it breaks (either a 20 km elevator or a ballistic vehicle)
* Human life-support systems (space suits, airlocks, etc)
* Cargo delivery systems to carry the largest replacement part in the system.
* Either a self-destruct system or a titanic insurance policy to cover risk from falling heavy equipment in the event of catastrophic failure.
Notice that most of these are safety and repair systems. The system is pretty simple if nothing ever goes wrong. But everything always breaks.
So yeah, it's kind of like a plastic stadium roof, if you scaled the stadium up to cover all of lower Manhattan, filled it full of hydrogen, sent enough light energy through it to instantly vaporize anything but a perfect mirror, and put it in an environment that's instantly lethal to humans.
Good list. You have a good feel for the outrageous scope of StratoSolar.
The 20 km elevator is expected to be a cash cow at least for the first few. We probably will sell rides up it for parachute jumpers.
Good job of extrapolating from a popular kind of article to most of the serious problem we have considered over the past couple of years.
Only thing I might argue with is an airlock. At 60 mph it only takes 12.5 minutes to go up or down the elevator. It would probably be less trouble to go down for lunch than to get into a pressurized lunch room at the top.
I suspect that a pressurized bathroom will be required (can't take off 25+ minutes whenever nature calls) and a lunchroom can just be a kevlar balloon with a pressurization pump and an ozone catalyst. Air will be a tad dry, though.
It would be a lot quicker to get down with a parachute than an elevator. Free skydiving could be a perk of the job.
Depends
NAOM
Let me be clear that I'm typically enthusiastic about projects of outrageous scope and complexity. An Afro-eurasian power grid pumping solar and wind power from the Kalahari to Vladivostok? Go for it. Massive deployment of ocean thermal energy conversion? Hit it. Turning the Great Lakes into a terawatt-class pumped hydro reservoir? I'm listening.
But outrageous projects need to provide outrageous benefits over the mundane.
Yes, the airlocks are optional, but I wasn't thinking of a pressurized breakroom: I was thinking of the bends. You can't do delicate repair work in a 1-bar pressurized suit: to keep the gloves flexible, you need a low pressure pure oxygen atmosphere. To avoid the bends, the workers either have to either purge nitrogen from their bloodstream on the ground, which means living in a 1-bar pure oxygen room (Apollo 1) or go through decompression in an airlock at altitude.
There are lots of ways to do this (the elevator car can be the airlock, for example), but the difficulty of manual labor in a pressure suit is a huge issue. Among other things, it means you can't rush people up to do emergency repairs, and you should expect work to proceed at a NASA astronaut pace, in which unscrewing 10 bolts is a hard day's work.
You wouldn't need a room all the time, an oxygen mask would do it. On the other hand, if you used a room then below 1 bar would be better. The nitrogen would leave faster which is the idea. Also 1 bar pure oxygen for long durations is bad for the body. A nitrox mix could be used with just a reduced partial pressure of nitrogen and, if using a reduced pressure room then again you could just use a reduced partial pressure of nitrogen.
A pure oxygen room would be a fire hazard too. You would need people living in these areas for extended turns so defiantly a no smoking area.
NAOM
Rather than quibbling about the details, I'll just say that while you've got a lot of options, you can't avoid the fact that humans + pressure changes = complicated and expensive. And that's just one item in my long list.
And while your retired NASA astronaut-turned-mechanic is futzing around with pressure suits, stratospheric elevators and pure oxygen, the ground-bound solar plant repairman is doing his job with a pipe wrench, an ATV, and an associate's degree from his local community college.
Yup
NAOM
With decent attention to design, there should be very little maintenance required. If anything turns out to need regular attention, teleoperation would probably be the choice. At least there are on rats to chew on the insulation, or ants to pack the switch boxes.
Why why oh why have several threads here had one side of the conversation deleted? The questions must have been reasonable because reasonable people are replying reasonably ... what gives?
Or is it just me?
"several threads here had one side of the conversation deleted?"
There was a data base crash.
Yes, my account was one of the ones that vanished, along with everything I'd ever posted. I'm back now.
That's something I've wondered about. Why don't they counter the internal pressure with small ribbons or cables going to spring assemblies on the forearm? The pressure straightens the glove fingers, and the cables would curl them more or less equally. The forearm assemblies would have to be adjustable to allow for a range of differential pressures, possibly even using suit pressure to be self adjusting. I don't see a theoretical problem with this approach, and NASA has a lot of smart guys on staff, so I'm guessing maybe it's the packaging?
Your solution adjusts the balance point so the fingers are partly closed when relaxed. Reduces the force needed to clench your fist, but now you have to strain to *open* your hand. Some sort of compound spring with a variable spring constant might work, or even an electronic force-feedback system, but that's a lot of equipment to pack into a very limited space.
I guess that's why they go for the reduced pressure approach;)
NAOM
EDIT:HTML oopsie
Add to the list:
* deicing equipment so chunks of ice do not break off the cable and fall out of the sky.
* lots of blinking lights to warn aircraft of its location.
* ability to survive lightning strikes.
* protection from static electricity.
* a recovery system in case the solar collector breaks free and floats away.
The airborne OCGT may allow the collector to be self-propelled for recovery purposes.
Good additions.
We never said this was *easy.*
Not to expose dissention in the StratoSolar ranks, but Keith and I are at somewhat opposite ends of the spectrum on the approaches we favor. Keith likes the efficiency and 24-hour power capability of the thermal approach he wrote about here. He isn't intimidated by the scale and technical challenges. I like the (relative) simplicity and lower startup hurdles of a modular PV approach.
Many of the more challenging items in your list have to do with maintenance at altitude. That's not something I think we should even try to support. The approach I favor is "build it on the ground, send it up, it works; if it stops working, haul it down. Diagnose, fix, or replace, and send a functioning module back up."
The chief technical challenge is in devising a reliable means to allow an array of semi-independent modules to link up at altitude and operate as one extended array, without shadowing each other. I'm thinking tether followers and inflated cone-like docking fixtures. But even that capability isn't needed initially. A single module can be an impressive power source.
Keith
I am not sure where you are getting your costings from but they are way out from reality. The cost of a 1GW conventional combined cycle power plant is in excess of $1.3B. Thats before you even start building the fancy stuff.
How do you define, or measure, complexity? There's nothing particularly complex or high tech about an aluminized mylar gas bag. And the simplification of the solar PV arrays by eliminating the need for protection from rain, hail, and accumulation of dust is one of the driving motivations for this approach.
It's two to three the solar exposure of the same collector area in the most favorable areas for ground-based solar -- like the US desert Southwest. But for areas like the U.K. and Germany that are frequently cloudy, it's more like 10x.
But you're right that it all comes down to cost. The Stratosolar approach will succeed or fail based on whether it can deliver power more reliably and more cheaply, over the long term, than competing RE technologies.
A very good question for which I don't know the answer. But somewhere in my glorious imaginary Excel spreadsheet for calculating complexity cost, there's a big checkbox for "Does It Fly?"
Case in point: a Cessna 162 airplane has about the same mass and engine horsepower as a Honda Gold Wing motorcycle. But the Cessna costs about 5 times as much. Why? Lots of reasons, but mostly because it flies.
I suggest the following rule: don't make something airborne unless it totally doesn't work on the ground.
(Also, don't fill it with hydrogen unless you can afford to replace it, but that's a side issue.)
Or more specifically, because it's certified to fly passengers. The plane's price includes a whopping margin to cover company liability. Leave out the passengers and things get a lot more affordable. Ultralights are almost cheap.
I don't see that a tethered, unmanned lighter-than-air platform should incur much of a "because it flies" premium. In the event of a failure, mylar film fluttering from the sky isn't going to hurt anyone. Even the solar panels are so thin and light that there's little threat. Riskiest would be the tether itself. But the systems will have to be confined to "no fly" zones that will tend to be unpopulated or very sparsely populated. Besides, it's easy to sprinkle auto-deploying parachute packages along the upper length of the tether "just in case".
Yes, but the motorcycle has to be certified, liability-insured, and government-rated to carry passengers too. This process costs more for the plane than the bike. Why? Because it flies.
Let's take your example of ultralights. They cost about $20K assembled, and have weight and engine power somewhere between a small ($4K) and a large ($10K) sport motorcycle. So even with minimal safety standards, the Just Because It Flies premium is a factor of 3 or 4.
And nobody, not the government, your investors, or your neighbors, is going to let you fly a gigawatt Hindenburg the size of a small city with the sort of casual safety standards used to build ultralight aircraft.
You're mixing up "just because it flies" and "because it's built in a small fraction of the volume".
Keith,
Some questions:
1) What did you actually use for the diameter of the off-axis parabolic reflector in your calculations? (Or what did you take the diameter of a TV dish antenna to be?)
2) Similarly, what did you use for the inner diameter of the light pipe?
3) What do you mean by the "angular divergence of radiation from the sun"? Surely the radiation comes out in all directions. Is 0.28 deg perhaps what you used for the angular diameter of the Sun's disk as viewed from Earth?
Thanks,
RE 1, 2300 meters, the DISH antenna I glued little mirrors on for a model is .53 meters across.
Re 2, 30 to 50 meters. It depends on the reflectivity of the liner plastic. Given a reflectivity, you work out the light throughput per kg of mirror plus light pipe over a range of light pipe size and take the minimum. Typical case gave 7% loss in the light pipe.
Re 3, yes, the effective diameter of the sun viewed from earth.
The angular diameter of Sun at Earth is approximately .5 degrees. The diameter of the photosphere is not well defined (it depends on the definition) and the Earth moves in an elliptical orbit which makes the size approximate.
Basic Astronomical Data for the Sun
Radius of solar photosphere: 695,508 km
Mean orbital radius of Earth: 149,618,753 km
angular diameter of Sun: 2 * atan(695,508 km / 149,618,753 km) = .53 degrees = 32.0 minutes of arc.
Keith,
I think BT's value of Sun angular diameter is right. How does using 0.53 deg change your proposal? Better or worse?
I'm not sure what the conventional usage is, but while you can use the angular diameter of the source as a measure of optical divergence, that's not quite the figure you need to use in thermodynamic calculations. Or for calculating the average number of bounces in a light pipe. The angular diameter of the source defines the maximum divergence angle for a portion of the light flux, but what you actually have is a distribution of flux vs. divergence angle. For the light pipe, you'll have some portions of the flux that are closely aligned with the pipe and incur very few bounces. Other portions will be near the maximum divergence and will suffer higher attenuation from more bounces. You have to integrate.
I didn't do the calculations myself, but I think 0.28 degrees (or whatever it was) is the effective divergence angle -- the angle that you can pretend all the flux is concentrated at to get the same result you'd get from proper integration. Something like an average, although it may actually be the RMS value.
You are right that the transmission factor, will be a function of the divergence angle. But this pipe will not be a perfect cyllinder, it will be curved, because of wind stress, and it probably has other optical imperfections that will increase the average divergence, possibly very substantially. And reflectivity is probably a function of wavelength as well, so you end up with multiple dimensions to integrate over if you want to do it right. Although with modern computers that shouldn't be a problem.
You're right about the effects of curves caused by wind stress. But the reflectivity, in this case, isn't a function of wavelength.
The reflector is a sheet of transparent plastic, with an embossed set of sawtooth ridges running along its length. In cross section, they're right angle prisms. Light entering the plastic undergoes total internal reflection off the faces of the ridges. That's how the reflectivity can be so high. Total internal reflection really is total! There are very small losses along the peak and bottom lines of the ridges, because they're not perfectly sharp. But the losses are over an order of magnitude less than you'd get from the smoothest and most reflective metallic films.
"The reflector is a sheet of transparent plastic"
A pointer to a data sheet got dropped in editing.
http://www.revelationlighting.co.uk/OLF%20Spec.pdf
Light that hits the film at less than 27.6 deg is totally reflected.
3M makes some really amazing products. Helpful too. They sent us a few meters of 2301 OLF as a sample.
That is way cool. However it raises some other concerns. Sounds like the cost per square meter might be high. Most plastics badly degrade when exposed to perhaps a years worth of groundlevel sunlight, they get brittle and fall apart. Thats why most plastic stuff for outdoor use is black, carbin added to absorb the light before it can penetrate too far. And your light is a thousand times as intense. I presume the lightpipe must last for many years to be economically viable. Then you have the issue of what you use for the gas inside. Any bug that gets in during the day, will be almost instantaneously converted to smoke, and that will precipitae out onto the inside surgace of the lightpipe. That would degrade its performance, but worst, raise the temp of the plastic. Doesn't take much heat to soften/melt plastic
.
You could buy one of those surplus fresnel lenses, for $200 you can get one with roughly the collection area of a meter in diameter. Then you can start playing with high intensity light versus engineered materials.
"Sounds like the cost per square meter might be high."
It's really quite modest over the cost of the polycarbonate plastic, maybe x2 for the amount needed.
"Most plastics badly degrade when exposed to . . . sunlight"
It's the UV that eats them and sunlight at 20 km has a *lot* of UV.
Various thoughts are to have the concentrator mirrors just not reflect UV, and to put additives in the plastic to prevent damage. It's a material problem that has to be solved for the idea to be viable.
"Then you have the issue of what you use for the gas inside"
A flow of dry, highly filtered air is the current default. As you say, bugs would be a problem. A mix of argon and helium with the density of air (or slightly higher) works better, but the lower scattering losses don't seem to be worth the cost. This is not set in stone so more analysis might change the conclusion.
Oh, no. I didn't realize you were using a prism film. Relying on total internal reflection means any significant bends in the light pipe, or any scattering from imperfections on the internal surface, will cause additional light loss. And even before the pipe gets dust inside it, according to your data sheet the film is *made* with imperfections every meter along its length.
Your pipe transmission losses are probably much higher than you've estimated.
I'm trying to understand Keith's proposal and the calculation that he has done to support it. Light pipes are not something for which I have ever seen conventional usage and I venture to a guess that this is also true for Keith. So I ask him for a few details on what he has done, using words that he has used.
For successful use of a light pipe, one must use a parabolic mirror, or a lens to focus an image of the source (in this case, the Sun) on the entrance end of the light pipe. In my opinion, the divergence of the bundle of rays inside the light pipe depends on the f/number of the focusing element, not the angular size of the source. But maybe I am totally confused.
I don't think thermodynamics is involved understanding the optical transport system. If it is I am really confused by this.
"I'm trying to understand Keith's proposal"
First per up thread, this is really Ed Kelly's proposal. Most of my work was on the aerodynamics and force models. I am going to let Ed explain the optics. I could do it with the help of a book I have on non focusing optics, but he can do it better.
The thermodynamic is easy to understand though. You can't focus something to where it is hotter than the source. To do so would violate the second law of heat going uphill.
Very simply, I'm not convinced a collector etc. being 12 miles up would make it much more worth doing. Not that much extra effective flux is there, or is it (more UV, but enough more to be worth the trouble?) Maybe some benefit getting the collection process "out of the way" - ?
That was also my first reaction to the concept. 1.36 kW / m^2 isn't all that much more than 1.0 kW, is it? But the difference turns out to be considerably more than that.
The 1.0 kW / m^2 that you see quoted so often for normal solar irradiance at earth's surface only applies when the sun is high in the sky. When it's not so high, the irradiance is substantially less. That holds even for tracking panels directly facing the sun. Think of it this way: in traversing the atmosphere along the shortest path (i.e., from directly overhead), just over 26% of solar input is lost to atmospheric absorbtion and scattering. When the elevation of the sun is 30 degrees, however, its path to the surface is twice as long as when it's at 90 degrees. As a result, losses are nearly doubled, and irradiance drops to 0.7 kW. When the sun is lower than 30 degrees -- as it is in morning and evening hours and for a large part of the day at temperate latitudes in winter -- the losses are even greater. That's why the "full time equivalent" hours per day of solar irradiance top out around 6 hours average, even for locations that are almost always sunny.
At 20 km altitude, above 95% of the atmosphere, losses are correspondingly reduced. Although the same inverse cosine scaling for losses as a function of solar elevation still apply (twice the losses when the sun is at 30 degrees as when it is overhead), the losses to which it applies are around 95% less to begin with. So there's hardly any difference in irradiance whether the sun is overhead or at 30 degrees or even lower. Losses are so low that the irradiance plot rises close to its full peak value in the first half hour after sunrise. As a result, the "full time equivalent
hours of solar input at 20 km are close to a full half day. When you add in that baseline level of irradiance is 30% higher than at sea level, you find you're at better than 2:1 advantage over even very good ground locations.
If one considers that the reflective area of the 50m x 20km light pipe interior alone is about 75% of the area of the primary collector, using all that reflective area on the ground would just about make up for the 50% solar deficit of ground based collectors.
I agree that high temperature solar storage can be extremely inexpensive at large scales, but this makes the ground based system even more attractive, since cheap storage mitigates ground based weather effects on energy collection.
One added complication you may not have considered: All the energy dissipated inside the light pipe, about 280MW, will end up heating the air inside. How hot it would get, I haven't tried to calculate, but let's assume the the "film" wall will stand the temperature. I assume that the pipe is sealed at the top and vented at the bottom, which would allow pressure equalization during the day. All that hot air would even augment lift. But when the sun goes down, the interior air will cool rapidly. Huge fans would have to reintroduce air into the light pipe in order to maintain enough pressure to prevent the pipe from collapsing. Even a tiny over-pressure from the outside would collapse the pipe.
That's only if it's absorbed by the air (or other gas). If it's absorbed by the reflectors, it'll heat the wall and be able to dissipate heat to the atmosphere.
The idea of using another gas intrigues me. What are the IR absorption and Rayleigh-scattering properties of e.g. hydrogen and ammonia vs. air?
I did a quick "R-value" calculation and found that the temperature inside the light pipe is just a few degrees above ambient if the reflective film is directly in contact with the outside air; more like 30 degrees if the pipe is surrounded by an aerodynamic fairing, which would act as an insulator.
If the pipe is sealed at the top, there'll be an overpressure there as the air inside heats up. To minimize this, you may prefer to have the pipe open at top and bottom, so air can circulate freely from bottom to top.
But then you have another problem: unless you remove all water vapor from the incoming air, you'll get *clouds* inside the light pipe.
Hoboy.
I was planning to ask similar questions.
The light intensity within, and below the pipe is quite high. It wouldn't take much of a dirty spot on the pipes inside to burn through the wall. And being highly reflective, the thermal radiative efficency of the lightpipe material is likely be quite low. So heating of the lightpipe, interior gas, as well as the wall, is an issue that needs to be considered. Then if we do end up with inside bounancy being different from the outside air, the rate of pressure change with altitude will be different inside/outside. I think this would create a very powerful chimney effect. Incodentally, if you can build very tall cyllinders, then you can use the pipe as a solar chimney, and for chimneys in the multi-kilometer range, thermal efficiency can be quite good.
I'll cover safety concerns in another comment.
I see no need for a pipe. Collect and collimate a lot (megawatts) of light energy and send it from one side of the atmosphere to the other. Don't need no stinking pipe for that. If its thermal energy you want at the target, stick to the atmospheric windows that are best for the wavelengths of interest. What is really keen here is that instead of the typical beam control problem of trying to figure out what is happening at the target and compensate for it in real time, you get to put all the sensors you want at the target (ground) to control your high altitude DMs and SMs. You will still have balloons and tethers, sorry I don't do those.
That is a nice death-ray you are doing there. Well, ok, the original idea was already a death-ray, but at least it was inside a tube...
Now I'm thinking on joining the NIMBY crowd.
I think that the light that is not reflected won't dissipate as heat, rather it will mostly scatter and leave the reflector. It should be possible to capture that light with photovoltaics around the outside of the light pipe. It won't be as efficient as the thermal conversion with the turbines at the bottom, but it won't be zero either.
I think that once you capture the light leaking from the light pipe with photovoltaics, that relaxes the constraint on light pipe diameter and reflectivity some, which lowers the diameter and reduces the wind loadings.
PV panels around the light pipe adds a lot of complexity and cost without much gain. Work out the math: mo matter how leaky the light pipe is, you'd collect more energy by just putting those photovoltaics in a solar farm on the ground across the street.
This is an interesting idea, but in addition to the other problems mentioned, the problem I see is the ability of the light pipe and steel anchoring cable to support their own weight. For example, if the light pipe weighs just 1 ounce per inch, by the time you reach 12 miles in altitude, the weight of the pipe is about 47,500 pounds. I don't know of a substance that is that light but which would not break under that much weight. When you add the weight of a steel anchoring cable, then the total weight reaches many many tons. It doesn't matter how much lift you have in the balloons, if the cable can't support it's own weight, it won't fly.
Not a problem, Monsanto has a special division where they have set up a giant farm where they raise genetically modified orb weavers and they take the silk they produce and make ultra lightweight high tensile strength cables. The only problem is the spiders are really large and have a predilection for human flesh...
Thanks, Fred, for speaking my mind on this kinda stuff. Saves work.
My own little game at the moment is home grown energy via biogas, CHP, gasifiers, and the like as promoted just now on Energy Bulletin by Warren Weisman. I am also getting enthusiastic about a very little free piston stirling that only pumps gas over a heater-turbine-rotary alternator. looks highly feasible at the moment. This works because the stirling's piston compressor is much more efficient than a tiny rotary brayton compressor at the 1kW power level. This thought came to me by way of Engineer-Poet's advocacy of a way to use nuclear heat a few days back.
If it works, might save us from 'em spiders.
Speaking of Stirling engines, are you familiar with Bill Gross from IdeaLabs' work with setting up tracking systems for a solar farm with stirling engines. They have adapted that tracking system at eSolar, another IdeaLab company.
http://www.idealab.com/inside_the_lab/articles/esolar_solar_rising.html
I have always liked bill Gross' tracking heliostats. Like my electronics sons keep telling me, logic costs nothing, use it. So a swarm of smart heliostats could do a lot of things in a city, plastered all over the walls of the bldgs. You could buy time on them, and cook your supper standing in the window, or lunch, anyhow.
I think the idea is to disperse gasbags throughout the length of the light pipe, so it floats on its own. The problem is that this adds to the aerodynamic loads on the pipe, and the complexity of adding sensors, hydrogen resupply lines and fire suppression systems along the entire length of the beanstalk.
Polymer cables like Spectra have no problem when you concentrate all the buoyancy at the top.
Steel cables, you really need to support them with buoyant aerodynamic shrouds so that you don't get high growth in the cable mass as it goes up.
Hmm. I have never tried this before, but Google docs claims I can share this openly. Try here:
https://docs.google.com/spreadsheet/ccc?key=0ApiotdmmTJQsdDV2VkpfTlJlSS1...
StratoSolar's website estimates that electricity from this technology will cost 10c per kwh, which they claim is about the same as wind?! Actually, onshore wind is around 6c per kwh, with a number of companies promosing 4c per kwh over the next few years.
I don't see the business model here. Cheap storage with ice can even out the peaks from air conditioning in hot climates.
Perhaps with a large campaign contribution, you could get it funded by DOE. Talk with George Kaiser.
Signed,
Horrified
Disclaimer: I'm a co-founder in a wind company.
Here are the correct urls for my posting
ice storage
http://www.ice-energy.com/
doe funding path
http://dailycaller.com/2011/09/01/bankrupt-solar-company-with-fed-backin...
Based on a small multiple of the cost of common materials, electricity from the tethered CSP plants could be as low as 1.5 cents per kWh when mature.
And I heard that Fission power would be such a great deal it would be to cheap to meter. Why should this claim of your be believed?
But lets move onto:
As noted in the introduction, the buoyancy needed to keep the collector at 20,000 meters would be provided by metalized plastic bags filled with Hydrogen inside the structure.
Let me get this strait - the idea is to introduce Hydrogen into the upper atmosphere. Where there is highly reactive Ozone.
Hrmmm, reactive H2 and reactive O3.
Sacrificing O3 for a light-pipe dream, what a plan!
Lets not forget the long view - removal of Water from the planet while increasing the O2 and the H2 dissipates to space.
Now that you cited O3... It tends to react with metals and organics too. What will hold the H2 again?
Keith,
Would it be practical to consider designing the cross-section of the tether cable to be teardrop shape instead of round with the capability of rotation so that it would reduce the wind sheer?
Alternatively, would it possible to make the tether thicker in cross-section but design it with a "honey-comb" of holes to allow the wind to "pass through" the tether and reduce wind sheer?
I am still concerned about aircraft impact. I don't see any high confidence method of avoiding an airplane impact. Since such an event would destroy the aircraft and probably destroy the billion dollar power plant, it is a big risk. Sky scrapers at least can be designed to withstand aircraft impact and few of them are higher than 2000 feet. However, your design crosses all potential commercial aircraft flight levels. I.e. they cannot avoid it by altitude.
Good luck with your work. I still like Cold Fusion (if it can be made to work!). Great post.
Iwylie
"the cross-section of the tether cable to be teardrop shape instead of round with the capability of rotation so that it would reduce the wind sheer? "
That's exactly what we proposed.
Your concerns about aircraft are exactly the same as mine.
However, the FAA people we have talked to seem to think they can establish no fly zones were the power plants are.
And the pilots seem to favor low cost synthetic jet fuel over the risk of flying into one of them.
I prefer solar power satellite, but will take any solution that provides enough cheap energy to avoid world wide starvation.
Keith,
I did a very rough calculation on the mass of a 16in steel cable that is 20 km long that would have to suspended by the hydrogen balloon. Even assuming that all the mass of the balloon displaced the air 100% (i.e. a vacuum), I get that the cable would be 20,000 tonnes and that the volume of the balloon would have to be at least (at 0.012 kg/m3) 20,000,000 kg/0.012 kg/m3 = 1.7 e9 m3 = 1.7 cubic kilometers.
Please correct me if I am wrong.
Pretty big balloon!
Iwylie
"I get that the cable would be 20,000 tonnes"
Close. The model run I put up through Google gives almost 30,000 tonnes for the steel around the light pipe.
That's a *lot* of steel if you want to take it home, but not very much for a ship. The battleship Texas was built 100 years ago and has about that much steel in it.
"Pretty big balloon!"
Yeah. The buoyant shrouds hold up the cable as it goes, with the excess buoyancy in the top 5 km.
These things are HUGE, like 25 times the area of the Pentagon. Only reason I didn't entirely boggle is that power satellites are even bigger. But those are built in zero g.
But, Keith, we know that the Space Based Solar Power (SBSP) satellites have not been built and likely will never be build for valid technical reasons, such as that large size you mention...
E. Swanson
True no power satellites have been built, but the have been analyzed for the more than 40 years. Near as I can see there are no technical reasons they could not be built, including the large size. If you know otherwise, let me know. It's economic problems, mainly the $100 B investment in infrastructure to get the lift cost to GEO down $100/kg that holds them up.
They are even bigger than the CSP StartoSolar concept, which is why the size didn't bother.
Surely you are joking, right? Size is a technical "problem" which hasn't been overcome. Building an extremely accurate transmitting antenna is part of that problem, as is station keeping due to solar wind. Also, there is the need for large radiators to cool the electronics.
Cost is always part of the engineering problem as well and the exceptionally large cost for launch to GEO would likely kill any such project. If cost were no problem, we could build cities completely covered with transparent domes, avoiding weather issues and heating/cooling problems as well, but only the sci-fi guys from the '50s considered that. We could simply build roofs over all the existing interstate highways, installing PV cells on them, and add PV to every roof in the US, but "economics" appears to be the problem. Didn't you understand anything we discussed the last time around?
E. Swanson
"Building an extremely accurate transmitting antenna"
I found a design for large space structures that are actively controlled using laser beams to make them flat. But a microwave engineer I ran into at a conference said the problem was even less because you can just phase lock the transmitters to an incoming beam (the original suggestion some 30 years ago) and flatness is not a concern.
If you go with thermal cycle power satellites, the big problem is getting rid of the waste heat from the steam or super critical CO2 turbines. Takes square km to do the job but Eric Drexler figured out how to to it long ago. July and August here: http://www.nss.org/settlement/L5news/L5news1979.htm
Cost to GEO was the subject of the last article I wrote. It either gets down to $100/kg (for 5 kg/kW) or the whole thing doesn't make sense.
The target of 1-2 cents per kWh is where you can make synthetic transport fuels for affordable prices.
Did Eric Drexler actually test his idea in zero gravity? What about the problem of moving the 2 phase gas/solid mixture thru the fans or pumps? Drexler claimed that erosion from the solids moving within the tubes of the radiators was a minor problem, but I think the higher velocities thru the fans or pumps would present a serious erosion problem. The mass flow rates would be rather high, as the heat capacity of the projected solid materials is going to be less than that for water, everybody's favorite heat transfer fluid here on Earth. Don't forget that a failure in the cooling loop would likely fry the electronics and a thermal cycle without cooling would similarly destruct, since the incoming solar energy could not be switched off quickly.
But, my big objection was to the placement of the radiators for the electronics driving the antenna. Given the relative motion of the antenna and the collector surface, the heat transfer fluid would need to pass thru a rotating coupling in both directions in order to keep the radiating surface facing parallel to the Sun-Earth vector. Recall, if you will, the problems with the rotating couplings on the ISS. Now, throw some dust into the bearings and seals...
E. Swanson
"But, my big objection was to the placement of the radiators for the electronics driving the antenna."
Oh. Transmitter input is 10 GW, output is 8.5 so waste heat is 1.5 GW.
Area of a 1 km disk is 0.785 km^2 or 1.57km^2 for both sides. So the waste heat is a little under a kW/m^2. The transmitter surface would radiate thermally a kW/m^2 at 95 deg C.
That's well inside the operating temperature of the transmitting tubes.
Are you planning to supply DC current from PV's to transmitters evenly distributed across the surface of the antenna, one for each element? How much copper would this require? Or, are you thinking of spacing transmitters with some distance between, each driving several antenna elements? If the latter situation is your design, then the thermal energy to be removed becomes more concentrated and the temperature increases. Worse, there would be times during each day when the black radiators would face the Sun and absorb another 1,365 w/m^2, thus the temperature would increase beyond that of your simple black body calculation...
E. Swanson
True no power satellites have been built, but the have been analyzed for the more than 40 years. Near as I can see there are no technical reasons they could not be built
And there are no technical reasons why fission based electrical power won't work correctly or within the rules set up by Man, yet something as simple as sleeping security guards are ignored and covered up.
You wouldn't use steel, you would use something like spectra or kevlar which is UV resistant.
Also, you wouldn't tether it with a single cable. Distributing the load across multiple cables not anchored at the base makes more sense to me. Then wind loads can be resisted by direct tension in the support cables and you don't need such high buoyancy.
If you put the support stations 20 km from the base, then the cables go up at ~45 degrees. You could hang stuff on them to do local weather modification, updraft and downdraft to simulate mountains and produce local rain.
Kevlar is very UV sensitive.
NAOM
I believe the buoyancy of hydrogen in air at 20 km is more like 0.08 kg/m^3. That's just what I recall off the top of my head, however. Could be wrong. I'll have to check.
Your number is correct.
A honeycomb of holes would turn it into a flute whistling in the wind. Noise pollution.
Whats the point of all of these techno wonder projects if the collapse of industrial civilization is imminent told by the likes of Mike Ruppert and others. I thought there is no solution to peak oil other than the death of billions in the coming dieoff where supposedly the survivors will live within the limits of nature?
Are you looking to be convinced otherwise, or just pushing doomer porn?
If Ruppert is correct, nothing you do will make a difference. That includes posting here. Stop wasting your effort and enjoy your life.
Needless to say, I do not think Ruppert is right. Yet.
Well said, EP.
Ok. Now for the inevitable safety issues, that might make licensing almost impossible. The light coming out the bottom of your lightpipe is quite intense. If rather than using it as a power plant, you do the following thought experiment. Dethether it, and have some mechanism of controlling where it flys over. Instant terror weapon. 1400C, that means nearly anything below will be instant toast. Anything with any organic or carbon content will almost instantly burst into flame. Rocks, concrete, metals will melt! Heck its not much different -excepting blast effects and ionizing radiation as being under ground zero of an A-bomb. So activists will raise the spector of this thing getting out of control, and drifting across the landscape, frying anything underneath.
If the light pipe is open at the top, then if the bottom breaks free, the gas inside the light pipe will rise and generate a pressure drop at the bottom. This will have the effect of closing off the bottom by collapsing it, shutting off any light escape from the bottom.
You're exaggerating a bit. It's not a coherent beam, so it's got no range. After so many bounces in the light pipe, the light will come out the bottom of the light pipe radially in all directions -- it's like a very bright light bulb rather than a laser beam, and subject to the inverse square law.
Right at the mouth of the pipe, the energy flux is 1.5 megawatts/m2, enough to melt almost anything. But 500 meters away, it's more like 2 kilowatts/m2, which is blinding but won't cook ya. The output is only lethal if the light pipe drifts right over your house.
In any case, a few hundred meters of detonating cord wrapped around the top of the light pipe solves this problem nicely.
"Dethether it, and have some mechanism of controlling where it flys over"
Ah, yeah. It takes 300 million newtons to hold it down. That would take a vehicle a good deal more ten times as massive than NASA's crawler-transporter, say 50,000 tonnes, or around the same mass as an Iowa class battleship.
Forget the light energy, just drive this puppy over yer target.
Use it to carry and drop anvils. The target-camera footage can be recycled as cartoons for the next 3 generations.
Lets talk about the least controversial part of this design, the part that is less than 200 feet above ground level.
A 1 GW combined cycle gas plant costs about $1.5 Billion.
The proposed soar plant needs everything except the gas supply system, so lets say $1.3 billion. Now add the cost of the solar/air heat exchanger, the heat storage facility, the light pipe foundation, facilities to control and maintain the flying portion of the plant.
Also the turbines will have to be somewhat oversized to compensate for the decreasing temperature of the hot air supply overnight.
I estimate that the ground portion of this project, the least risky part, will cost about $2 billion, more than 4 times the cost estimate in this proposal.
I expect a factory mass produced modular molten salt reactor designed for a 40-60 year life will cost less than the ground based part of this design if we build at least 100 units.
$1.5 B sounds more like what I've seen bandied about for a new 1 GW coal-fired plant. Gas turbines, I believe, are usually quoted at more like $400 M per GW without CC, or $600 M with CC. Those numbers might or might not be correct, but it's really beside the point.
I won't dispute what you say about the potential for factory-produced modular MSRs. If we could get past the anti-nuclear sentiment, the regulator hurdles, and the lead time needed to develop and prove out new nuclear fuel cycles, I suspect that factory-produced modular MSRs would be the way to go.
But that's a big IF, and we're not looking at a mandated national program that requires an up-front either / or decision. We're talking private equity. Within that domain, there's plenty of room to explore multiple paths, and see what works out.
A 1 GW combined cycle gas plant costs about $1.5 Billion.
See table 2.
978 million per GW.
But I have seen lower prices. I don't know how much of what is in the number above includes expenses we already figured in.
Forgive me if this was already mentioned but why not go another seemingly much cheaper and less complicated route for making electricity.I have only read about it but it seems very plausible,that is the creation and use of high altitude wind kites and turbines.There are several ideas and models already being experimented with on small scales.From one website:
"At any moment, the winds in high-altitude jet streams hold roughly 100 times more energy than all the electricity being consumed on Earth"
It certainly would seem cost effective to construct models and products for use with the high altitude winds.Simply Google this for plenty of information.
Some examples:
http://www.kitegen.com/en/
http://www.magenn.com/
Now this explains where the expression "pie in the sky" comes from.
Joke aside, I don't know of any heavy high stratosphere objects being successfully put in place, let alone multithousand tonne ones. And you know that the light cable that's supposed to withstand winds of hundreds kms per hour is simply not going to fly.
I have a suggestion - scrap this idea, there are much better ones in that direction. IIRC Russia was almost successful in lunching a mirror in orbit which focuses sunlight on a certain area on Earth. Imagine scaling this up and placing thermal collectors (or even PV) on that area (should be in desert with rare clouding). This would provide a perfect 24/7 baseload solution and is achievable without any engineering breakthroughs.
About that steel cable
(8 inches) times (8 inches) times pi times (20 kilometers) = 2 594.34229 m^3
That's over 20 kilotons of steel.
Oh c'mon now! This concept is a discussion which is surely being conducted whilst reclining on deck chairs on a sinking ocean liner!
Occam's Razor says Use the simplest approach which will achieve the required outcome.
The required outcome is some energy output. Any will do. Concentrated Solar at ground level does a fine job that is only marginally less efficient than the same at 20km altitude. But the Flying Thing is so utterly complex, so far beyond our actual technological reach as to be at the other extreme.
20,000 tonnes of cable, and another 20,000 tonnes or so of other infrastructure. It will never fly!
Next!
Any chance of using optical fibre instead of a light pipe? These can carry 2MW/cm^2 with little loss. Might be a problem concentrating the light at the reflector though.
There is more than enough energy reaching the ground. Dont bother flying just to increase the harvest.
The effort would be better focused on finding ways to have more efficient and low cost PV panels.
I think what the O.P. is going after is reliability. Ground based PV is affected by weather (clouds), and the winter/summer ration can be problematic in non-tropical latitudes. This proposal, coupled with thermal storage satisfies the requirements for baseline power. groundbased PV, is for cheaper, but time and weather variable power.
I just hope the obvious practical problems here don't detract from similar, sensible suggestions. Wind power generation by kite looked a lot more realistic.
People of a certain unhelpful mindset like to conflate bad concepts with good ones. For example "climate change" debates derail easily when people start talking about temperature, which is a horribly inappropriate metric to focus on.
Other tethered technologies could work well.
Other solar technologies could work well.
This? I'll believe it when I see it. So far we've never got twenty kilotons of ANYTHING to fly.
"twenty kilotons of ANYTHING to fly"
These things float rather than fly. I am not sure what the record is for a floating object, perhaps a million tons.
No, you don't get to count floating in *water*. I'll let you argue that it's fundamentally the same thing if you'll go outside and swim to your neighbor's rooftop first.
The largest lighter-than-air vehicle by far was the Hindenburg, with a mass of 0.2 kilotons.
I think the big problem is the big leap needed. Both size, and the need to be up at 20KM. Maybe a much smaller less ambitious project to demonstrate airborne collectors that avoid much of the clouds. The central valley of California is problematic for year round solar energy, because it is usually socked in by fog/low clouds during the winter. Get up to 500 to a thousand meters, and most winter days have brillant sunshine. Maybe you can find some major compromise pilot project, that demonstrates that you can get above eighty percent of the clouds, at reasonable cost?
I agree, leap size is what sticks out here. The proposal, if it happens will be
* the largest airborne object ever
* the first construction of a light pipe on this scale
* the first time we've built anything this tall
* the first time we've had to contend with these weather effects (buildings and aeroplanes have different problems and solutions)
* the first time all these technologies (many still theoretical) have been integrated
* the first time we've had to deliver repairs to anything in this area
...and so on and so on. It beaks too many records -- it's too risky to be palletable -- and there *IS* no half-sized trial version. What have the investment bankers said so far?
What happens if the light pipe snaps (steel cables snap too) and it all floats away? What happens if the top balloon tears and it all falls down? Good luck getting insurance for that!
Ok so maybe governments could afford the investment and insurance. They're going to wonder what ELSE they could get for their money. Opportunity cost. I'll be charitable and say the technology is going to work, it's going to cost politicians their jobs trying to prove that it is.
"beaks too many records -"
Hey, I came at this project from SBSP. That's a far larger project and would cost 50 times the cost of a StratoSolar plant just to set up low cost transportation. Your objections are psychological/sociological. I was mostly trying to see if there were physics reason it could not be done.
"- it's too risky to be palletable"
Eventually governments are going to be pushed into a corner. It's nuclear, SBSP or *maybe* StratoSolar. Nothing else I know about scales large enough or gets down into the cost range you need to feed the existing population. Investment bankers who have talked to us suggest government funding is the most likely source right now.
"steel cables snap too"
Well, yes they do. When was the last time a suspension bridge cable failed? That's what the safety factor of two is doing in the spreadsheet model.
Given the downside risk of not solving the energy problem and the upside of low cost energy, it would seem to me that spending ten million on engineering studies or so would be a prudent investment. As you probably know, the government wrote off half a billion last week on a failed solar panel plant.
"Your objections are psychological/sociological."
Yes, exactly. The investment is a political one. Non-technical problems tend to dominate.
"Eventually governments are going to be pushed into a corner."
Historically, when this happens, you get war. Some politicians will calculate cost vs expected returns of invading Iran against projects such as this.
It's not enough to show that the physics is sound, you have to show it's more competetive than other investments.
"Non-technical problems tend to dominate."
Not to engineers.
"Historically, when this happens, you get war."
I am aware of this. H. Keith Henson: Evolutionary Psychology, Memes and the Origin of War.
http://www.mankindquarterly.org/summer2006_henson.html
http://www.kuro5hin.org/story/2006/4/17/194059/296
"Some politicians will calculate cost vs expected returns of invading Iran against projects such as this."
I doubt it. Wishful, not rational, thinking dominates people in "war mode." For what the US blew in Iraq alone we could have had power satellites and StratoSolar ten times over.
"It's not enough to show that the physics is sound, you have to show it's more competetive than other investments."
Like war?
"Not to engineers."
Engineers don't make the decisions.
"For what the US blew in Iraq alone..."
Yet to the decision-makers, it seemed like a good idea at the time.
I know it's difficult and unpleasant to see the world through the eyes of people who are stupider and more powerful and corrupt than you, but it's something you must do if you want to be realistic about actually building this thing.
This project is theoretically very interesting, and very clever, you get credit for that, but it isn't enough. It has to be more attractive than the alternatives. We are engineering solutions for a resource-constrained world.
"Engineers don't make the decisions."
They don't in the US, but in China 19 of the top 20 people have engineering type backgrounds.
Even here, the concepts of what can be done come from engineers.
"realistic about actually building this thing."
I can't say I expect the US to do either of the big energy projects I have been working on.
"more attractive than the alternatives"
Failing to solve the energy/carbon problems is not very attractive at all, though from the more rabid posters on The Oil Drum, you would think they find starvation and war attractive.
"We are engineering solutions for a resource-constrained world."
It's always been "a resource-constrained world."
What are your engineering solutions?
"19 of the top 20 people have engineering type backgrounds"
That's good news, but not decisive. If you have any such figures for the middle managers I'd love to see them!
"Failing to solve the energy/carbon problems is not very attractive at all, though from the more rabid posters on The Oil Drum, you would think they find starvation and war attractive."
Our leaders are either in denial or are keeping their solutions secret. And I think they find war more attractive than sensible alternatives. Recent history speaks volumes.
"What are your engineering solutions?"
The only solution I can see is to use less energy. That's not an engineering solution, so perhaps you're not interested in it.
All this back-and-forth is detracting from the main point I'm trying to make, which is pretty simple:
Unless and until a demonstration model is built, any sensible investor will go into other technologies first -- not because they are superior, but because they are KNOWN to work.
"Unless and until a demonstration model is built, any sensible investor will go into other technologies first -- not because they are superior, but because they are KNOWN to work."
For the people who want to invest in solar energy, can you point out which technologies they should invest in? I am not aware of any that make a profit producing electric power without subsidies that seem rather unlikely to continue over the multiple decade life of power sources.
I am reminded of how Intel used to do processors. One generation they would take process risk (finer design rules, other semi-fab tweaks etc.), the next generation they would dink with the architecture (how you put the transistors together to make a computer). They never did both together, that was deemed too much of a risk.
Well for starter he could put a ballon 12 or 15 miles up in the air and try to keep it here as long as he can, just to have empirical data on the conditions up here with an hydrogen ballon. Or trying to move it up gradually.
Try a small one first and get bigger until you can say you've put a 200 meter thick hydrogen ballon at 12 or 15 miles up in the air and nothing can put it down.
That is a million sized investment, instead of billions, and is required for the final projet. So you can start with that.
Just trying to send a wire 12 miles up, is a feat. Do it the way you want and make it last a year. After we'll see were we'll be.
This is a good idea. If you use an inert working fluid like He or H2, you can go to much higher temperatures in your turbine. You don't need particles to absorb the light, you can use wire or extended surface darkened metal for H2 or carbon for He. If you go to 2000 C, you can get real high thermodynamic efficiency. I would use quartz windows with thermally stratified gas to keep the window cool.
The problem with this is that it does obsolete coal fired power plants. By doing that it makes the value of existing coal reserves go down and will reduce the valuation of companies that own those existing coal reserves. They will push back to prevent this from ever being tested.
I saw an article which explains why fossil fuel interests are so intent on casting doubt on AGW.
http://www.energybulletin.net/stories/2011-07-14/unburnable-carbon-%E2%8...
The problem is that the fossil fuel assets still in the ground have a “value” that is predicated on their use as fuel. If they can't be burned as fuel, for what ever reason, then their value goes down. When a major asset of a fossil fuel company is the value of its fossil fuel reserves, a decline in the value of those reserves means a decline in the value of the company.
The value of coal still in the ground is the major asset that coal companies have. They can (and have) borrowed money (i.e. sold stock) based on the value and projected value of that asset. If that asset goes to zero, then the lenders will require more margin to back the loans. The carbon fuel industry is unsustainable because all the reserves can't be consumed at prices they are currently valued at because it would destroy the environment. When the stock market realizes that, the carbon fuel industry bubble will collapse.
Japan is likely the best place to try this. They need the power, don't have the space and don't have coal/oil/gas interests to block it. Nuclear interests might try to block it, but they don't have the resources in Japan to do so now.
Japan has some of the strongest jet stream winds in the world. The American airforce discovered this during WW2 when they tried high altitude bombing. They also get hit by several typhoons every year. Seems like a challenging weather environment to try it.
The lightpipe plus conventional powerplant makes the entire idea implausible; the cost of the plant consumes most of your cash while the lightpipe is beyond near term technology to be both practical and cost effective.
Focusing on solar PV allows you to build much smaller units that still supply a useful amount of electrical energy, these might be particularly useful for off grid locations like mines, oil platforms or military installations (providing both energy and a high platform for surveillance and communications). Solar PV also allows the ability to scale as technology evolves, bigger platforms, more efficient solar cells or even ultracapacitor energy storage on the ground for 24/7 operation can all be added incrementally.
Some other thoughts:
The aerostat should be aerodynamic, perhaps the "V" shape of JP Aerospace proposed high altitude airships is a good starting point so we don't have to depend on articulating mechanisms to deal with wind events. The hydrogen cells should be in the form of "over pressure balloons" which have been studied for missions to Mars and should hold hydrogen without leaking for periods measured in years. Secondary aerostats should be attached to the tether(s) at altitudes corresponding to light and civil aviation air corridors. These would carry radar reflectors, transponders and other warning devices to minimize the possibility of collisions, as well as relieve some of the stress on the overall tethers. Finally, has anyone considered the use of tethers made of synthetic materials like Spectra or M5 which are far stronger and lighter than steel? M5 in particular is resistant to UV radiation, and should not degrade over the life of the project.
The basic thinking behind this needs to be broadened to encompass different solutions and potential markets, but there is a lot of potential here.
I believe that such expensive new technologies will come into existence only if and as the various supporting technologies that are involved mature commercially on thier own.Everything I can think of which is very expensive to build but neverthe less being built IS getting built as a result of the builder's being able to take advantage of previous technical advances.
Galileo might have built an airplane had the IC engine been invented prior to his time.Henry Ford did not have to invent the engine before building his first car.
The builders of early airplanes were able to incorporate the rapid advances in engine designs made by automotive and industrial engine builders into THIER airplane engines-just as the builders of automotive emgines were able to incorporate the progress being made in metallurgy, machine tools, etc, into thier work.
Advanced computers are available today for the most part simply because primitive computers were cheap enough to build and yet useful enough that they could be built and sold profitably ,enabling the enabling exponential growth of the programming and fabrication industries which have put powerful computers at our fingertips.
We might love to bitch about Windows, but if there were no Windows, the computer industry might be decades behind it's current state of development.Bill Gates put computers in the hands of the public far faster and more efficiently than would have likely been the case had we had more choices, thereby throwing a steady stream of gasoline on the industry browing like wildfire.
(The paradox is that in the end, the growth made possible by MS will be the very thing that destroys MS eventually-The millions of dissatisfied users will create , have already created, better systems.Had there not been SO MANY of them, progress would have been, and bev , much slower.)
Unfortunately I don't see any likely business scenarios which would enable the proposed high altitude solar collecters being built in the near future.
Perhaps if Old Man Bau were to remain hale and hearty for a few more decades, the various supporting technologies( such as the manufacture of super strong,superlight materials suitable for building such high altitude collectors) just might become three d commercial realites.
Old Man Bau is not exactly hale and hearty,although he will probably live for a while yet-perhaps even for a few more decades.
Political and economic reality , not technical considerations, ALMOST certainly preclude the building of such collecters-barring " miracle" break throughs in the materials sciences.
Otoh, I am old enough to have known people( dead for many years now) who took the first airplane they saw as a material manifestation of God, and I can personally remember advent of SEVERAL technologies once dismissed as being either technically or economically infeasible.
"barring " miracle" break throughs in the materials sciences"
What part of this design takes materials we don't already have? It uses steel wire, aluminum, and plastics.
Steel wire has been used for suspension bridges for well over a hundred years.
Aluminum has been used since the 1930s and is produced at 30 million tons per year.
The skin plastic has been used for stadium roofs for more than 40 years, the Denver Airport has one.
Even the reflective plastic liner of the light pipe is more than ten years old.
I was personally skeptical that 50 the meter spans of the primary concentrator could be seamed up from 2 meter stock, but the German company that makes the stock and seaming machines says "no problem," they do it all the time for large roof spans.
I might be overlooking something, but I can't think of any material problems in these things.
Perhaps I should have stated my concerns somewhat differently-maybe steel, aluminum, and currently availavle plastics will work-if it is possible to actually manage these materials in such large quantities , meaning not only the dead wieght, but also the large surface areas involved, while suspended many thousands of feet up in the air, by means of ballons filled with hydrogen.
Apparently there is nothing else on the drawing boards to supply the lift.
I doubt if it would have been possible for the shipbuilders of the nineteenth century to build a WWII battleship, even though they would have had a pretty good idea how to go about the job, from both a theoritical and practical point of view.
The catch is that they were ONLY ABLE to do it after developing the technology and techniques piecemeal but steadily over another century-during that time they learned how to fabricate the components and manage the joining of them together.
They simply could never have succeeded in joing from a civil war era iron clad to a fifty thousand ton warship in a single giant step-they gap between thier capabilities and thier goal would have proven too wide in terms of money, talent, and time.The fact that they could build incrementally larger ships over that century, at prices they could be sold, explains why WWII type ships could be built
Now if on the other hand it were to turn out that you could build your stratospheric collecter with carbon fiber cables, etc....you could probably get by with much smaller and more tractable (so far mostly nonexistent) hoisting machinery.
Maybe a market for such hoisting machinery will develop-perhaps the first generation will have to lift only ten percent of the wieght to one fourth of the altitude.
If the technological /engineering/financial chasm must be crossed at a single bound, Old Man Bau simply isn't up to the job anymore.
Despite my pessimism in respect to the likelihood of these things getting built, I want to say that I found the article informative, entertaining, and intellectually broadening, in the sense that travel is said to be broadening.Well done!
It is imperative that all possible avenues of energy production and conservation be explored and thoroughly discussed-if for no other reason than to learn to distinguish the possible from the impossible, and the affordable from the unaffordable.