A Nation-Sized Battery
Posted by Rembrandt on August 10, 2011 - 11:47am
This is a guest post by Tom Murphy. Tom is an associate professor of physics at the University of California, San Diego.
As we look to transition away from fossil fuels, solar and wind are attractive options. Key factors making them compelling are: the inexhaustibility of the source with use (i.e., renewable); their low carbon footprint; and the independence that small-scale distribution can foster (I’ll never put a nuclear plant on my roof, even if it would make me the coolest physicist ever!).
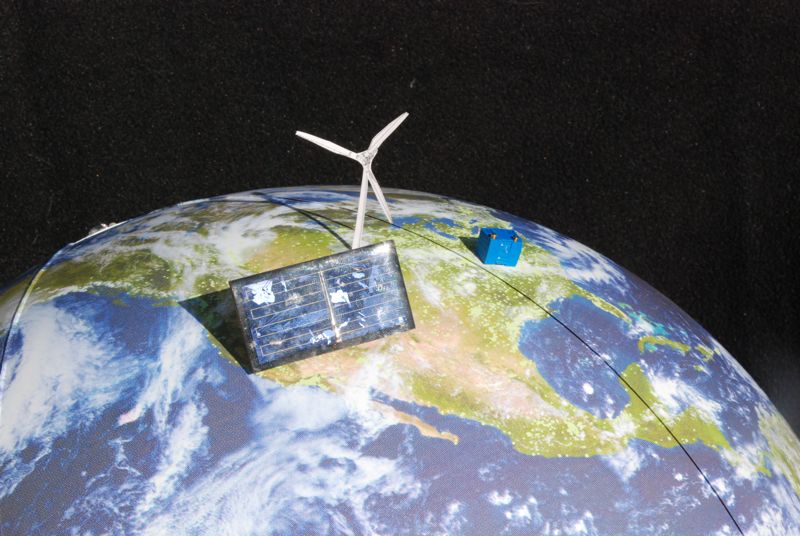
But solar and wind suffer a serious problem in that they are not always available. There are windless days, there are sunless nights, and worst of all, there are windless nights. Obviously, this calls for energy storage, allowing us to collect the energy when we can, and use it when we want.
Small-scale off-grid solar and wind installations have been doing this for a long time, typically using lead-acid batteries as the storage medium. I myself have four golf-cart batteries in my garage storing the energy from eight 130 W solar panels, and use these to power the majority of my electricity consumption at home.
It’s worth pausing to appreciate this fact. Compare this scheme to the dream source of fusion. Why do people go ga-ga over fusion? Because there is enough deuterium in water (sea water is fine) to provide a seemingly inexhaustible source of energy, and there are no atmospheric emissions in the process. Meanwhile, solar provides a source that will last longer (billions of years), produces even less pollution (no radioactive contamination of containment vessel), and is here today! It’s even affordable enough and low-tech enough to be on my roof and in my garage! People—we have arrived!
Storage works on the small scale, as many stand-aloners can attest. How would it scale up? Can it?
Meeting Requirements
So what would it take? We’re not a nation tolerant of power outages. Those big refrigerators can spoil a lot of food when the electricity drops away. A rule of thumb for remote solar installations is that you should design your storage to last for a minimum of three days with no energy input. Even then, sometimes you will “go dark” in the worst storm of the winter. This does not mean literally three days of total deprivation, but could be four consecutive days at 25% average input, so that you only haul in one day’s worth over a four day period, leaving yourself short by three.
So let’s buy ourselves security and design a battery that can last a week without any new inputs (as before, this is not literally 7 days of zero input, but could be 8 days at 12.5% average input or 10 days at 30% input). This may be able to manage the worst-case “perfect” storm of persistent clouds in the desert Southwest plus weak wind in the Plains.
Let’s also plan ahead and have all of our country’s energy needs met by this system: transportation, heating, industry, etc. The rate at which we currently use energy in all forms in the U.S. is 3 TW. If we transition everything to electricity, we can get by with 2 TW, assuming no growth in demand. Why? Because we currently use two-thirds of our energy supply (or 2 TW) to run heat engines, getting only about 0.6 TW out for useful purposes in the bargain. An electrical system could deliver this same 0.6 TW for only 1 TW of input, considering storage and transmission efficiencies.
Running a 2 TW electrified country for 7 days requires 336 billion kWh of storage. We could also use nuclear power as a baseload to offset a significant portion of the need for storage—perhaps chopping the need in two. This post deals with the narrower topic of what it would take to implement a full-scale renewable-energy battery. Scale the result as you see fit.
Lead-Acid Delivers
I’ll use lead-acid batteries as a baseline. Why? Because lead-acid batteries are the cheapest way to store electricity today. They’re bulky, sloshy, and very heavy, which makes them unsuitable for electric cars or laptop computers. But they’re very efficient, commonly achieving 85% or better energy efficiency in a charge cycle. The technology is well tested, having been around since 1859. And lead is a common element, being the endpoint of the alpha-decay chain of heavy elements like uranium and thorium. Their economic favorability makes lead-acid batteries hands-down the most common battery type in stand-alone renewable systems worldwide.
Large lead-acid batteries occupy a volume of 0.013 cubic meters (13 liters) per kWh of storage, weigh 25 kg/kWh (55 lb/kWh), and contain about 15 kg of lead per kWh of storage.
How do we put this into more familiar terms? A 12 V battery rated at 200 A-h (amp-hours) of charge capacity stores 2400 W-h (watt-hours: just multiply voltage and charge capacity), or 2.4 kWh. 200 A-h means that the battery could discharge a 10 amp current (120 watts) for 20 hours, or a one amp current (12 watts) for 200 hours—though in actual practice the capacity is lower at higher currents.
I can’t resist the temptation to ask: what is the minimum amount of lead that is theoretically needed to build the battery? The chemical reaction for a lead-acid battery is such that each interaction involving the transformation of one lead atom to PbSO4 liberates one electron at a 2.1-volt potential. This electron then is bestowed 2.1 electron-volts (eV) of energy, amounting to 3.4×10−19 J (see page on energy relations). One kilowatt-hour is 3.6 million Joules (1000 W times 3600 seconds), so that it takes 1025 lead atoms (where every one participates). If you remember that Avogadro’s number is 6×1023, we need about 20 moles of lead atoms. At 207 g/mol, this comes out to about 4 kg per kWh of energy, which is a factor of four less than the realized value above. Real implementations always fall short of theoretical ideals, so this isn’t new. We would do well to push for future improvements on this score, although we should bear in mind that lead-acid has had 150 years of development before we get carried away by dreams of perfection.
The National Battery
Putting the pieces together, our national battery occupies a volume of 4.4 billion cubic meters, equivalent to a cube 1.6 km (one mile) on a side. The size in itself is not a problem: we’d naturally break up the battery and distribute it around the country. This battery would demand 5 trillion kg (5 billion tons) of lead.
Get the Lead Out!
A USGS report from 2011 reports 80 million tons (Mt) of lead in known reserves worldwide, with 7 Mt in the U.S. A note in the report indicates that the recent demonstration of lead associated with zinc, silver, and copper deposits places the estimated (undiscovered) lead resources of the world at 1.5 billion tons. That’s still not enough to build the battery for the U.S. alone. We could chose to be optimistic and assume that more lead will be identified over time. But let’s not ignore completely the fact that at this moment in time time, no one can point to a map of the world and tell you where even 2% of the necessary lead would come from to build a lead-acid battery big enough for the U.S. And even the undiscovered but suspected lead falls short.
What about cost? At today’s price for lead, $2.50/kg, the national battery would cost $13 trillion in lead alone, and perhaps double this to fashion the raw materials into a battery (today’s deep cycle batteries retail for four times the cost of the lead within them). But I guarantee that if we really want to use more lead than we presently estimate to exist in deposits, we’re not dealing with today’s prices. Leaving this caveat aside, the naïve $25 trillion price tag is more than the annual U.S. GDP. Recall that lead-acid is currently the cheapest battery technology. Even if we sacrificed 5% of our GDP to build this battery (would be viewed as a huge sacrifice; nearly a trillion bucks a year), the project would take decades to complete.
But even then, we aren’t done: batteries are good for only so many cycles (roughly 1000, depending on depth of discharge), so the national battery would require a rotating service schedule to recycle each part once every 5 years or so. This servicing would be a massive, expensive, and never-ending undertaking.
Who Needs Lead-Acid?
I focus here on lead-acid because it’s the devil we know; it’s the cheapest storage at present, and the materials are far more abundant than lithium (13 Mt reserves worldwide, 33 Mt estimated global resources), or nickel (76 Mt global reserves, 130 Mt estimated land resources worldwide). If we ever got serious about building big storage, there will be choices other than lead-acid. But I nonetheless find it immensely instructive (and daunting) to understand what it would mean to scale a mature technology to meet our needs. It worries me that the cheapest solution we have today would break the bank just based on today’s cost of raw materials, and that we can’t even identify enough in the world to get the job done.
This post does not proclaim that there is no way to build adequate storage to accommodate a fully-renewable energy infrastructure. A distributed grid helps, and an armada of gas-fired peak-load plants would offset the need for full storage. Storage can be augmented by pumped hydro, compressed air, flywheels, other battery technologies, etc.
Rather, the lesson is that we must work within serious constraints to meet future demands. We can’t just scale up the current go-to solution for renewable energy storage—we are yet again fresh out of silver bullet solutions. More generally, large scale energy storage is not a solved problem. We should be careful not to trivialize the problem, which tends to reduce the imperative to work like mad on establishing adequate capabilities in time (requires decades of fore-thought and planning).
so why is solar and wind so attractive if it costs so much more and we cannot build a battery?
Well, for one thing, how's that fossil fuel powered, infinite economic growth paradigm based civilization, working out for ya?
Unless humans find a way to control their population growth all of these discussions are pretty much moot!
Good point. I think it would be useful, rather than just saying that a technology will or will not work, to identify the scale at which it would work and for how long, at least at a back-of-the-envelope level. We might say something like -- this technology would be pretty sustainable for 500 years at 10% of current energy use, using currently known lead reserves. That would allow us either to get used to 10% of current energy use, or 10% of current population, or somewhere in between, if we want to rely on it.
Or use something else for the other 90%.
You could go old school - nickel iron or if the majik-in-a-bottle EEStor-type supercapacitors for the new school.
Other batteries are flow type, pumped water, compressed air and even spinning flywheels in a vacuum.
But as noted in another place on TOD (and given a BS non answer) - how does this energy fact address the long term lack of 'economic' Phosphorous, the erosion of topsoil, idiots playing games with fiat money? At what point does "growth end" on a finite planet?
One way 'tor another there are gonna be limits smacked into on a finite planet.
how does this energy fact address the long term lack of 'economic' Phosphorous, the erosion of topsoil, idiots playing games with fiat money?
It doesn't. It helps to deal with one thing at a time.
OTOH: phophorus can be recycled, topsoil can be rebuilt, and fiat money works pretty well. The idiots playing financial games...see "This Time is Different - Eight centuries of Financial Folly".
It's attractive if you use it where it's sensible to use it, don't waste it and are realistic on what it can do. Asking it to replace EVERY source of fuel for a large, wasteful country when we don't demand that now of any other sources of fuel now is obviously going to make it look bad.
I don't think that he is trying to make it look bad. Electrical storage capacity, as a class, is something that will be needed, functionally, in any renewable system. It seems to me like he is taking the most efficient, abundant form of capacity, and generalizing it out of the abstract to show scale needed.
I would be curious what proportion of total storage capacity other types of batteries might be capable of holding, given known reserves of their respective elements.
It really is too bad that electrolysis of h2o is so energy inefficient.
It's always tricky when such subjects are idealized into one form. ('Battery Storage') This, of course is why electricity is so valuable, since we have a variety of tools to generate it, to store it, and to utilize it, so turning a blind eye to that flexibility of forms carries countless inherent omissions.
I don't think the author was really proposing such a monolithic approach, of course, but just illustrating the issues around chemical electricity storage, doing so without challenging overall electricity demand, which with Negawatts can most dramatically reduce the size of the 'battery' we need in the first place, or any of the other ways that some industries and regions could store renewable energy without assuming it just had to turn into a Stepford Wife clone of today's grid.
Pairing Renewables off with Modified Hydro Reservoirs is one of the most familiar.. and is an approach which, while possibly inviting a new round of Geoengineering and Terraforming Monstrosities, otherwise carries with it fewer chemical dangers or material limitations. It could also be part of a WPA style effort to get some employment for great numbers of people.. Other Hydro and Pumped Air storage proposals use the depths of the seas to provide the 'Spring Lever', and have at least, different and more benign materials-requirements than Chemical Batteries..
But it was understandable that Ida Russkie got in his jab nice and early, from the ever-hopeful position that nuclear is going to somehow become economical and reasonable in enough of its processes that it will actually be a true advantage to our populations generally, and not just a cash-cow and a power-center for a handful, and a waiting liability to the rest. (Unspoken in his post, but clear enough from past comments. Apologies if that was not the subtext to your comment, I-R)
It's true that some of the Massive Turbines being made now seem quite as overblown and industrially topheavy as the Reactors that they challenge.. but I'd be willing to pitch a tent and feed my family under the fallen ruins of the Former.. but not the latter, be it on the day it falls, or a couple centuries later.
If the vehicle fleet is to be electrified, Negawatts aren't going to happen.
Instead of storing electricity, stockpiling other renewables is likely to work better. I suggested storing ~515 million tons of charcoal per year to produce ~3400 TWh of electricity in DCFC's. But I only wrote that 5 years ago, so I expect there are plenty of people who haven't gotten around to reading it yet.
So you want to Poo-poo the idea of reducing Electrical Demand where you can, just because it MIGHT not stay ahead of the increasing demand as we bring some of our Auto and Truck Fleet out of the ICE business, and then you want to instead devote our energies into making manmade coal? There may well be a place for your storage of Charcoal plan as well.. we'll have to include any workable tools and systems we can find, but I'd really prefer making other plans for that carbon.
It seems to me that the EV fleet is essentially a PART of this national battery, for starters, and so in fact including them does preclude making the fixed storage national 'battery' as large as it would have been. No telling if or how these V2G plans will pan out, but nevertheless, it's the usage and the charging options with these vehicles that can help determine what role they play in using and storing the power that the grid has available, with or without their feedback.
Negawatts ARE happening. Read Here In Halifax's comments.. Just because someone else in the system is still trying to build out.. it doesn't negate the fact that some are figuring out how to function with a lower demand of Electricity, and still get the job done.
I'm saying that electricity cheap enough to create a stampede away from ICEVs to EVs is unlikely to also sustain a major push for negawatts.
The "manmade coal" was a thought experiment to show that, yes, we could buffer enough RE to do the job even with a "purist" approach. The losses in stored charcoal are approximately zero. CO2 from DCFC's could be sequestered, used for EOR, etc.
I'm not a purist. I think we'd do a lot better with molten-salt or liquid-metal cooled nuclear reactors, reheating compressed air as the buffer of stored energy. This slashes both the amount of RE and biomass required to run the system, without adding carbon emissions.
You mean "If the motor vehicle fleet is to be electrified". Considering that bicycles, scooters, buses, and trains are vehicles, we could electrify many vehicles and still end up with negawatts, considering that bikes don't need to be electrified at all to work just fine, and we could use buses and trains to replace most of the other motor vehicles. If we have to electrify the entire existing, motor vehicle fleet, with equivalent horsepower, that would be a problem.
No doubt. That's always a faulty assumption.. over and over we go with these narrow-view extrapolations.
IF he wasn't then considering the comment I was responding to, he may have inadvertantly done so.
That being said, there is quite a lot of playing and discussion you could do with this. If America cut it's fuel use, population for example, or what if other countries did this, what would be the amount of recycling to maintain this etc...
For clarity, I am not trying to make solar or wind look bad. I'm a big personal fan of solar, in fact, and would love to see it explode. What I am not a fan of is the tendency to trivialize the difficult aspects of a practical large-scale transition to alternatives. I want us to go there, but we've got to understand how to pull it off or it could turn into a debacle.
"Rather, the lesson is that we must work within serious constraints to meet future demands."
The entire article can be shortened to this sentence. Any child knows batteries can't scale. I guess it takes a physics professor to state the obvious and be arrogant about it.
Next up, a 500 page treatise proving santa clause could never visit every house in one night.
Batteries can't scale?
http://www.ettexas.com/projects/presnas.asp
Note that is a 32 MW-hr battery, 4 MW for 8 hours;
http://www.ngk.co.jp/english/products/power/nas/index.html
http://www.nextenergynews.com/news1/next-energy-news4.14.08a.html
While I've got high hopes for NaS batteries, keep in mind that the "national battery" tmurphy is spitballing would require building 10 MILLION batteries like the one in your first link.
Yes, you can scale batteries up six orders of magnitude from the common D-cell alkaline to a state-of-the-art NaS installation. But you've got another seven zeroes to go!
Well, differently from the lead, litium or nickel, sodium and sulfur are available in enough amounts for today's eletricity use.
The fact that scalling it up would require HUGE projects is, well, not unexpected. That is well within the definition of scalling something up.
Certainly a good point. These problems do need to be fixed, however, some of the mentality that we have to go all solar or we can't do it all are wrong. We can in fact right now do more solar and use it as a sumpplemental power source, and fall back on other energy sources when solar production is low. It can work that way.
ANother thing i would like to say is that we can develop other ways than metal batteries to store energy, which use few resources and are environmentally friendly. One is to store energy as air pressure for instance. Another is to store energy by lifting a weight, such as a block of rock, and then slowly releasing it by a controlled drop of the weight. Just ideas.
We cannot look at the future as though things will be locked in our current time reference.
Let us first assume humans are currently living well past Earth's sustainable limits, in deep overshoot.
Next, let us assume our future activities must balance these sustainable limits and then make a rough estimate as to the human population and natural resource utilization that will allow this equilibrium.
When that is done, we can easily conclude humans won't be needing anywhere near the author's presented values for energy generation. We will not be living in McMansions, driving Hummers and going to work in giant skyscrapers.
Think "basics" like fresh running water, flushing toilets, minimal food preservation capability, humble levels of lighting, home gardens and safe local communities.
Once you accept that level of existence, the calculations for how much solar, wind and energy storage is needed results in almost trivial amounts of power output. Also, we will probably work and travel when the sun is shining, thus eliminating the expense of storing energy for a rainy day (or month). Rainy season? Do what developing nations do - nothing.
Remember, before the fossil fuel era the population of the world was less than 1 billion people and the make up of those humans were majority serfs, minority kings. What would the sustainable population be if we all lived like modern day middle class citizens? Well, let us say I'm counting on technology to maybe allow us to match those numbers. To do that, however, is going to require our collective genius, tolerance and planning.
With complexity comes overhead and that requires energy and resources.
I have several applications running on lead-acid batteries including a solar charged pond pump, an extended life laptop and a wood gasifier stove via a 12v to 3v convertor. They are great if you keep the electrolyte topped up (unsealed type) and the terminals dry. I imagine there would be all kinds of problems if you stacked them in large numbers in racks. A shed big enough to house a jumbo jet might store just one night's energy for a suburb though I haven't calculated it. Factor in inverter losses and heating. With solar charging perhaps a least cost for electricity via l-a-b's might be 30-50c per kwh.
I think we should investigate pumped sea water hydro as per this pilot plant in Okinawa, Japan
http://en.wikipedia.org/wiki/Okinawa_Yanbaru_Seawater_Pumped_Storage_Pow...
The Wiki article curiously omits the octagonal tank at the top of the hill. I've seen estimates that the cost per stored kwh could get as low as 6c. That's for an eight sided tank 7km across atop a 100m cliff. No need for a lower reservoir (in this case the sea) or erosion of rapidly shifting dam levels. If such a tank burst people would get hurt. I have no idea what the scope is for this but I'd ask this question; without storage how do we balance intermittent wind and solar when gas is depleted?
We can use hydro power as a battery. Dams with inadequate water supply but great reservoirs can accumulate the energy over time and give it back when both solar and wind fails .
But i'm not so worryed about electrical power ,a payment plan which taxes MORE the heavy user will get rid of the excesses. I get along very well with 4.5-5.5 kwh a day and have all the modern day things for 3 people .
Darn right we can use Hydro. In New Zealand it's done that way. Too obvious. I run my house on a pelton-wheel, using a litre a second @ 80ft. It would be easy to use wind to pump water back up, to a pond or tank. I don't do it because we have only run out twice in 17 years (and if it's drought enough for that, solar must be happening in a big way) but as a closed-system it's much better than lead-acid. Losses? Who cares, bigger blades (the wind is free) and a bigger tank.
Works in macro form the same way.
Consider that hydro dams currently provide 3% of our total power. If we used this current infrastructure to act as a battery, we would quickly run into trouble. For one thing, it is not easy to have water gush out at arbitrary rates, so even if the storage volume is adequate behind them to last for days, the rate at which we can extract power is not likely significantly bigger than the current 3%.
Most of the obvious hydro sites are developed in the U.S., but we could conceivably double it. Still not good enough. It's easy to say one solution or the other would just work. Problem solved. But the scale of the problem often prevents such dismissals.
Tom - here's a study of dams on federal lands which evaluated existing dams as potential power producers. The study looked at height/head, inflow and distance to existing transmission lines and found several dams which could be converted and brought on line.
http://www.usbr.gov/power/data/1834/Sec1834_EPA.pdf
The study did not evaluate those dams for pump-up hydro potential, but one could easily guess the numbers by simply adding back in the dams which were excluded from the power producer list because on inadequate inflow.
We've got tens of thousands of existing dams in the US.
Additionally, pump-up can be built without using natural sites. Closed loop pump-up is totally feasible. Create two artificial reservoirs, one significantly higher than the other. After the initial filling all the 'outside' water needed will be evaporation replacement.
In areas with limited elevation change the lower reservoir could be an abandoned mine, underground cavern, or even excavated chamber.
I suppose lead would go from a buck or two to maybe four or five times a pound if anybody tried to to build so many lead based batteries.
It isn't going to happen, at least not anytime soon-lead is not so far as I can find out on short notice all that plentiful..
That was amusing, i thought this was going to be a cornucopian tech post, before it dropped me down reality canyon.
I don't believe enough attention is being paid to the possibility of redesigning appliances and other electricity consuming machines for use when the power supply is intermittent.
It looks as if it would be from a technical pov a trivial problem to superinsulate a refrigerator and add a couple more cubic feet of volume to the entire machine-that two cubic feet to be occupied by a water reservoir that would be frozen and chilled to a subzero temperature-such a refrigerator would probably stay sufficiently cold for a week or longer with no input power at all, excepting possibly a small built in rechargeable battery to run a circulator fan.
Water heaters can be similarly oversized and super insulated, to take advantage of any surplus wind or solar power, and ac systems can incorporate tanks of chilled water or ice to be made when juice is available.
We are going to see a simply awesome move towards energy conservation and efficiency driven by simple dollars and cents considerations within the next few years.Of course it won't be soon enough, or large enough to solve our problems.
In the end, our great grandchildren will simply have to get by with whatever renewable power they can afford collectively and as individuals.
This brings up an interesting point.
Let us suppose that a building code mandating new construction domestic solar hot water and a couple og kilowatts of pv were to be put in place in sunny parts of the country-with all the advantages associated with standardized design and installation procedures, plus the volume brought on by the mandate.
Does anybody here have a good estimate as to how much this might reduce the cost of installation?
My own wag is that it could easily result in a savinggs of fifty percent or more, based on my experience in trade work-it takes only half or less as long to build a new bathroom from scratch as it does to remodel an equivalent sized old bathroom .
Installation is the real deal killer right now and will be the deal killer even more so in the near future as module costs continue to come down.
Mac about $5/watt total installed cost about $1.50/watt for panels. I'll let you work it out, remember that includes the paper work.Just aver the boarder in Germany about $3.50/watt total installed cost I was talking to an installer in a German village just up the road. I was wondering if I could justify a system here in Holland without the F.I.T. still too expensive.
Just go back to the IcyBall idea, though perhaps regenerated with a pump instead of heat. Melting ice yields 80 BTU/lb, vaporizing ammonia yields upwards of 590 BTU/lb. Plus, this keeps freezers at freezer temps.
If the change in practices included use of SIPs such as ThermaSave, the PV could be integrated into prefab roof sections at the factory. Lift into place, screw together, plug in. The saving on installation labor would be huge, I suspect.
I don't believe enough attention is being paid to the possibility of redesigning appliances and other electricity consuming machines for use when the power supply is intermittent.
Or even human habits.
Before the trustbusting of the 1920's the electric firm owned and used electric rail as a dump load/regulator for the power grid.
Right now you have people who's position is "we've got to run the system as it is" VS 'Oh hey - perhaps there is another way'.
In the end, our great grandchildren will simply have to get by with whatever renewable power they can afford collectively and as individuals.
Ala Tom Jefferson 'farmers on the land' model.
This sort of fridge is available now and is called a 'eutectic refrigerator'.
Usually these are seen in caravans and boats and work really well.
Couldn't agree more about the insulation design in the modern family fridge. Apparently, all consumers want a fridge like Dr Who's Tardis -- bigger on the inside than the outside.
Modern smooth back fridges put more demands on the insulation by using the sides as the radiators, also meaning that the fridge has to be smaller to allow air circulation on its sides.
I'll try and remember to double check mine tomorrow. I believe the coil is air vented in the base. an not in the flat panels. I hope it is not the back as I want to add insulation.
NAOM
Examples of more practical energy storage media:
- Hydrogen from electrolysis of seawater, and Ammonia produced using the hydrogen and energy from renewables. Ammonia has about one third of the energy density of hydrocarbons, which is extremely good.
- "Molten-salt" technology proved itself in the 90's. The salt can be obtained from seawater which has been electrolysed. The molten salt can be stored for a week and possibly more.
I think this has crossed many peoples minds already: have windturbines generate Ammonium at times when high winds produce more energy than can be transported or used and oxidize the Ammonium during times of low wind or peak demand. The process of creating hydrogen for the Ammonium is not very efficient so costs per produced kWh will be high. But maybe we should allow energy prices to rise in times of low cheap supply which should trigger more flexibility on the demand side.
The same has happened way back with the rise of rigid coal plants which are difficult to cycle between peak day and low night demand (e.g. due to thermal stresses). This introduced high/low pricing schemes in many countries for day/night&weekends to level the demand a bit. If we have done this before, why can't we do this again but smarter? E.g. make the biggest energy users like warehouses for frozen goods, (aluminium) smelters, and other energy intensive industry more flexible and provide incentives to do so.
Yes, it has crossed a few minds. Both ammonia and thermal storage have excellent scaling properties. As a matter of fact we have enough ammonia storage in Iowa *already installed* to handle seasonal demand for ammonia fertilizer that could store about 20 Gigawatts of wind generation, and store that wind energy for 3-6 months.
In reality, if the economics can be proven to make ammonia energy storage work, we're going to suck up all the capacity for industrial uses and fertilizer before we ever start burning ammonia for energy. Of course there are already commercial NH3 fuel cells already.
This is a solved problem. All it takes to deploy is about $4million to build the first 1MW ammonia production unit. (disclaimer: I am a shareholder in the group doing Freedom Fertilizer)
Perhaps you can help me, then.
Ammonia as a fuel appeals to me, and I'm mapping out a plan for useage in my spare time. Problem is that I'm not really good at maths, so converting 1 Ton of AA into Litres is... difficult for me. :)
The service I am looking at requires about 500L of Diesel per day. A Tonne of Diesel is about 1150L, so 500L is *about* half a Ton. Given the different energy densities, do you have any idea how much Ammonia would be required as a replacement?
I see on the old FF blog that one of the 1.65MW turbines combined with the Freedom plant might produce 1 Ton a day.
If I was looking for electricity storage today, I'd stay quite away from anything that involved a thermal machine.
In the future that requirement may change. But just that change is already a signal that things aren't good.
Agree. If the Carnot efficiency law applies to your energy storage system, you lose.
Concise article, but one question..
Maybe I lack a fundamental understanding on efficiency metrics, but I don't understand why, if lead batteries are 85% efficient, that their realized capacity is a factor of four lower than their theoretical limits. I had always assumed efficiency was the ratio of realized values to theoretical limits.
Nevermind, figured it out.. Factor of four is capacity, efficiency is energy lost in the process. I would still consider capacity a form of efficency, but one of resource efficiency, as opposed to energy efficiency.
The lead-acid battery uses ~4x the amount of lead theoretically required because most of the lead is used to carry current between the active material and the terminals. The common failure mode for these batteries is for the support grids to corrode and break, disconnecting part or all of the active material in a cell.
Firefly Energy proposed to radically improve the PbSO4 battery using carbon as the mechanical support and the conductor, lightening the battery and eliminating most corrosion problems.
The lesson here is that it's easy to be mislead by simple calculations and linear thinking.
To begin with, why would one want to supply ALL the present US energy demand with batteries powered by renewables? The best application for solar energy these days is solar thermal, which can provide low temperature heat energy at a cost which is presently below the retail cost of electricity, given the same economics. The heating demand to the end user is often low temperature, such as hot water and space heating and the thermal energy can be stored on site, if the system is so designed. We know that AC demand is directly tied to the amount of solar energy available and solar PV can be optimized to closely match that demand, especially if low temperature phase change storage (ice cycling) is used. Removing the applications which could benefit from a transition to solar would greatly reduce the overall need for the electric storage which the author calculates, especially when multiplied by the stated need for 3 day storage.
The second hidden assumption is that the present transport system primarily using private automobiles and trucks will continue into the future. Of course, we could transition to electric powered vehicles, but these already have storage built in. Using a large storage capacity to recharge small storage in cars during a brief period of low energy availability would appear massively excessive. Then too, there's the possibility that rail commuting could replace a large fraction of vehicle use and that could be provided by using much less electricity. Also, people might just take a short vacation during those occasional periods of extreme outage, as they are forced to do after large storms cut power and roads.
The basic assumption here appears to be that BAU will continue without interruption. It's grossly unreasonable, IMHO, to think BAU will continue as before, once Peak Oil kicks in and the decline begins. The problems we face as the result of our massive consumption of fossil fuels are as much the result of over consumption as lack of alternative supply. We need to get away from thinking that the future energy we will experience can be simply a repeat of what we all experienced in the recent past...
E. Swanson
I could not agree more. As I will often do, I step into a mindset that I believe to be common: that we can continue BAU, that we can snap our fingers and scale up any idea that pops into our minds, that "peak what?" is not on the radar, etc. I do not personally share these points of view. Of course we'll see diversification; almost certainly we'll lower demand; in all likelihood we'll design for intermittency. So if you're already on board, my efforts to show that the prevalent "why can't we just..." attitude has pitfalls will seem simple-minded.
You're right that the electrified fleet storage would offset part of the monolithic lead acid battery. But while we're fantasizing about BAU, note I held demand flat. But even if you knock my over-estimate down by a factor of two, we still would not have enough lead in the world for the U.S. lead-acid battery.
tmurphy,
Is disappointing that you consider lead acid battery storage but not what is presently being used in most parts of the world to store surplus electricity; hydro and pumped hydro.
The limitations of most hydro is the low delivery capacity, but can deliver for up to several years, depending upon dam capacity. Up sizing of generators can be a low cost method of increasing delivery capacity.
The limitations of most purpose built pumped storage is the low storage ( usually only a few hours output) ideal for daily peak demand, as it was designed to provide.
If you want to imagine the need for much larger storage, think of Lake Eire/Land Ontario, approx 100m difference in elevation, 18,000 km sq surface area; 18 billion cu meters/m change in lake level. Many other possibilities also exist for very large pumped storage but havn't been built because so far there has not been a need for more than a few hours storage.
Lake Winnipeg is the world's largest hydroelectric reservoir. elevation 217 m.
H'ammm
Let water out through enlarged dam power station, then pump the same water back up ?
Something to at least look at.
Like pumping water from Lake Ontario back into Lake Erie.
Alan
I step into a mindset that I believe to be common: that we can continue BAU, that we can snap our fingers and scale up any idea that pops into our minds, that "peak what?" is not on the radar, etc. I do not personally share these points of view. Of course we'll see diversification; almost certainly we'll lower demand; in all likelihood we'll design for intermittency
But the BAU grid already includes diversification and design for intermittency. Now, we need to do much more of that and phase out FF. But, the barriers to that aren't technical, they're political.
The problem here - you're knocking over non-existent problems.
Interesting article. I'm sure folks like me (we're going into our 16th year off-grid, PV, lead acid batteries) read it with some amusement. Not to belittle the post, but it itterates a few things like this:
"Obviously, this calls for energy storage, allowing us to collect the energy when we can, and use it when we want."
...when we want...Those of us living on solar and batteries have come to terms with needs vs. wants. If you want to watch TV all night then you may need to wait to do your laundry. If you want to fully cycle your batteries every day, then you'll need to buy new batteries much more often. I could go on, but those who have chosen to live within their solar (or wind, etc.) intermittant allotment learn quickly to manage their consumption. I doubt this meme scales well. Too many folks feel entitled to use as much as they want, when they want, especially those who can afford it. We've become used to people asking us "What if this?, What about that?, What happens when? They are locked into focussing on what they perceive as energy poverty, intent on finding flaws in our choices.
The way I see it, I don't have a production/storage problem. They have a consumption problem. Further, their unwillingness to use more restraint in their energy use hurts us all. We, collectively, could gain so much through conservation, efficiency and restraint, but Jevons rules. What we get is electric billboards. One man's negawatts are another man's opportunity.
Keep your solutions local.
Nevertheless, when all is said and done, the lowly and boring negawatt will ultimately prove to be the most practical and achievable long-term solution to the worldwide energy problem. There is no magic bullet to replace fossil energy. Renewables and smart grids and fusion fantasies alone cannot supply the growing electricity consumption levels of industrialized nations.
If you're minimizing your household electrical consumption while your neighbor is offsetting your economies by running their air conditioning and plasma television twenty-four hours a day, there's no need for embarrassment. You're acting in a thoughtful and responsible manner and they are not. The Jevons Paradox is an economic observation, not an ethical one. Needs and wants are not equivalent.
In our competitive and consumptive Western culture, thoughtful and responsible people are commonly regarded as 'losers', while those who aggressively take all they can get are often viewed as 'winners'.
How will we come to define winners and losers in a future era of economic and material scarcity?
Sure, the 'strong' may pillage the resources of the 'weak' when things start to get really tough, but eventually even those strong survivors will have to adopt a 'thoughtful and responsible' approach to living, or there won't be any of them surviving.
Hmm, perhaps an opportunity for slingshot target practice... It's been a very long time since I made my own slingshots and went hunting for small game.
Yair...a bit off topic but two things that to my simple uneducated mind are quite remarkable...and most folks take for granted.
1/ On the desk here is ten dollar solar/battery calculator. It's about three years old and the battery is flat but if I put it in the sun or under an incandescent light even it will do the most complex of calculations.
2/ In the garden are some five dollar solar powered lights. If I remove the top section from the fancy glass diffuser the little LED will provide about the same amount of light as a small candle...you could if needs be read. So for five bucks we have a little solar charger, some kind of battery, a light sensing switch, the LED and the housing. They have been going for twelve months now and on a reasonable day will run till well after midnight...I get annoyed when folks tell me solar is "useless" and if I point out these two examples they look at me as if I'm nuts
Sabatier won a Nobel prize in chemistry to transform hydrogen and carbon dioxide to methane using a catalyst. Instead of thinking of the problem as power storage, we should look at this issue as a means of transforming energy into fuel. Instead of storing energy in a battery, we store the fuel in tanks...
For those who don't know, if you don't regularly charge a lead acid battery, it will shorten the life of it, and even a new lead acid battery will become worthless in a few months if it's not charged. Completely discharge a lead acid battery, and you shorten the life of it.
For more on the chemistry behind this, look up lead acid battery sulfation.
2 starters for people
http://www.windsun.com/Batteries/Battery_FAQ.htm
http://batteryuniversity.com/
NAOM
All this article shows is that lead acid batteries are not a solution to utility scale power storage. The author starts out by saying this would be a good choice because they work so well at a small scale and then works through the math and discovers they simply don't scale up. It would make more sense to do the analyses on utility scale solutions like pumped storage, high temp salts and momentum fly wheels. All of which could not compete with lead acid on a small scale but I suspect would work much better at the utility scale.
It's simply silly to assert at the beginning of the article that lead acid is the best solution.
It seems you didn't read the article very carefully, or missed the point. That lead-acid batteries is a solution for massively scaled storage was, as he said, a base point hypothesis. It didn't take long to understand the problems. He tried to lead the reader to reach this conclusion, though I guess it didn't work on some.
Yes, but what's the point? If there are solutions that are enormously better suited to the task, why spend the time debunking lead acid?
Tom
We get power outages (they used to be much worse than now in our rural area, and I suspect we could go back to that situation). I am interested in UPS and long-life batteries. (I remember car batteries used to be available, stored dry for use a few years or so down the line?) Golf-carts seem to be deep-cycle? 350 cycles? How does that marry with intermittent trickle charge and mostly 'light-use'? Might be better to have high-quality small diesel generators and a few quarts of locally grown diesel and only one deep battery (and one in the store?)?
I'm trying to get my head round 'sensible' investment to cover the next few decades.
Also any thoughts on this from OFM?
A well designed off-grid system is, essentially, a big UPS. A UPS (as for a computer) is simply a battery and an inverter/charger. My inverter/chargers are scaled up versions (2-4KW, 1-2KW). If we had grid power available we could charge the batteries using the inverters (they have grid connections and transfer switches). All of this can be programed/automated with most of today's inverters. One can set the inverters to charge the battery during off-peak periods. They can also be programed to autostart the generator.
You mentioned a small diesel generator, which is what we have, primarily to equalize and maintain the battery, but also useful for long cloudy periods. While we could live well on our solar allotment, lead-acid batteries need to be topped off periodically to reduce sulfation. This is a mistake many off-grid newbies make; their batteries rarely reach full charge and fail prematurely. Iron-nickle batteries don't have this issue but are 4 to 5 times as expensive per KwH and have higher standby losses.
I know one guy who has maintained his lead-acid battery set religiously for 15 years and it still likely has 80% of it's original capacity. He equalizes every week from his neighbor's grid connection. He also installed an automatic watering system, as have I.
Golf cart batteries are deep cycle. Sometimes they are not "true" deep cycle, but the Trojan T-1275 batteries I have use the same internal plates as their reneawable-targeted T-105 deep cycle batteries. For your sort of application (very occasional discharge), golf cart batteries should be fine. I routinely discharge my batteries to 50% (daily), and have gone about 500 days on this set with no significant degradation yet. Keeping the batteries topped off with trickle and infrequent discharge should lead to long life if the electrolyte is kept full. But you may be dismayed at the cost to give yourself adequate storage for a day or two—if that's your target.
Golfcart batteries are generally a poor choice. They aren't designed for constant cycling and usually don't last. For smaller battery sets, some of the RE AGM batteries do well, and the L-16s are considered an excellent choice. For larger battery banks, I recommend the large 2 volt cells, such as forklift batteries (I have these ) or these.
Thanks both.
Hope others also find info useful.
phil
One of the biggest problems with lead acid is that the plates become disorganised as the battery is cycled. Generally, the design life (5 or 10 years for 'solar' batteries) is only achieved by maintaining a typical depth of discharge of about 15%, resulting in a huge overcapacity in the battery.
In the sort of buffering battery we are talking about, this is a feature, as it allows for occasional deep cycles corresponding to bad weather.
I'm behind the curve in this respect-I haven't any wind or hydro power resource and I am waiting for pv costs to drop some more before going that route.
I do have a small 3000 watt Kawasaki generator that will be used to run the refrigerator and freezers for a few days if necessary. It comes in very handy occasionally around a farm where extension cords mostly won't reach.
Unfortunately it won't run the deep well pump -or the pump in the spring that supplies everything except our domestic water.
For that I will use my welding machine, which has 240 volt thirty amp ac power.It cost over three grand years ago, and it is a fuel hog, but I wouldn't need to run it more than a few minutes a day to get enough water.We keep at least fifty to one hundred gallons of gasoline properly stored on hand, and rotated every few months.
If I were to buy another new welder, I would get a diesel so I could store the fuel more easily.We already keep a good quantity of diesel on hand-enough to grow ALL our own food for three or four years in the event we must, as well as some for the rest of the family.
Gravity powered domestic water is in the works from a spring UPHILL from the house, I just haven't ever finished the pipeline which will be a couple of thousand feet long..I bought the pipe long ago.
We live in the edge of coal country, and so far we have had extremely reliable electrical service, averaging only a few hours of outage a year, mostly due to storm damaged lines.
Mac- Get that gravity flow system working. Not only does it save energy, pump wear and maintenance use up time and resources. My parents system is still working after 40 years. The new owners replaced the old concrete tank with a poly tank a couple of years ago, but the system keeps producing year after year. Just a hike up to the spring box on occasion to clean it and get the spring lizards out, a good gravity flow system is a beautiful, simple thing. My system was #1 on my list when I put down stakes; perhaps the main reason I chose our site. Hire some kid with a ditch witch.
(GRIN)Hi Ghung
I have recently acquired a BIG shiny four wheel drive backhoe of my very own-every guy should have at least one status toy, and this is mine, long delayed.
At least it is not the hot rod red convertible which lots guys who are getting old and fat buy to try to recapture thier youth! ;-)
I couldn't really justify the price in terms of utility,not needing it often enough, but she has already make short fun work out of some long delayed improvements on the place and it will take only a day or two to dig the water line-which runs thru the woods most of the way where the ground is too rough for just about any other machine.
Some family members have working gravity water already-some of the systems are nearly a hundred years old already, and as you point out, they very seldom give any trouble-the pipes need replacement every four or five decades, that's about all.
My pipe is extremely heavy duty electrical conduit, schedule forty,inch and a half inside diameter the kind that gets plowed in with a bulldozer to lay high speed cable-it cost a buck and a half a foot a decade ago-got it for nearly nothing just after the dot com crash.I have QUITE A BIT OF IT.If you will come up for a day to visit, I will give you a five hundred foot roll of it, some homemade cider, and a good dinner and consider it a bargain to have the opportunity to pick your brain in respect to pv in particular and renewables in general.
It's made out of exactly the same stuff as most plastic water pipe, only about four times as thick.I expect it to last more or less indefinitely-maybe as much as a couple of hundred years when buried.
Sounds great Mac! I've got a 1600 gal. poly tank to trade. Used, but sound; DOT approved, food grade. The chicken hatchery up the road used it to put egg shells in; ground them up and put them on folk's pastures and roads. A few gallons of bleach will clean it right up. If I can get my wife to allocate the fuel funds we'll set it up.
Ghung321[at]yahoo[dot]com. BTW: how far from Asheville are you?
If I were to buy another new welder, I would get a diesel so I could store the fuel more easily.
Propane and Alcohol are, in theory, "forever storable" so long as the container remains intact. Both should be able to work with what you have ATM - if you wanna play some games with the engine.
Here is a L-16HC that is less expensive than the one Ghung linked. My previous battery array lasted 14 years. My current one is 6 years old using these Crown 395 A·hr batteries.
What sort of depth of discharge did you use on those 14 year cells?
NAOM
For the first 3 years they were discharged more greatly, a few times down to about 30% charge. After I rotated my PV array in azimuth to receive more sunlight and eliminated my old power hungry TV, I cycled them to about 90% charge each day. Down toward the bottom of this thread I posted a chart showing depth of discharge vs. number of cycles for lead acid batteries. Based on my experience that chart is accurate.
Thanks for that. Yes, I saw the chart and bookmarked that page.
NAOM
Cross-posted at http://physics.ucsd.edu/do-the-math/2011/08/nation-sized-battery :
This is just the kind of calculation I love. There's plenty of room for argument about whether we actually need to store the entire US energy consumption for a week, but this calculation gives us the right ballpark answer.
But I started to wonder about sodium-sulfur batteries, an emerging technology I've been really intrigued by. The disadvantage of NaS batteries is, you have to keep them hot so the sodium and sulfur stay molten. However, if you build them really big, this is no big deal. The advantage is very high energy density, plus they're made from two of the most abundant elements on Earth.
I took the time to re-do your calculations for Na-S batteries.
Like lead, Na-S batteries produce about 2 volts of potential. But since the atomic weight of 1 Na + 1 S atom is about 1/4 the weight of a lead atom, so you get about 4x the energy density of a lead-acid battery:
http://www.wolframalpha.com/input/?i=2.1+eV%2Fatom+%2F+%2854+grams%2Fmol...
I calculate 1.0 kg of Na+S per kwh of energy stored, compared to 4 kg/kwh for lead.
Real-world sodium-sulfur batteries have an energy density of 4.5 kg/kwh (3.5x better than lead batteries), so the theoretical advantage does carry over into the real world.
Na and S needed per kwh:
0.4 kg Na
0.6 kg S
To build your 360 billion kwh "national battery", we need:
216 megatonnes S
144 megatonnes Na
World annual production of Na and S:
70 megatonnes S (minerals.usgs.gov/minerals/pubs/commodity/sulfur/mcs-2010-sulfu.pdf)
0.2 megatonnes Na metal (http://environmentalchemistry.com/yogi/periodic/Na.html)
However, the low production of Na metal is mainly because there's no demand for it. It's produced by electrolysis of NaCl: the chlorine gas is extremely useful, but the Na is less so: it's usually converted to NaOH (caustic soda) and sold dirt cheap. Salt electrolysis plants could probably retool to produce more metal and less caustic soda: world chlorine gas production is over 40 million tonnes, and NaOH production amounts to about 60 million tonnes (http://en.wikipedia.org/wiki/Sodium_hydroxide), which works out to 33 million tonnes of Na.
Thus, with a little work, our current infrastructure can crank out a "national NaS battery" in about 5 years. (144 megatons sodium needed / 33 million megatons produced per year). There are economic issues to consider, but from a physics perspective, this is doable!
The comparable figure for lead batteries is 1300 years.
On further research, I see it's not a simple matter to convert current salt electrolysis cells to produce Na instead of NaOH. But the point remains: because of the abundance of Na and S and their high energy density, production of a national battery using NaS technology is orders of magnitude easier than using lead.
Excellent work! Thanks for contributing this. It's interesting that the NaS energy density scales so simply with lead, suggesting that both are about a factor of four shy of the theoretical maximum (except I have not done a careful accounting job of how many electrons per reaction in NaS: it may be a factor of two better, as I would expect in a liquid form).
Ah, good point. Since each lead atom in a lead-acid battery releases 2 electrons as it reacts with sulfuric acid, you get 4 eV of energy per atom, not 2, so you need half as much lead. The same isn't *quite* true for NaS:
4 Na + 2 S -> 2 Na2S
Na -> Na(+) + 1e
S -> S(2-) + 2e
so the math above works out to 108 megatonnes sulfur, 144 megatonnes sodium. But the conclusion still holds: while there's not enough lead in the world to make a national-scale lead-acid battery, building a huge NaS battery is a big job, but not impossible.
Careful about lead accounting: the reaction involves one lead atom in the PbO2 plate and one lead atom in the straight Pb plate, each becoming PbSO4 and liberating two electrons in the process. So each lead atom can claim responsibility for one electron, not two. But I had not looked at the NaS reaction/accounting.
If sodium from salt production is boosted massively what would happen to the chlorine released? That is not exactly a good gas for atmospheric dumping.
NAOM
"If sodium from salt production is boosted massively what would happen to the chlorine released?"
As it happens, one of the main inputs for making silicon solar cells is chlorine. it arrives on site in the form of SiCl4 or HCl.
It leaves as various metal chlorides or CaCl2, or as NaCl. The sodium came in as Trona, (sodium carbonate.)
Hmmm, Calcium Chloride to dehumidifiers, Sodium Chloride recycled, interesting.
NAOM
PVC plastic would get a whole lot cheaper. The industrial chlorine and sodium markets have always been linked by the stoichiometry of NaCl, and demand shifts for one cause price swings in the other.
But I think the most likely outcome is that any excess chlorine will be combined with the hydrogen released by the chloralkali process to form hydrochloric acid, and sold. Who will buy it? Many industrial processes which need a strong acid can use either sulfuric or hydrochloric. At the moment, sulfuric acid is cheap, but once NaS battery makers start buying up all the sulfur to make batteries, HCl will become the preferred option.
It's kind of slick, actually, how well it might work out.
And there is always the use of the Na + water as a power storage solution.
http://web.archive.org/web/20040616090321/http://www.powerball.net/
(it seems a cyber-squatter has the domain now.)
First you got me thinking about a NaO + H2O battery, but those NaH pellets are interesting too.
A better option todumping it in the atmosphere is todumpit in the oceans. It does kill everything in its way, but over time those Cl atoms will find Na atoms to pair up with and neutralize. Atmosphericdumping is the worse of the two.
Your redox chemistry may be a little rusty (pun intended): there's a big difference between elemental chlorine gas and the chlorine ions in seawater. To stop Cl from being poisonous, you need to let it grab an electron from another neutral atom. Almost anything that burns in oxygen will do. Up-thread, I suggested using the hydrogen that's produced as a by-product of chlorine manufacturing, producing hydrochloric acid, a useful industrial chemical.
Most of my ideas have already been mentioned above. But consider the following system -
A "Wind Export Belt" from northern Alberta, Saskatchewan and Manitoba to New Mexico - Texas and NE Mexico. Most other wind is used regionally except Atlantic Canada & Quebec which export wind when available and hydro otherwise.
Solar thermal in desert SW USA and NW Mexico
Solar PV most everywhere, but most below 35 degrees latitude or clear skies area. density increases with insolation.
USA adds 15 GW of small hydro plus increased efficiency and capacity at existing hydro. Quebec adds 25 GW, Newfoundland 7 GW, BC 2 GW, Manitoba 5 GW of new hydro. Canada adds more turbines at existing and new hydro to increase peak power.
Efficiency gains reduce US and Canada energy consumption to EU rates, i.e. cut in half. Further gains from "more efficiency" and electrification cut demand by another third despite rising population.
Power costs 4 cents to 60 cents/kWh, depending on supply & demand. At 4 cents/kWh it makes economic sense to synthesize fuel (ammonia is my choice) to burn at 50 to 60 cents/kWh.
This economic incentive also leads to load shifting. Load new washing machines with dirty clothes and set to run when power is <7 cents/kWh. Freezer drops temperature 8 degrees when kWh =< 5 cents/kWh and lets temperatures rise to 27 F (-3 C) when kWh >28 cents/kWh. Weatherman gives power price forecasts as well as rain & temperature forecasts.
HV DC lines (mainly E & W from Wind Export Belt) and from Canada hydro south shift renewable supply to demand (4 hour offset East Coast to West Coast).
Massive pumped storage projects everywhere economically practical (with 4 to 60 cent spread - that is a lot of places).
Lake Winnipeg and the Great Lakes are "held back" in the spring and released in the summer for seasonal shifting of power.
Under-girding all this is a nuclear power backbone. Most built inside mountains for extra margin of safety. Every mild night, Nuclear power exceeds baseload . Wind and excess nuke goes into pumped storage.
Charcoal is kept by efficient coal fired plants for back-up @ 60 cents/kWh.
Batteries not Required,
Alan
Alan, good ideas. With a diverse energy supply the need for storage batteries will be reduced or eliminated.
I agree with the Wind and Solar belt. However if you would marry this with a Water Transfer and Storage system.. you could store part of the energy generated.
I've been intrigued with NAWAPA ever since I heard about it.. There was significant engineering done in the 1950's and 60's.. The Transfer of 4.4 Billion AF of Water from surplus to market.. While generating tremendous amounts of Hydro.. as well as providing stored power.. with the pumping of water up hill during off peak electricity demand for release later for Hydro at peak creates a natural battery.
If you married the renewable generation of Wind/Solar.. along with Hydro.. with the movement of water down the NAWAPA system.. You could solve several intertwined problems.. 1)Increased Electrical supply 2)Increased fresh water supply for the inner Mountain West.. for development as well as.. 3) Increased food production.. 6 Billion people on the planet headed to 10 Billion in 50 years. 4) It would create a significant amount of jobs and economic activity.
Here are a couple of links.
http://en.wikipedia.org/wiki/NAWAPA
I don't endorse the LaRouche outfit.. but they do have a cool video on the NAWAPA system.. and is worth a look if interested.
http://cecaust.com.au/main.asp?sub=media&id=2010_08_30_tour.html
NAWAPA builds around some of the natural strengths of North America.. the abundant water supply.. the Rocky Mountain Trench.. and the cities and farms that would flourish with the nourishment of the water that would be provided.. as well as the power generated and delivered.
This system would run down the spin of your wind/solar belt.. so building the transmission lines from it/along with it would make sense.
Granted this is a very big project.. so.. I understand a little bit of dreaming here.. but still Hydro storage along with wind/solar makes for your "natural battery." This is on a scale "that matters."
I've always thought that this was an exciting idea. We've discussed the possibility of pumping Mississippi or Great Lake area flood waters as far as Lakes Powell and Mead. A couple of caveats seem to be massive costs, and the possibility of transfering unwanted aquatic species (i.e. zebra mussels, asian carp, etc.) into other watersheds. This project would be on the scale of TVA, if not larger. Tough to do politically and economically considering the times.
Another big idea that will likely never be (90 min video in parts):
Cadillac Desert: Water And The Transformation Of Nature - Mulholland's Dream
Add to all these good ideas something new but simple: underwater balloon energy storage. I'm sure there will be many other new storage technologies that will be coming down the pipe now that there is the need for storage. As you have suggested, the next generation energy generation and storage system will not be a one-technology system, but rather the integration of many different systems. Some small part may even be lead-acid batteries :)
Alan. its always good to hear you saying so much of what I am thinking. Gives me a sense that I can relax at last and let all you good young guys carry the flag.
All this sounds great. I would add a little touch- solar cities at ideal sites combining seacoasts, intense solar, nearby elevations for sea water pumped storage, and other things needful, like peaceful surroundings. Baha california looks like one candidate.
And the red sea, except for the peace part.
So one could put things like aluminum smelters and such-like energy gobblers there, served by ships to and from everywhere.
But then-- always that downer thought-- energy is not a tech problem at all, it's a people-with-wrong-heads problem. MUCH harder to fix.
Many thanks for all your good work.
What we really need is a way to generate wind and/or increase wind power through structure layouts and topography.
Another battery alternative that changes the economics would be nickel iron batteries. Unlike lead batteries, good only for a thousand cycles or so, nickel iron batteries last, if not exactly forever, for very, very much longer. They are also *very* forgiving of over- or undercharging.
A nickel iron battery is like an ox. Big. Dumb. Heavy... and it *just* *keeps* *working*.
Cheap too. Nickel and iron are both fairly common and neither is very expensive.
Practically speaking, of course, it would make much more sense to build a few thousand town batteries rather than a large national battery, with inputs from solar, hydro and anything else we can think of. One thing can break. A few thousand things? Less likely.
Distributed generation and storage of power is a good long-term goal.
For Americans at least, it will take a major cultural shift for us to start thinking small and local. We don't seem to be done with thinking big and global quite yet, although the events of the past few months have gotten more people wondering about our present course.
Not to say you couldn't install a nickel-iron battery bank in your home right now. If they last as long as they are supposed to, you or your descendants could end up contributing your cells to a consolidated neighborhood or town battery. Might as well get started gathering the resources.
As the author pointed out, there's not enough nickel in the world to do this. Repeating his math for nickel-iron batteries, I calculate that a "national battery" made from nickel and iron would require about:
78 megatons nickel
73 megatons iron
World nickel production is about 1.5 megatons per year, and presently-recoverable reserves are about 60 megatons.
Sorry, but this is nonsense. The "recoverable" reserves of ANY metal is somewhere around 40-80 years of present production. This is because "recoverable" is defined in economic terms, which means only the very best deposits are considered, while the rest is disqualified.
Nickel is abundant but not very useful. This means that even though its crustal abundance is 80-90 ppm on average, only 1.3 MT/year is mined. However, zinc at <80 ppm is mined at a rate of 12 MT/year and copper at <70 ppm on average, at 15 MT/year.
This means that if we want to do that battery, nickel mining would be quite possible to scale to satisfy demand.
"Nickel is abundant but not very useful."
Not very useful? How about the entire 300 series of stainless steels, and the duplex stainless steels, monel, copper-nickel, all the Inconels, all the Hasteloys, the Incoloys (which are not inconels), Alloy 20, and Nickel metal hydride and nickel cadmium batteries, and a whole bunch of catalysts.
As for abundant, there was 1 nickel mine in the US, at Riddle Oregon. It closed when the ore ran out.
And Nickel is important enough that the US of A put Silver in Nickels to keep the Nickel "for the war effort" back in WWII.
I concede the point: copper is a great counterexample. BUT, you're only going to increase mining rates by a factor of 10 if you drastically increase the price of nickel. Nickel isn't particularly cheap now (it's about $22/kg, 10x the price of lead, twice the price of copper), and one of your big selling points for NiFe batteries was the price.
Even at *today's* metal prices, the nickel in a NiFe "national battery" would cost about $2 trillion.
It seems, then, that nickel is a bit more evenly distributed in the crust - otherwise it should be much cheaper than copper. That's a drawback, but the upside should be that the ore grade is more even and thus deplete less fast and require comparatively smaller price increases to scale. When I google it, it seems to be the case that nickel finds are low grade but very high tonnage, which seems to support my hypothesis. However, I'm no expert, so I'm speculating a bit here.
I'm currently working in the metals recycling business, a business I like to call, urban mining. Yes, Ni isn't cheap but I'd like to see increases in all forms of metals recycling long before I'd wish to see any kind of mining rate increases.
http://www.metalprices.com/FreeSite/metals/nickelalloy/nickelalloy.asp
LB MT KG
Nickel Prices and News
Nickel Premium
Truckload Quantity $/KG
LME NI Officials $/KG
C $21.225
3M $21.225
It may be also be an interesting educational experience to look at the scrap auctions listed at Government Liquidators.
http://www.govliquidation.com/scrap.html
I'm all for "urban mining", but nickel is a worst-case scenario in that regard. You can gather truckloads of things made from aluminum, steel, or copper and melt 'em down to make new ones, but you can't collect a truckload of nickel. It's a few percent here, a thin coating there, and it takes a ton of labor and energy to purify it out of recycled stainless steel countertops and scrape it off plated exhaust pipes.
Yes that is correct, however my point was that if we recycle more of those alloys which already contain nickle, then there will be less need for mining it.
"It's a few percent here, a thin coating there, and it takes a ton of labor and energy to purify it out of recycled stainless steel countertops and scrape it off plated exhaust pipes."
We get paid really good money for scrap 800H (32% nickel) so your point is well taken. The good news is that most stainless steel is recycled back to stainless, so there is no need for purify the nickel back to virgin state. Those hand-held X-ray fluorescence guns are a big help is recycling, as you can sort the various grades on the spot and remelt like to like. (Separate out the 304 and remelt it to more 304, without worrying about moly contamination from 316 or having some 309 throw your ratios off, etc)
Good to know you guys have some good high tech to help with that problem. In the very long run, we're going to have to be able to recycle almost every element in the periodic table, and humans create some really complicated mixes of elements that are pain to separate.
But the topic of discussion is increasing our rate of production of elemental nickel from current rates of about 1 MT/year to 10 MT/yr so we can make batteries. Recycling nickel alloys is great, but it doesn't create new elemental nickel. It might let you use a good fraction of the current 1 MT/year for battery making, but it can't help with the other 9 MT/yr you need.
If we can get a pratical magnesium-sulfur battery, as recently demonstrated by Toyota, the materials-scarcity issue goes from "right now" to a long, long way down the road.
Batteries are like PV: there are an enormously wide range of chemistries and designs, using a wide range of materials.
This makes both resilient: if one or more materials become difficult to find, substitutes will be easily available.
I name this fallacy "appeal to diversity".
What makes you think it's a fallacy??
We have an ongoing discussion about this elsewhere in this thread, right?
Not about battery and PV chemistries.
Now, if you're talking about the concept that a diverse set of sources of electrical generation allows a much more optimal solution than pushing any one source too far...well, that's just basic grid management. That's old, proven wisdom.
That's nonsense. Sure, you have coal/nuclear as base and hydro/gas as peaking, but other than that, diversity is more of a necessary evil in the face of resource limits, not something that helps grid management. In terms of grid management, intermittent sources are pollution, and nobody is interested in diversifying costs - we all like costs to be as low as possible. If we need to diversify, that means the best options aren't scaleable as we'd like.
Regarding chemistries, diversity here is nonsense too. The article claimed the most practical chemistry's resources (of lead) was 2 orders of magnitude too small. Do we want to take the next 200 less practical chemistries in order? No. If you can't present the solution, you have no solution. Hand waving doesn't cut it.
Jeppen,
That's nonsense. Sure, you have coal/nuclear as base and hydro/gas as peaking, but other than that, diversity is more of a necessary evil in the face of resource limits, not something that helps grid management.
You have list 4 different fuel types, you could also add diversity of nuclear designs, OCGT gas for peaking and CCGT gas for medium demand, and for hydro, we have run of river, short term storage(weeks to several months) and long term storage and as well pumped hydro.
Both wind and solar PV add additional diversity by geographic locations. PV can certainly help reduce grid peak summer loads.
If we need to diversify, that means the best options aren't scaleable as we'd like.
Gas peaking is definitely not the lowest cost option but is very flexible and has low capital costs but high operating costs. Nuclear, wind and solar all have high capital costs but low running costs. THERE IS NO ONE BEST OPTION.
The article claimed the most practical chemistry's resources (of lead) was 2 orders of magnitude too small.
But other chemistry's have much much larger resources; eg sodium /sulfur, but lead acid is more practical for starting ICE's.
Capital/running costs together with some other parameters gives a single Levelized Energy Cost per energy source. One of those is the lowest, and thus the best option in economical/technological terms.
"But other chemistry's have much much larger resources; eg sodium /sulfur,"
Case in point: Then THAT should be the argument, with some sort of quantification. Not just handwaving that there are several other chemistries that could be used when the lead runs low.
One of those is the lowest, and thus the best option in economical/technological terms.
That's a very puzzling thing to say. Nuclear doesn't make sense for peaking, and single cycle peaker gas units don't make sense for baseload.
As far as handwaving: if you want evidence, say so. If neither of us has time to produce it, then we'll just have to acknowledge that some of us have day jobs, and we can't do everything...
Actually, nuclear makes plenty of sense for peaking. Using nuclear heat to re-heat compressed air in a CAES system could double the instant thermal efficiency of the plant (discounting the off-peak electric power used to compress the air in the first place).
Nobody's done this because NG has historically been cheap, and so have the old, small, quick-to-start coal-fired plants. That's changing.
hmmm. Do nuclear plants really have that much waste heat? I guess I haven't heard of double cycle nuclear plants - why not?
CAES using reheats seems wasteful - what do you think of http://www.isentropic.co.uk/index.php?page=storage ?
LWRs are around 33% efficient. Nuclear heat is cheap, IIRC they don't usually bother with reheats or other economy measures.
If you re-heat compressed air at ground temps to 280°C (LWRs run hotter; the AP-1000 hot-leg temp is 321°C), you can potentially get over-unity heat-to-shaft-work conversion ratios. The compression side helps with grid management too; shutting down a 30 MW compressor in a 600 MW bank takes seconds, while starting a 30 MW gas turbine takes minutes. Any operating compressor can be counted as spinning reserve, eliminating the need to keep FF plants burning.
The isentropic pressure ratio for air (Cp/Cv=1.4, Cp specific heat 1005 J/kg-K) dropping from 550 K to 300 K is over 8:1, so a two-stage expansion engine with air input at about 80 bar and one reheat looks feasible to me as a SWAG. If a regenerator re-heats compressed air to 400K for the first stage and the two turbine stages receive air at 550 K with exhaust at 300 K (total heat input ~402 kJ/kg), isentropic expansion work assuming ideal gas properties and 8:1 pressure ratio (allowing for pressure drops) yields a theoretical 502 kJ/kg output. Turbine efficiencies these days get close to 90%, so over-unity heat to work ought to be possible. This would roughly triple the electric output of the plant versus the steam cycle; it would also eliminate the use of cooling water while operating on compressed air. (The cooling demand would be right back when the air reservoir had to be refilled, of course.)
Not worth it when SCGTs have low capital costs and used relatively cheap fuel. Switching heat from a steam generator to an air-heating loop would add complexity and testing burden, and would probably make the bureaucratic empire-builders at the NRC rub their hands with glee at the thought of all the people they'd be able to hire to go over the details.
This sort of thing would work much better with a molten-salt reactor; the MSRE dumped heat directly to an air radiator, and would have been a natural for a self-powered gas-turbine to supply the forced air.
I'm skeptical of the efficiency claims (a real-world isentropic machine?), and wonder how the waste heat is dealt with.
I like pumped hydro storage and only enough for one day with shedding of loads based on priority. Hospital hearth machine top priority, Walmart ad sign bottom priority. When the backup runs out we take a holiday until the wind or sun returns.
What a strange and pointless article. It is hardly a newsflash that lead-acid batteries are not suitable for utility scale energy storage.
If you had said "This article shows how lead-acid batteries are not suitable for utility scale energy storage" in the summary at the start I could have saved 10 minutes to read something more interesting.
One thing that bothers me with this article (and with TOD in general, btw) is that it is so US centric. Reasonable countries (industrialized non-North America) use 5 KW/capita, all energy. Say we do some negawatt and get it down to 3 KW. Times ten billion people, that's 30 TW. That should be the goal. The US isn't the world. The EU has a bigger economy than you do. Soon, China too. Eventually, India as well.
When you say "reasonable countries," you sound like you are onto a gross over-generalization. For example, California lives on a European type electricity consumption diet. They are 10% of the US population. Aim your scarlet "G" lutton onto the other states. ;-) But seriously, climate is a critical factor determining electrical consumption. People living in Northern Europe use more electric than those in the temperate southern parts of Europe.
Sure one day India and China will be the world's leading economies. That would require that the West falls more or less out of existence. Now where would China sell its goods -- to themselves? When that happens we will all be using sticks and stones and our bare hands to make things. So more people means more GDP ;-) One would not even need to worry about electrical consumption at that point I guess.
As I don't live in the US, I tend to consider the US a single country. :-)
Climate as a critical factor? Perhaps that's a part of it, but I would say that hydro resources may be another explanation.
Yes, China would primarily sell its goods to itself, and no, the West needn't fall out of existence.
Thanks for assessing the (non-)viability of lead acid batteries for electricity storage as a 100% solution for dealing with the intermittency of clear sky solar thermal power.
This may be a paper tiger. There may, for example, be other more thermodynamically direct routes to addressing the problem. Thermochemical conversion pathways could be worth a try - at the very least for lower temperature process heat. The Swedish Tepidus interseasonal heat storage system, if I remember correctly, offers not just time shifting but also space shifting - you can truck the potential heat around.
Who needs a National Battery? What we need is a variety of electricity "storage" (and storage-avoiding) options, including smart grid technology, and a flexible, resilient energy system based on multiple switchable electric and non-electric conversion pathways.
- Colin Moorcraft
Reserve power requirements for our aging and increasingly imperfect electricity grid is typically in the range of 12-15% of peak load. Why on earth would we want (or need) to run our electricity system continuously for a 7 day period with only stored energy?
Better storage and demand management often displaces the need for additional source generation (or new power plants). Given the cost of building new power plants to meet increasingly steep power consumption profiles (not from population growth but proliferation of consumer electronic devices and other demands), we appear to already have the money and resources to meet our current and future energy production and distribution needs. What we lack is political will (increasingly lacking these days), and the right incentive structure (regulation of utilities) to make this happen. So far as utilities make profits primarily on energy sales (rather than conservation and management … or a combination of both), we'll be stuck on the slippery slope of peak energy technologies and diminishing market share for baseload and other generating technologies that don't inextricably link volatile marginal costs to energy speculation and profits.
There's currently money to be made in energy storage (and lots of new developments and even game changers in the pipeline), but not as much as can be made in something like natural gas (if you know how the system works). Once again, a non-renewable energy resource with a large marginal cost is winning the day (and consumers will continue to pay a price … in both up front capital, environmental, and health consequences for generations to come). Energy storage, demand management, conservation, and efficiency will have to be left for another day.
I would argue that storage is not the solution. It is expensive and inefficient. Overbuilding a system, with excess capacity taken off-line (simply done with wind - just feather the turbines) when unneeded. This, however, would require large-region interconnection and regional, trans-state regulatory agencies to manage planning, maintenance and revenue distribution. Wind on the North American Great Plains is not an intermittent power source. What is missing, presently, due to the low population density, is the availability of 60 kV collector lines to allow for massive wind power development. Excess wind power could also be used for Haber-Bosch nitrogen fertilizer synthesis. The original method was developed for electric, not natural gas, power.
Wind on the North American Great Plains is not an intermittent power source.
Fred, that sounds reasonable, but have you seen hourly production data? I'd love to find it.
Significant errors in this article are, it doesn't consider alternative energy storage mechanisms, load management, over capacity factor of (2.5 to 3x) which is already required aspect of our grid, etc. (Plenty of room to conserve, the french use electricity @ ~1/2 the rate per person. )
http://en.wikipedia.org/wiki/List_of_countries_by_electricity_consumption
The current crop of modern combined cycle NG fueled power plants will operate on H2(hydrogen gas) somewhat more efficiently(5-10%) than CH4(methane), they can also run on any mixture of those two gases. With recent advancements in catalyst tech, the electricity to(95%) H2 to(65%) electricity cycle is near 60%. Which is just about as good as a new Pb, H2SO4 battery(~70%).
Note: We can also produce CH4 from atmospheric CO2 & H20 at ~60% when needed. http://www.iset.uni-kassel.de/abt/FB-I/publication/2010-036_Renewable_po...
This is one reason why NG fueled combined cycle plants have a bright future in a post carbon world. Why do you think electric co's are building them like there is no tomorrow.
Lastly, the amount of storage capacity for H2 is nearly unlimited(depleted NG fields, etc), thus there is no real limit to the available backup capacity.
The linked paper (3.5MB pdf) on synthetic methane makes a case for using the gas grid for longer term low leakage storage than batteries or hydro. They give 8 eurocents per kwh thermal for the cost. That doesn't jibe with $2.40/L or ~10 kwh thermal for liquid car fuel in Germany unless taxes are high.
They have to show this concept can be scaled up and that costs are affordable. If this is one of Germany's ideas behind the nuke phaseout it is a gamble.
For a supposed expert professor in his field he is surprisingly unaware of Beacon Power a company in Massachusetts which is currently operating and producing 25 Megawatt inertial battery power storage units which are scalable and repeatable. They are designed for a 25 year bearing life and have no emissions, toxic waste or other problems. I hope the good professor can further his education and give us a review of these kinetic batteries and their positive benefits for the future of power storage from multiple power sources. PS-they also help smooth out the grid. Hope this is some help.
Less snark and more links would be nice.
Beacon website here.
While these flywheels are good for many uses, I don't see the claim that they are useful for longer term, heavy duty storage such as overnight or extended cloudy periods. They look useful for short term intermittancy, grid regulation and smoothing, still a requirement for PV and wind.
BTW: Not sure where your 25 megawatt claim came from. From the site:
Perhaps you were refering to this press release:
Methinks they mean 20MWH storage or 20 MW peak (but for how long?). Impressive, nevertheless.
thats the least snarky I could do but you are right its better to be a gentleman. However when professors need to further their education whats a guy to do. You are also correct that the whole plant operates at 20 MW not 25 but as i said this is all scalable and repeatable and I also am trying to convince the company to open up their operating system to be more adaptable to various systems and voltages but so far have had no success with their bureaucracy. Until tomorrow, In solar I trust
"However when professors need to further their education.."
...they put their stuff out here on TOD, and good on'em for doing it. We'll do what we can to help ;-)
Beacon Reports and Papers
The friction losses in flywheels make them useful for storage only up to a few hours or tens of hours. Buffering RE for a week or more requires something which "leaks" less energy.
From the Beacon Site, Fact Sheet:
Note that the long section you quoted mentions absolutely nothing about the idle losses at various "charge" states. Efficiency under continuous cycling (regulation) with 15-minute charges followed by 15-minute drains will not be the same as efficiency carrying energy from Saturday to Wednesday.
Beacon's flywheels have *terrible* energy storage density. I did some math on 'em when they appeared on slashdot: making a rough guess at their mass, I calculate their energy-per-mass to be about 1/2 the humble lead-acid battery.
So, they're half as good at storing energy as a lead-acid battery, and they involve spinning high-precision carbon fiber machinery around in a vacuum chamber at twice the speed of sound.
No thanks.
Good point! Here's my $0.02:
"DOE’s new renewable energy Venture Capital unit ARPA-E has just funded an entirely new kind of liquid battery innovation from MIT professor Donald Sadoway, that works like an aluminum plant running in reverse; producing power instead of consuming it."
http://cleantechnica.com/2009/11/19/arpa-e-37-top-funding-goes-to-renewa...
http://web.mit.edu/newsoffice/2009/liquid-battery.html
Tom Murphy, when you do this calculation, please use realistic numbers for the amount of electricity that would need to be stored. The U.S. would not power everything from electricity generated from wind and PV. Solar thermal is better suited for some applications, such as heating water and houses. Hydroelectric and geothermal generators are not going to disappear. There are other ideas, like kite-gen, out there too.
If the national battery stores 7 days of power, then on sunny and windy days the turbines and PV would have to generate enough power for society and to charge the batteries. Wind turbines and PV would have to be overbuilt which in the case of PV would supply more than 25% of the sunny day power on a cloudy day. A minimal off-grid PV system is overbuilt by a factor of two, that is, on a sunny day the PV array outputs 2 times more power than one uses on an average day to provide power to charge the batteries. That means on a cloudy day the PV array outputs 40% to 50% of the average power used per day. For a minimal PV system, the national battery would actually last for 12 to 14 days when including the cloudy day PV power (assuming all PV and no wind). One could obviously overbuild PV by 4 or 5 times which would reduce the national battery to supplying overnight demand, more than 14 times smaller (7 days / 12 hours and there is less demand at night than during the day) than you calculated.
As others have commented there are other, less expensive, ways to store electricity besides using expensive batteries. Pumped hydro is the low hanging fruit. Designing thermal mass into refrigerators, freezers & air conditioners and adding automatic controls that only allow them to run on sunny days is easy. Solar hot water panels, a PV powered heat pump and a large thermal mass also works well.
The battery array in my off-grid PV system is sized to power my loads, such as my microwave oven, not to supply power during extended cloudiness. I do not deep cycle my batteries because I reduce my electric power consumption on cloudy days. I have 8 Crown L-16HC that store 8 * 6V * 395 A·hr = 19 kW·hr. My previous array lasted 14 years which contradicts your assumption of 5 years (abused, deeply cycled batteries last ~5 years). In 2005 I paid about $180 / battery. Now they cost $304 / battery. The national battery needed for "running a 2 TW electrified country for 7 days requires 336 billion kWh of storage," would cost $43 trillion, not "$25 trillion," assuming no discount for bulk purchases and ignoring the costs of wiring, tax and installation. With such a high price you can be sure people would be a bit more cost efficient by any number of means. Because PV panels are less expensive than batteries (Kyocera KD-240GX PV for $490 with durability of 30 years & price falling vs. Crown CR-395 battery for $304 with durability of 5 to 15 years & price rising), people will over build PV arrays before they will splurge on batteries. Batteries will be used for overnight and high power temporary loads, not to store power for cloudy days. This greatly reduces the size of the national battery.
A series of very good points. First, let me say that I frequently hear cavalier (reckless?) dismissals about how we will solve our future challenges. The more thoughtful ones usually start with "why can't we just...," but many are more certain: "well, we will just..." Maybe it's the word "just" that gets my goat. I realize that many of the readers on TOD are more sophisticated than this, so my approach may seem patronizing and pedestrian. While I am delighted that TOD is interested in picking up some of my material, the pieces are not written for TOD and its audience, but rather for a more general crowd to get them thinking about these issues. All the same, I value your patience and the useful comments generated.
A couple of specific issues: In order not to "abuse" the national battery with deep discharge, it too would have to be "overbuilt" by a factor of two or more. So reducing the need for battery storage with other options is compensated by the overbuilt battery, putting us back (near) where we started. I think you're right that batteries will likely find their niche for shorter term (overnight) storage more so than for long stretches. This is another way to say that it may not be practical to rely on batteries for our storage needs—reinforcing the point of the post.
I need to look into it more, but I am skeptical about statements that we will "just" use pumped hydro storage. The scale we need is massive. So even if we could identify enough high bowls (even if we need to complete some of the sides), how likely are we to transform our landscape at this scale? I've watched the raging controversy over a new power line to bring renewable power (and Mexican FF power) from the California desert over the mountains to San Diego. You want how many forested vales turned into lakes? Also daunting is the knowledge that massive hydro deployment already in place gives us 7% of our power (previously I used 3%, but this is compared to total thermal energy; 7% is more fair). Figuring that pumped hydro would be charging half the time and discharging half the time, we would need about 30 times the current hydro-turbine capacity installed. Makes me quiver. I'm not ready to smile and say we can just do it. Of course any real solution is a mix, but even the "low hangers" represent unprecedented challenges.
I'd like to see what could happen if you took your knowledge to investigate how geography and daily weather would effect the concepts of hydro-electric-based energy storage.
We don't have to do all the heavy lifting. The Earth's topography and other known constants regarding atmospheric convection cycles of water vapor offer clues to as to how "hydro/gravity" batteries could be created.
Of course - volume would be the issue - and much energy would be consumed maintaining such endeavors but it's probably the not as time-intensive as the current America's interest in "reality TV." :-)
Discharging the battery by 10% every day and then recharging completely yields a lifetime of about 19 years. Discharging half of the batteries by 20% yields a lifetime of about 9 years. Discharging 1/4 of the batteries by 40% yields a lifetime of about 4 years. To minimize the cost and number of batteries, the amount of discharge should be limited to less than 20% of the battery capacity. Therefore the capacity of the battery array should be 5 to 10 times the amount of electrical energy that is used during the night, provided the battery array thus calculated is large enough to power the maximum load.
To estimate the size of the national battery, I would calculate it as follows:
1. Determine the maximum historical power consumption during one night in the U.S.
2. Human world population is following a bell curve. Assume it will rise from the current 7 billion to a maximum of 9 billion. (9 billion) / (7 billion) = 1.29 or 29% increase. Assume U.S. demand for electricity will rise by 29% due to increasing population.
3. Assume the U.S. converts transportation to a combination of all electric vehicles, plug-in series hybrid vehicles and electric rail with the liquid fuel coming from biomass. This might increase electric consumption by ~20%.
4. Improvements in efficiency will probably balance the conversion of things that use natural gas to things that use electricity.
5. Coal is already used to produce electricity so its elimination does not increase demand for electricity.
6. Assume that electricity from wind turbines will not be stored in the national battery. Instead it will be stored in the form of pumped hydro and the creation of products that can be manufactured intermittently, such as ammonia.
7. Estimate how much continuous electrical power the U.S. could get from combining wind, pumped hydro and geothermal during the night. Estimate how much solar thermal to electricity with storage would provide. Subtract these estimates from the future estimate of total nighttime demand.
8. Assume PV will be overbuilt by 4 times to provide ample power in the daytime to provide the remainder.
9. Multiply the remaining nighttime demand by 10 to estimate the maximum size of the national battery with a lifetime of 19 years.
1. Wiki: List of countries by electricity consumption lists 3,741,485,000 MWhr/yr in the U.S. for the year 2009. That is 10.2 billion kW·hr / day. For lack of better data, I'll make a WAG that the U.S. uses 1/3 of that power at night making the estimate 3.4 billion kW·hr / night.
2 & 3. Maximum future U.S. nighttime electrical demand: 3.4 billion kW·hr / night * 1.29 * 1.2 = 5.3 billion kW·hr / night
7. EIA: Net Generation by Energy Source: Total (All Sectors) groups geothermal and solar thermal under "other renewables" and gives the conventional hydroelectric generation for 2010 as 6.24% of the total. Centralized solar thermal could create a lot of power, but I will assume that distributed PV will undercut it in price which limits its growth. I'll calculate the amount of energy using pumped hydro between Lake Superior and Lake Ontario assuming a 2 m change in water level in the smaller Lake Ontario.
Lake Superior:
surface area: 82,400 km2
volume of water: 12,000 km3
elevation: 180 m
Lake Ontario:
surface area: 19,500 km2
volume of water: 1,640 km3
elevation: 75 m
density of water: 1000 kg/m3
efficiency of pump: 90%
efficiency of generator: 90%
loss to water evaporation: 10%
mgh = 2 m * 19.5 billion m2 * 1000 kg/m3 * 9.8 m/s2 * 106 m = 4.1 x 1016 kg m2 / s2 = 4.1 x 1016 J = 11 billion kW·hr
11 billion kW·hr * .9 * .9 * .9 = 8 billion kW·hr
Surprisingly this is larger than the estimated 1 night national demand rendering the national battery unnecessary in the northeastern U.S. and even parts of Canada. Transmission line losses to distant locations would mount, so I estimate 30% of U.S. continuous electrical power could be supplied by conventional hydroelectric, geothermal, wind and pumped hydro.
9. Now the national battery needs to store:
5.3 billion kW·hr / night * (.7) * 10 = 37 billion kW·hr
which is 9 times smaller with a lifetime 3.8 times longer than your estimate of 336 billion kWh and 5 years. If the batteries are Crown L-16's, 2.37 kW·hr / battery and $304 / battery, the price is $4.75 trillion spread out over 19 years or $250 billion / year. With the number of houses increased by 1.29 from 110 million to 142 million and 70% of the population having the batteries, there would be 157 Crown L-16 batteries per home. A residence can get by with much fewer (I have 8 batteries), but street lights, industry and such will be drawing their share from the national battery.
The 5 trillion kg of lead that you estimated is now reduced to 556 billion kg or 612 million tons. The estimated global total resource of lead of 1.5 billion tons is sufficient to construct the national battery, but we should still reduce our consumption, improve efficiency and shift our demand from the night to the day to reduce the burden and cost.
Thanks for the graph. One thing that is very interesting to note about it is that the amount of energy cycled through the battery in its lifetime is much more nearly a constant. 5% discharge at 15000 cycles gives 750 times the battery's total capacity; 10% --> 700 times; 20% --> 660; 50% --> 575; 100% --> 500. So you get somewhat more out of a battery set by light discharge, but then for a given system you need to buy a bigger battery with larger up-front cost. For this reason, I opt at home to drive my batteries hard (50% discharge daily) rather than have the increased space and maintenance of a larger set.
I did the same calculation you did, and was astonished by the pumped hydro potential of the Great Lakes. It makes my earlier schemes involving running the Colorado River backwards look like small potatoes.
Not as much as you'd think. Transmission losses for a HVDC line are around 3.5% per 1000 km. San Diego, the continguous US city furthest from Niagara, is 3500 km away, so the expected transmission loss is 12%. I'd call that loss acceptable.
But Great Lakes pumped storage doesn't have to power the West. Almost all existing US hydropower is already there. It amounts to only 7% of US electricity flow, but it's about 30% of *western US* electricity flow. If we choose to run these hydro plants at 3x the output, 1/3 of the time, they could act as a "battery" for the entire western US. We may need to add new turbines to existing dams, of course, and the environmental consequences to the Colorado and Columbia rivers will be severe. But perhaps that's worth it.
I think you're selling pumped hydro short. I just demonstrated that hydro can cover for wind/solar intermittency in the western states: your math showed that Niagara pumped hydro can cover the central and eastern states. There really is enough water to provide *all* of our stored energy needs, not just 30%.
Anyway, back to Niagara Falls. If we really did build a pumped hydro system there capable of powering the eastern and central US, what would it look like? At peak flow, it'd be generating or storing 0.4 terawatts of power, which, given your figures, means 0.4 million cubic meters of water must flow through it per second. That's about 200 times the natural flow of the Niagara River. So good news: we can afford to keep Niagara Falls flowing as a tourist attraction, it'd be just a trivial leak in the system. Make sure to get your picture taken next to the 100-meter-diameter penstocks as you drive back home!
Probably the biggest problem with this plan is not a lack of energy or materials or money: it's that you have to send the entire country's electricity flow through one spot. This means that things you'd never think of, like dealing with the 10% waste heat from 90% efficient motors and generators, become a huge issue.
And lord help us if Canada ever declares war on us.
Motors and generators in the size range you are talking about are 99%+ efficient.
Pick-Sloan seems like the place to start for massive pumped storage, plus wind, plus long distance DC transmission.
Thinking about pumped storage in terms of replicating conventional hydro is missing the point. Conventional Hydro typically has much larger storage capacity per MW than what you are talking about. In other words, you might need 30 times the turbine capacity (although that'd be double the existing electric grid capacity so I think you've missed something), but you need a lot less dam capacity. For instance, the Colorado dam system has years worth of storage at the conventional flow rates. You are talking about a week's worth of storage. In other words, you could (theoretically) add 30 times the turbine capacity to the existing reservoir, if you had a big enough second reservoir. You usually don't have a way to do that much, but the need is definitely not equivalent to replicating the existing infrastructure times 30.
Batteries are very long known but also old fashioned.
Check out this really huge storage possibility:
http://www.ringwallspeicher.de/
(sorry, only german)
The next step that has become feasible in experimental installations is not the creation of H2 that is difficult to handle but the generation of CH4 from surplus renewable energy.
On the german wikipedia you find it here:
http://de.wikipedia.org/wiki/Windgas
We can do it if we try, but we must do it now, that is the only problem ;-)
The upper reservoir is an artificially created lake which is expensive to construct. The floating PV array reduces water evaporation and might reduce the energy needed for tracking. The artist seems to be thinking only of centralized power generation because the houses are orientated in random directions and do not have PV or solar hot water systems on their roofs. Although the text indicates there are grid-tied PV systems on the roofs. Since the wind turbines located around the parameter of the upper reservoir are already elevated 215 m, I do not see benefit from attaching them to tall poles. I think maximum air speed would occur near the rim of the upper reservoir, not 100 meters above it. Maybe the air flow around the upper reservoir needs to be modeled.
The big question: How much does it cost?
As a person who does not believe that it is remotely possible to build an all RE energy source in the first place, if you are going to go with far out ideas why not choose possibly the cheapest and best solution.
This being no storage at all, just a world wide connected grid with solar power coming from the various deserts around the globe as the earth rotates. Might have to have a fair bit of wind as well for when the sun is above the Pacific Ocean in the northern winter.
Of course the resources needed to do it would be massively beyond anything produced at present, which means a ramp up in resource development, which of course means higher energy use which means even more resources, for the higher level of energy needed, .....oops we're in a spin, just like every other idea, unless you don't do the sums of course.
The discussion here is focused on scaling up known quantities to large scale. But long-distance power transmission across oceans is a very difficult problem for which we have no good answers yet.
Also, the Pacific ocean is very, very big. When it's noon in Kiribati, the sun is on the horizon in both California and Australia, so even a globally linked solar power network will have a few hours hours of zero power each day.
Actually transmitting power under-sea is a mature technology with hundreds of examples around the world.
Vancouver Island to mainland Canada, Netherlands to Norway, and UK to Europe are some existing examples.
Capital requirements rather than technical issues are the main impediments to expanding sub-sea power transmission, although many projects are in either construction, planning, or proposal stages.
A sub-sea cable to transmit geothermal power from Iceland to UK, and thence to Europe is currently proposed
http://en.wikipedia.org/wiki/Submarine_power_cable
"Longest
NorNed (between Eemshaven, Netherlands and Feda, Norway), HVDC, 700 MW, 580 km (360 mi)[3]"
I was careful to say "long distance across oceans". Your examples, even NorNed, are very short compared to what's needed to bridge the Atlantic. These problems can probably be overcome, but as I said earlier it requires future tech, not just massive deployment of modern tech.
"Also, the Pacific ocean is very, very big. When it's noon in Kiribati, the sun is on the horizon in both California and Australia, so even a globally linked solar power network will have a few hours hours of zero power each day."
Which is why I stated that we would need a large amount of wind as well.
There will be very high capital costs in linking up the world, yet everywhere coming up with their own nation sized battery would be very expensive. My opinion is that we have nowhere near enough resources to do either, so we may as well dream about what would be 'best'.
Does anybody know anything about diborane. It looks like it might work in with the wind or solar storage issue.
All boranes are ridiculously toxic. All are unstable, tending to break down spontaneously into the elements. Diborane, the lightest of them, tends to ignite spontaneously if leaked into air. This could be good if it always did so -- explosive fuel-air mixtures could not accumulate -- but this hypergolicity isn't guaranteed.
I know this: diborane is such a scary chemical that rocket scientists scream in terror when someone suggests using it as rocket fuel.
It is lethal at parts-per-million concentrations, it explodes spontaneously if mixed with air, and it continues to burn in most common fire extinguisher gases (pure nitrogen, halon, or CO2). And when you burn it you get a *solid exhaust* which sticks to the inside of your engine.
Diborane is in the running for the worst fuel known to man.
I ran into a plausible solution (PDF, you may need to search down for "gigawatt-season"), for CSP anyway, when I was looking for thermal processes that separate the B and the O in B2O3. (As with H2O, brute force doesn't work: the oxygens and the other atoms are, when the gas is hot enough, free, but there is no obvious good way to unmix them at that temperature, and on cooling they stick together again.)
Maybe you could summerise this article for the chemically challenged.
Wind and solar plants don't work full-time. If you wanted to run the United States using batteries when there was no wind and no sun, you'd need an impossibly big battery. For a lead-acid battery like the one in your car, there isn't enough lead in the world to make it work.
Replies to the article have suggested we can make do with a much smaller battery, or we can use batteries that use more common materials than lead, or that we can store energy in other ways than batteries.
Does anyone have an opinion on the VADIUM REDOX BATTERY? This system was previously owned by
VRB power company in Canada - but I think they went bankrupt and the system was bought by
PrudentEnergy. Here's their website: http://www.pdenergy.com/
Years ago I was in periodic contact with VRB, asking about a 5KW sized sytem for home use...but
it never happened.
The energy density of the vanadium solutions is low, leading to high losses in the systems pumping fluids through the active part of the flow battery.
There's been mention lately of using colloidal solids in a liquid carrier to increase the energy density.
We’re not a nation tolerant of power outages.
Actually, we are - we just want notice. It's called Demand Response, or Demand Side Management.
A rule of thumb for remote solar installations is that you should design your storage to last for a minimum of three days with no energy input.
That's off-grid. That's very different, and doesn't apply to the overall grid.
If we transition everything to electricity, we can get by with 2 TW, assuming no growth in demand.
This is a very big error: we could get by with only 1TW. For instance, oil for personal transportation currently consumes about .6 of the 3 TW of primary energy: that could be replaced by about 100GW of electricity.
This post deals with the narrower topic of what it would take to implement a full-scale renewable-energy battery. Scale the result as you see fit.
That's a bit misleading. If we only need a battery 1% as large, the problem is very different.
lead-acid batteries are the cheapest way to store electricity today.
Not true. LA is berst for an application with a medium large number of discharges. Li-ion and pumped storage are better for a very large number of discharges. There are alternatives for applications like seasonal renewable backup that are far, far cheaper.
about 15 kg of lead per kWh of storage...We would do well to push for future improvements on this score
Firefly Energy has reduced that by about 50%.
...336 billion kWh of storage...15 kg of lead per kWh of storage...$25 trillion price tag...today’s deep cycle batteries retail for four times the cost of the lead within them
That's an assumption of about $150/kWh for retail batteries, and $75 for utility scale pricing. I'd say that's about 50% too high for both.
We can’t just scale up the current go-to solution for renewable energy storage
Again, LA really isn't the "go-to" solution. The go-to solution is an integrated grid with diverse energy sources, with substantial over building and very cheap backup for very rare periods of low production from primary sources.
large scale energy storage is not a solved problem.
That's because it's very, very far from the best solution.
We should be careful not to trivialize the problem, which tends to reduce the imperative to work like mad on establishing adequate capabilities in time (requires decades of fore-thought and planning).
We should be far more careful not to exaggerate the problem. Our primary problem is political opposition from legacy industries to a conversion away from fossil fuels. Exaggerating the technical problems only helps them.
"This is a very big error: we could get by with only 1TW."
But the US has quite few people. Scale by 20 to get global requirements.
"The go-to solution is an integrated grid"
20% added cost?
"with diverse energy sources"
100% added cost compared to using the cheapest option?
"with substantial over building"
50%?
"and very cheap backup"
Thus very little? Added cost is what, 25%?
"for very rare periods of low production from primary sources."
These can only be rare if you rely very heavily on DSM, right? Added cost for DSM, 50%?
Total added cost, what? 200%?
Cost comparisons depend on your accounting and your benchmarks. If you include external costs, fossil fuels even now are rather more expensive than wind and nuclear. Wind and nuclear costs are likely to fall, while FF costs rise.
The current US grid is rather overbuilt, because utility regulated profits are based on capital expenditure ROI. If you don't build, you can't increase profits. So, we're overbuilt.
DSM is very cheap. Heck, you can build it into appliances for pennies with a simple voltage/frequency sensor.
Very cheap backup can include something as cheap as diesel generators, if they're used rarely enough. Solid biomass, like wood, is pretty darn cheap and practical if you only use it to supply 2-3% of the grid's kWhs.
A rational non-FF grid could easily be cheaper than the current regime.
"Cost comparisons depend on your accounting and your benchmarks. If you include external costs, fossil fuels even now are rather more expensive than wind and nuclear."
I was talking about diversified renewables compared to cheapest-option-renewables.
"The current US grid is rather overbuilt"
But perhaps not where it counts? You need a strong backbone to haul wind. Btw, there are some losses in long distance transmission too.
"DSM is very cheap."
Of course it is, since you take low-hanging fruit first, but if you want to scale it to allow for intermittent renewables, costs are going to soar.
"Heck, you can build it into appliances for pennies with a simple voltage/frequency sensor."
I likely want my toast and my tea/coffee not be dependent on wind. I'm sceptical about pennies for a practical solution. And it will increase complexity, sometimes needs interfaces for human intervention/tuning, will sometimes fail and need reparations and so on. And it won't go such a long way. Also, monitoring and tuning by humans is a cost, in a way, even if it just eats into leisure time. To turn on-off more times increase wear-and-tear and decrease efficiency and readyness. To cycle food temperatures in your freezer will affect storage time. And so on.
"Very cheap backup can include something as cheap as diesel generators, if they're used rarely enough. Solid biomass, like wood, is pretty darn cheap and practical if you only use it to supply 2-3% of the grid's kWhs."
That is unrealistically little, if you don't have unrealistic amounts of DSM. That's the problem with you "renewables diversity" guys - you muddle the fact that we don't have any working solutions by shooting off a dozen methods and technologies that don't scale in isolation, and then claim they scale together. That's pure hand-waving, AFAIK.
As I have mentioned before, the hope that is raised by handwaving solution diversity and future smart grids is THE political obstacle to nuclear power in much of the world and the best alibi for continued coal use. France decided to get rid of FF in electricity generation by nuclear power, and did it. That's the only success story we've had, and they did it 1973-1992. Germany, Denmark and others has tried renewables route since then, with little to show for it.
Jeppen,
That's the problem with you "renewables diversity" guys - you muddle the fact that we don't have any working solutions by shooting off a dozen methods and technologies that don't scale in isolation, and then claim they scale together. That's pure hand-waving, AFAIK.
1)Hydro and pumped hydro suitable for short term back-up(several hours to weeks), but cannot scale to >30% electricity generation( presently 20% world-wide). Requires long distance transmission from remote locations.
2) Wind provides >20% electricity in several small regions(Iowa, Denmark, South Australia) but may be difficult to scale >50% of generation. Main problem low output for short periods in summer (1-10 days). Requires long distance transmission.
3)Solar PV provides 50-100% of a few individual households and businesses, could scale to supplying 50-100% of most homes and workplaces world-wide. Main problems, no output during nigh-time, low output in winter-days and reduced output on cloudy days.
4) Geothermal, works well in some locations, would not scale to regions very remote from geothermal resources.
5) Nuclear, provides 13% of electricity world-wide not suitable for many small unstable countries and has been shown to be problematic where located close to large populations, but can be solved by locating in regions of low population density. Main problem is capacity to build rapidly and the need for hydro or FF peaking
6) Solar CSP with short term storage. Requires locations with low cloud cover, could scale to provide >50% energy use. Main problem is cost and the best sites are remote locations (deserts) requiring long distance transmission to populations.
The common factor is the need for long distance transmission and hydro back-up.
France decided to get rid of FF in electricity generation by nuclear power, and did it.
With the help of hydro and pumped hydro and sharing a much larger EU grid. Just as Denmark has replaced 23% of its coal-fired generation with wind.
the hope that is raised by handwaving solution diversity and future smart grids is THE political obstacle to nuclear power
I think cost overruns, many nuclear accidents, have been the major obstacles. On the other hand political opposition to coal-fired power has prevented many new coal-fired plants being built.
To 1, 5, and 6 you can add under problems, rabid opposition from environmental groups.
That's also becoming a problem for wind (#2) and transmission lines as well.
I think environmental resistance to CSP is very rare, despite a problem in California.
I think the US farmers who are the primary lessors of land for wind farms would be happy to accommodate all of the possible demand.
I disagree with much of what you claim, but I do think your list emphazise what I said: You have no real solution, and the hand-waving you present is expensive.
A few observations:
* Conventional hydro is mostly following load and cannot be diverted to following wind.
* Denmark et al has MORE problems with balancing 20% wind than France has with 80% nuclear. I have seen no region with little imports/exports claim 20% wind.
* Solar PV is extremely expensive. Germany has had to have FITs with 20-30 euro cents/kWh to get it built.
* Nuclear actually scales to close to 100%, which the intermittent stuff doesn't. Its inapplicability to small, unstable countries is no big problem, as those doesn't need much energy anyway.
* Nuclear has had problems, but those would be of no real consequence in an AGW-serious world if coal didn't have alibies, if renewables didn't present a straw to hold on to.
My question is: How long are you going to hold on to that straw? Coal is expanding 4% per year. France had a complete proof in 1992 that nuclear can replace FF electricity in two decades. Now, two decades later, Germany's and Denmark's ways are still very costly but uneffective. They could have been done replacing coal by now. How many more decades of renewables can the climate handle?
Jeppen,
If only environmentalists had the power you're suggesting!
Now, on to details:
Conventional hydro is mostly following load and cannot be diverted to following wind...Denmark et al has MORE problems with balancing 20% wind than France has with 80% nuclear.
Those don't sound quite right. Do you have evidence for them? France doesn't really have 80% nuclear: it has 60% domestic nuclear consumption, and 20% nuclear exports.
Solar PV is extremely expensive.
It was. Getting less so.
More...
"If only environmentalists had the power you're suggesting!"
In Europe, I think a majority is environmentalists in one way or the other. If established parties would have disregarded green issues, the Green parties would be way bigger. Instead, big established parties triangulate and adopt green issues to avoid losing votes. Despite this practice, green parties sometimes possess swing votes in European parliaments. In Sweden, Barsebäck nuclear power plant was decommissioned due to that.
"Those don't sound quite right. Do you have evidence for them? France doesn't really have 80% nuclear: it has 60% domestic nuclear consumption, and 20% nuclear exports."
This isn't poker, you don't win by bluffing. Look it up in the CIA World Factbook:
Denmark: electricity exports 33%, imports 37%
France: electricity exports 11%, imports 2%
Solar PV is still extremely expensive. It is so far from being reasonable that it is doubtful that it will ever be. I talked about a 100% price premium for doing diverse renewables (compared to just wind), and that's because I disregarded PV altogether in favour of CSP and other stuff that seems at least remotely viable.
I'm not deeply familiar with European energy politics. I have the impression that the Green party got most of it's start in the anti-war movement in the 1980's, which was focused against...nuclear weapons.
The arguments on both sides of the nuclear power issue seem hopelessly muddled to me. Personally, the link with weapons and weapons proliferation seems like the only real problem with nuclear power, and it seems essential to me to deal with it. Until that happens I foresee very disappointing progress.
Yes, I know Denmark has a higher percentage of imports and exports. It doesn't seem important to me - I don't see Denmark as the poster child of wind power. Further, Switzerland imports electricity from France, Germany, and Austria (about 50% of all the electricity that the country needs), while it exports to Italy about 60%. These are high percentages - do they tell us something about the success of Switzerland's energy program?
Sure, a country could be run on nuclear alone. Is that optimal? Very far from it. French reactors have capacity factors far below optimal and they'd be much lower still if France relied solely on nuclear power.
Solar PV is still extremely expensive. It is so far from being reasonable that it is doubtful that it will ever be.
Nah. PV continues to decline quickly in both cost and price - it clearly can compete in certain locations. Is it close to competing in northern countries? Not so much. What does that tell us? That energy nationalism is expensive.
As I've mentioned before, I don't see proliferation as something that concerns commercial nuclear at all. We don't need to beat that horse here, though, that would be off topic.
Then what is the poster child of wind power? Where's the success story?
"Sure, a country could be run on nuclear alone."
Hey, the fact is that 20% wind is at least as hard as 80% nuclear. Now you seem to want to imply they are equivalent since you don't do any of them to 100%?
Regarding PV, the last I've seen is that installed costs has started to level off at a point that is still far from making economic sense, and if it ever gets to a reasonable price point, it will have the same intermittency problem wind does.
what is the poster child of wind power? Where's the success story?
We don't really need that. Engineers plan for new things all the time - heck, France didn't need someone else to be a poster child in order to proceed.
you seem to want to imply they are equivalent
Not equivalent, just not as different as is sometimes suggested.
installed costs has started to level off at a point that is still far from making economic sense
I'm seeing under $3/Wp including all costs. In the right areas $3 can produce $.15/kWh, which is competitive for peaking power. I'd say $2/Wp is clearly achievable, which is extremely competitive.
it will have the same intermittency problem wind does.
It will have very different intermittency problems. It has large variance from day to night and summer to winter, but that's entirely predictable. In the right places it has very little unpredictable intermittency - that's much harder to deal with.
Ok, so we don't need a wind success story? Good, since there isn't one and we're not going to get one either. We're only going to keep burning coal and dream about high wind penetrations.
"Not equivalent, just not as different as is sometimes suggested."
You try to cover up an extremely important difference by saying neither is perfect.
for peaking power
As I mentioned in another thread, all power produced by PV can't be regarded as peaking, and all peaking power can't be covered by PV. It still doesn't make economic sense, and by trying to hide behind peaking prices you also say that it shouldn't be scaled very much.
"It has large variance from day to night and summer to winter, but that's entirely predictable."
That helps a little, but still makes the scale very limited.
Good, since there isn't one and we're not going to get one either.
Good thing no one said things like that to France's planners.
You try to cover up an extremely important difference by saying neither is perfect.
It is somewhat important, but not nearly as important as you're thinking.
all power produced by PV can't be regarded as peaking, and all peaking power can't be covered by PV.
True - some peaks are in winter, and even the A/C peak doesn't match PV generation perfectly. OTOH, it really wouldn't be hard to move the daytime peak a little earlier: just create price incentives. As you know, price incentives work quite nicely.
you also say that it shouldn't be scaled very much
Well, a significant amount of demand has moved to night time just to accomodate lower night time prices. That can move back. Price incentives really do work.
OTOH, I think it's very likely that trying to scale solar above say, 25%, would be suboptimal.
That nuclear, a base load tech, would scale to cover 75% of France's needs was obvious. That wind doesn't scale that way is also obvious. If someone would like to contest those assertions, the burden of proof is on them.
Regarding price incentives, they do work, but as I've mentioned, they don't come for free.
Ok, solar 25%, wind 25%, and lots of DSM, NG, HVDC, gasified coal and time to accomodate that. The climate is still f-cked. The only hope to go far enough (in GHG/AGW terms) with renewables seems to be that we somehow make it economically viable to synthesize liquid fuels with stranded electricity from extreme overbuilding of intermittent sources. But then again, high temperature electrolysis by nuclear power should be a much more rational basis for such fuels. And we don't have time. Nuclear could give us time, but will not, due to the vain hope of purer sources.
That nuclear, a base load tech, would scale to cover 75% of France's needs was obvious.
If it worked exactly as expected - which is always the point of having a "poster-child" demonstration project. On the other hand, they took a reasonable risk, and succeeded - which is the way things ought to work.
That wind doesn't scale that way is also obvious.
Actually, it's not at all. That it's harder is obvious, but how hard? A data point: anti-wind activists have been consistently proved wrong about the difficultiy of reaching various levels of market penetration.
If someone would like to contest those assertions, the burden of proof is on them.
Actually, there's quite a bit of research out there. Here's a sample:
"Equalizing Effects of the Wind Energy Production in Northern Europe Determined from Reanalysis Data". http://130.226.56.153/rispubl/vea/veapdf/ris-r-1182.pdf
It shows correlation coefficienof wind patterns across Europe between -0.2 and 1.
The total hourly outputs for Spain, Britain and Denmark show correlation coefficients of 0.08 (Spain and DK), 0.09 (Spain and the UK), and 0.32 (UK and Denmark). Barcelona (Spain) and Copenhagen (Denmark) are in the same time zone. They are separated by 14.5 degrees of longitude - about 500 miles.
This tells us that a modest separation (UK and Denmark) gives good independence, and a reasonable separation (Spain and DK&UK) gives almost complete independence.
So, as we add more countries, and connect wind resources at greater distances, the ratio of variance to mean output will continue to fall dramatically - the Law of Large Numbers.
solar 25%, wind 25%
Oh, my goal for 25 years might be 45% wind, 15% solar, 15% nuclear, 10% hydro, 5% biomass, 10% other: geothermal, wave, tidal, coal, NG etc.
due to the vain hope of purer sources
Again, that's really not the case in the US - coal and NG are here because utilities like them - period. Are you sure it's the case in Europe? I suspect that the fossil fuel industries have more political pull, and greens have less, than you're suggesting.
"If it worked exactly as expected"
What could go wrong? In 1973 when the French decision was taken, nuclear performance were quite well known. It was in their own hands to get it to 75%.
With wind, there are political issues in achieving full technical and economical grid integration across state and nation boundaries, and in pushing through smart grids with significant DSM all the way into all these countries' households. And this is just to push wind into the 20-40% penetration range.
"A data point: anti-wind activists have been consistently proved wrong"
I've never seen any anti-wind activists. There are skeptics, and there is NIMBY folks. But nobody attacks wind plants like Greenpeace attacks nuclear plants.
"quite a bit of research out there."
I think figure 8 is a bit depressing - wind generation for Northern Europe would keep within 50%-150% only 50% of the time. 20% of the time, it would be above 200% or below 25%.
"So, as we add more countries, and connect wind resources at greater distances, the ratio of variance to mean output will continue to fall dramatically"
Well, there is kind of diminishing returns here, as variance falls with 1/n, where n is the number of independent variables. And there is transmission losses. And, as I said, significant costs and political issues involved in making countries' energy security dependent on a grid backbone spanning entire continents.
"Oh, my goal for 25 years might be 45% wind, 15% solar, 15% nuclear, 10% hydro, 5% biomass, 10% other: geothermal, wave, tidal, coal, NG etc."
So, 60% intermittent sources, 20% baseload (all nuclear and half the hydro) and 20% dispatchable? You must really believe in DSM. I mean REALLY believe in DSM.
"in the US - coal and NG are here because utilities like them - period. Are you sure it's the case in Europe?"
I'm not sure about the US, but my understanding of the public opinion of Europe is that it is divided between nuclear and renewables, and that that division (along with the ineffectiveness of renewables) gives the coal interests their relative power. As long as politicians waste enormous amounts of money on renewables and the hope of high-penetration renewables is kept alive, loud environmentalist interest and a big green-leaning part of the public is rather content and won't say much about coal. Germany is a good example.
Regarding NG, the NG industry should love wind power. Every wind plant guarantees matching NG production for 20 years.
What could go wrong?
I'm sorry - is that meant to be ironic? Of course there were risks. They were manageable, and they paid off. Just as so many other engineering projects do things for the first time, based on well known engineering planning principles. Exactly the same thing applies to wind, and other relatively new sources of power.
With wind, there are political issues in achieving full technical and economical grid integration across state and nation boundaries
My goodness - are you saying that wind is more political than nuclear??
pushing through smart grids with significant DSM all the way into all these countries' households.
That's already law in the US.
And this is just to push wind into the 20-40% penetration range.
No, as we saw in the other study I gave you, 35% is achievable with the current infrastructure.
I've never seen any anti-wind activists.
Again, I'm having trouble believing you're serious. If you are, I can begin to understand your skepticism towards wind: you've been reading websites maintained by anti-wind zealots without being aware of it. OTOH, if you agree that wind is less political than nuclear, why would you suggest that it faces larger political obstacles at the beginning of your comment?
nobody attacks wind plants like Greenpeace attacks nuclear plants
You should read some of the stuff written by anti-wind activists in the UK.
figure 8 is a bit depressing
Unfortunately, I couldn't retrieve my own source(!) - something crashed. I'll try later...
Well, there is kind of diminishing returns here, as variance falls with 1/n, where n is the number of independent variables.
Actually, there's some negative correlations to be exploited - I'll have to find that study...
And there is transmission losses.
Not large. Long distance transmission is surprisingly efficient, and only a balancing fraction of the overall load would be transmitted.
And, as I said, significant costs and political issues involved in making countries' energy security dependent on a grid backbone spanning entire continents.
Perhaps the US has an advantage there. I note that Europe still hasn't integrated it's rail, so that trucks still carry most freight. In the US it's the reverse.
So, 60% intermittent sources, 20% baseload (all nuclear and half the hydro) and 20% dispatchable? You must really believe in DSM. I mean REALLY believe in DSM.
Don't forget that wind and solar have some negative correlation. Further, a modest amount of overbuilding eliminates a lot of the problem. OTOH, I do think that DSM is extremely useful. In a world in which we actually eliminate coal, we'll also eliminate ICE vehicles, and 20% of demand will be perfectly suited to DSM.
As long as politicians waste enormous amounts of money on renewables and the hope of high-penetration renewables is kept alive, loud environmentalist interest and a big green-leaning part of the public is rather content and won't say much about coal.
I'd say that really means that Europeans aren't really that serious about environmental concerns. That puts them solidly in the same zone as the US and Asia.
Every wind plant guarantees matching NG production for 20 years.
Not at all, though that's one way to do things.
Do you have any examples of this that you can point us to?
NAOM
hhmm..I looked in my notes, but I hadn't saved any examples.
I did a quick google search: I'm encouraged by the difficulty I had finding good examples of bad web sites.
I think this is one: http://www.savewesternny.org/index.html
The first thing you see: "Wind "farm" madness"! They even put "farm" in quotes, as if make it clear that there are no cute little animals here, just "industrial wind". The horror.
-----------------------------
Use of the term "industrial wind" is a pretty good tipoff that you're looking at an anti-wind website.
"I'm sorry - is that meant to be ironic?"
No. Again, what could go wrong? You're hand waving again.
"My goodness - are you saying that wind is more political than nuclear??"
I'm saying a determined country can do 75% nuclear by itself, while a (smaller) country can't hope to do much more than 20% wind by itself.
"That's already law in the US."
Oh, good, you're all set then.
"you've been reading websites maintained by anti-wind zealots without being aware of it."
And you ask me if I'm being serious? As I said, anti-wind sentiments are much more mild than anti-nuclear. I guess you agree, since you talk about nuclear being more "political"?
"You should read some of the stuff written by anti-wind activists in the UK."
I was talking about physical attacks on plants by foreign activists. Do you have those for wind plants? They certainly happen for nuclear plants.
Not large. Long distance transmission is surprisingly efficient
I started off by mentioning a number of additional/external costs for renewables. They add up. 10% here, 50% there, 25% there and so on.
Further, a modest amount of overbuilding eliminates a lot of the problem.
No, it really does not. Overbuilding 10% doesn't help much when output is 25% of mean. You just go to 27.5%. Also, don't forget that wind destroys its own spot price and hand a great spot price to NG. High penetrations mean electricity prices will be close to zero when total generation is high. Overbuilding can only worsen that, and to raise the mean wind output also risks that NG and coal gets ramped for balancing reasons.
eliminate ICE vehicles, and 20% of demand will be perfectly suited to DSM.
Another faint hope of a distant solution. I don't question that it might be feasible, in a fashion, eventually, but I keep arguing that the big price swings you'd need to convince people to charge when the grid needs it rather than in a fashion that is optimal for battery health and readiness will impose a cost. All your suggestions cost, and those costs are external to the intermittent sources.
I'd say that really means that Europeans aren't really that serious about environmental concerns.
Perhaps not according to your standards. Of course most aren't revolutionaries or activists. If told that stuff is being taken care of, that there is a bright future ahead, and they have proof that something is done in the form of some pain in their wallets, they feel good and go on with the rest of their lifes.
Not at all, though that's one way to do things.
That's the way it is done. Germans plan to keep on doing that, as I showed.
Again, what could go wrong? You're hand waving again.
Well, one major risk is related to a fundamental problem of nuclear, namely scale. Historically the nuclear power industry has chosen GW scale plants, which have been complex to design, and time consuming to build. IMO this has raised costs and greatly extended the time between each generation of nuclear generation technology.
The effect for France: they chose to scale up a single design, which created a risk that a fundamental unanticipated flaw would be replicated in all of their new nuclear plants. Fortunately, the risk paid off.
Now, I'm glad that you have strong faith in engineering planning. Again, the risks were manageable, and they paid off. Just as so many other engineering projects do things for the first time, based on well known engineering planning principles. Exactly the same thing applies to wind, and other relatively new sources of power.
I'm saying a determined country can do 75% nuclear by itself, while a (smaller) country can't hope to do much more than 20% wind by itself.
Both wind and nuclear become more difficult for small countries. For instance, Ireland would love to go nuclear, but they've decided against it: just one plant tripping would create too much disruption of their small grid. It's worth noting that France is firmly embedded in Europe's grid: their costs would be much higher if they were to go it alone. IOW, they're not a poster child for nuclear independence, because they're just not independent. A projection of how they would handle independence would be just that: a projection, not a real-world demonstration.
anti-wind sentiments are much more mild than anti-nuclear. I guess you agree, since you talk about nuclear being more "political"?
I do, actually, except for relatively isolated exceptions, such as parts of the UK, and the affluent coast of Cape Cod (in the US).
I started off by mentioning a number of additional/external costs for renewables. They add up. 10% here, 50% there, 25% there and so on.
I think I'd have to use the term "handwaving" for that. Do you have evidence for that? I've given you studies showing trivial costs for penetration of up to 35% - that of course isn't direct evidence for what would happen at larger scales, but it's suggestive.
a modest amount of overbuilding eliminates a lot of the problem. - No, it really does not. Overbuilding 10% doesn't help much when output is 25% of mean.
It helps enormously for the kind of seasonal problem we're talking about here. Output won't stay at 25% of mean for a week at time: instead it might, at worse, be at 75% of mean for a month. 20% overbuilding would raise that to 90%, a level that's easily handled by a variety of other measures.
20% overbuilding would indeed be modest: remember that the US grid is 145% overbuilt.
don't forget that wind destroys its own spot price...High penetrations mean electricity prices will be close to zero when total generation is high. Overbuilding can only worsen that.
Nuclear does the same thing at night
...to raise the mean wind output also risks that NG and coal gets ramped for balancing reasons.
Keeping 5% fossil fuel wouldn't be terrible.
eliminate ICE vehicles, and 20% of demand will be perfectly suited to DSM. - Another faint hope of a distant solution.
Sadly, I kind've agree: we're not likely to handle either our AGW or our PO problems nearly as expeditiously as we should. Still, my point remains: a world in which we do a 100% non-FF grid is also a world that will got to 90% EVs.
the big price swings you'd need to convince people to charge when the grid needs it rather than in a fashion that is optimal for battery health and readiness will impose a cost.
I agree. I think those will be relatively small, but they will exist.
I'd say that really means that Europeans aren't really that serious about environmental concerns. - Perhaps not according to your standards. If told that stuff is being taken care of...they feel good and go on with the rest of their lifes.
Sure. That's how it's going in the US, as well - that's the shape that a general lack of social commitment takes in a democracy: opinion-makers in government and the media develop a plausible excuse to do little, and everyone else acccepts it. Of course, Asian governments don't consult their citizens quite as much.
most aren't revolutionaries or activists.
Exactly.
That's the way it is done. Germans plan to keep on doing that, as I showed.
Their purchase of NG isn't directly related to wind - it's just the easy way to replace the loss of nuclear. Again, Germany isn't really the place for cheap wind, not with 18% capacity factors (compared to 35% in the US). Spain could really use the exports...
I don't think the liquid-fuel synthesis step is necessary. Piping RE directly to roadways isn't all that hard, and slashes the GHG emissions even if FFs are used (compare CCGT efficiency to ICEVs). If the RE systems fail to come up to the plate, nuclear power drops in without changing the rest of the scheme.
Excuse my ignorance: RE?
Renewable Energy (aka electricity). Wire the roads.
Hmm, that is perhaps a better solution overall, but excarbates the problem of matching intermittent sources to demand. Synthetic fuel generation from stranded electricity, OTOH, would go a long way toward handling the intermittency problem.
Comparing the efficiency of electricity-to-fuel plus ICE losses to something like regenerative CAES, the CAES would almost certainly win any contest for a storage period shorter than weeks.
The stranded/excess electricity might be better used to convert waste carbon-based material to syngas. The syngas can be used to make fuels or synthetic materials. (This assumes that "stranded" energy is available close to the source material, which may not be valid. Un-stranding the energy by expanding the grid reduces this problem.)
This bore repeating. And the bold tag.
E-P,
I'd be curious to see evidence for that. I don't see it in the US. I don't see any evidence that utilities see wind/solar as the main alternatives to nuclear. They're pretty happy with coal and NG as the primary alternatives.
I'd say fossil fuel (coal & NG) continues to be used because it's cheap (to the utilities, anyway) and easy and utilities like it. Period.
Utilities don't. The public face of the environmental movement (pushed heavily by the gas industry, which is the main interest which stands to benefit) does.
The major nuclear operators seem to be doing a good business.
Highly variable prices for electricity (I am thinking 4 to 60 cents/kWh - $40 to $600/MWh) will both induce DSM and the most economical forms of peaking power.
Below 4 cents/kWh, storage should absorb the surplus. And 60 cents/kWh allows for a number of options for peak generation.
Perhaps the range is wrong (any better ideas ?) but I think the concept of highly variable prices (avg perhaps 11 cents/kWh) is sound, both from a supply and demand POV.
Best Hopes,
Alan
Makes perfect sense.
Combine price variation with automated industrial/commercial/residential response, and you have a very workable system.
Jeppen,
There are a number of useful things I can say in response to your questions, but the biggest is: neither the Original Post nor my response ruled out nuclear.
"The current US grid is rather overbuilt" But perhaps not where it counts? You need a strong backbone to haul wind.
I was really referring to generation plant, not transmission & distribution. It's worth noting that the BAU mainstream plan for the US adds a strong T&D backbone.
."DSM is very cheap." Of course it is, since you take low-hanging fruit first, but if you want to scale it to allow for intermittent renewables, costs are going to soar.
Not at all. Consider that in the kind of future we're discussing, all personal vehicles will carry large batteries, whose charging can be easily scheduled in an entirely unattended mode, and which will be able to provide a very large storage and V2G function.
likely want my toast and my tea/coffee not be dependent on wind.
I'm talking more about larger things: vehicles as discussed above; washing during the night (which is done now); lighting (which can easily vary by 30% without affecting utility); HVAC, etc.
it will increase complexity
Have you looked at cars lately? Washing machines? They all have quite a bit more computing power than the space shuttle.
monitoring and tuning by humans is a cost
Very little of that will be needed. Mostly it will be set at the factory, and finetuned a bit on purchase.
To cycle food temperatures in your freezer will affect storage time.
You don't raise the temp during high prices, you lower it during low prices.
2-3% of the grid's kWhs." That is unrealistically little, if you don't have unrealistic amounts of DSM.
A week of storage represents 2% of annual demand.
you muddle the fact that we don't have any working solutions by shooting off a dozen methods and technologies that don't scale in isolation, and then claim they scale together.
Heck, they all work and scale right now. They're not optimal alone, but they work just fine.
Germany, Denmark and others has tried renewables route since then, with little to show for it.
No, they really haven't. Denmark in particular wasn't trying to reduce fossil fuel consumption at all - they just wanted to reduce oil imports.
"Consider that in the kind of future we're discussing, all personal vehicles will carry large batteries,"
Please calculate the cost of cycling those, or putting off charging at the risk of not having enough juice if you need to go somewhere unexpectedly, or that rates actually rise even more while youäre waiting.
"Very little of that will be needed. Mostly it will be set at the factory, and finetuned a bit on purchase."
Dreams of simplicity. Reality may not be that good.
"You don't raise the temp during high prices, you lower it during low prices."
A higher temp differential increase power consumption quite a lot.
"A week of storage represents 2% of annual demand."
That's all well and good for a battery, which can be cycled. You talked about solid wood, now how do you cycle that? You don't expect the wind not blowing for just 7 days a year, do you?
"Heck, they all work and scale right now."
They don't scale very far, and that's why you're handwaving diversity and a battery of solutions to that lack of scaling. Depressingly enough, that handwaving will keep up hope and the coal alibi almost indefinitely, as it can't be disproved and it will always be too expensive to realize, so we're stuck.
"No, they really haven't. Denmark in particular wasn't trying to reduce fossil fuel consumption at all - they just wanted to reduce oil imports."
Again, this isn't poker, so stop bluffing. Wind isn't an oil competitor. Denmark was aiming at lowering carbon emissions from coal. Ineffectively, obviously.
Please calculate the cost of cycling those, or putting off charging at the risk of not having enough juice if you need to go somewhere unexpectedly, or that rates actually rise even more while youäre waiting.
If I'm charging overnight, it really doesn't matter when it happens. As far as running out of juice - that's what EREVs are for.
Dreams of simplicity. Reality may not be that good.
That's a design choice. We'll choose it if necessary.
A higher temp differential increase power consumption quite a lot.
Not really. A freezer at -30 degrees (C) is 55 degrees from room temp. raise the temp by 5.5 degrees and the losses will only rise by an average of 5% (10% to begin with, zero at the end). That, of course, would only require a roughly 5% price differential.
You talked about solid wood, now how do you cycle that? You don't expect the wind not blowing for just 7 days a year, do you?
This is a longer term project - you plan ahead a bit. And, again, we're dealing with seasonal questions that are fairly predictable.
They don't scale very far
And what evidence do you have for that?
it can't be disproved,/i>
Sure it can. Simulation using historic wind data is quite easy. No one has seen the need for it, that's all.
Wind isn't an oil competitor. Denmark was aiming at lowering carbon emissions from coal.
No, they really weren't. In the 1970's Denmark generated quite a lot of electricity and space heat from oil, and the wind and coal generation projects were intended to greatly reduce that. CO2 emissions were a very late addition to the program.
"And, again, we're dealing with seasonal questions that are fairly predictable."
Yes, but still 2% biomass doesn't help much in balancing high penetration intermittent sources.
"And what evidence do you have for that?"
What evidence do you have to the contrary? Have you ever seen a wind generation graph for a country or state? Could you explain why Germany is ramping expensive Russian gas if cheap wind could replace nuclear?
"Sure it can. Simulation using historic wind data is quite easy. No one has seen the need for it, that's all."
I generally mock conspiracy theorists, but perhaps the reason such simulations doesn't see the light of day is that it doesn't give the results you want? Another reason may be that it is too obvious that high penetration wind isn't economically feasible.
"In the 1970's Denmark generated quite a lot of electricity and space heat from oil, and the wind and coal generation projects were intended to greatly reduce that. CO2 emissions were a very late addition to the program."
Again, you're wrong. Coal was to reduce oil. Wind came later to reduce coal. They never managed to go above 20% even though politicians' goals were 50%.
2% biomass doesn't help much in balancing high penetration intermittent sources.
Remember, we're primarily dealing with seasonal problems here. Underproduction that adds up to a weeks worth of overall power consumption will be very rare. OTOH, diurnal or even weekly variation can be handled with very different strategies.
What evidence do you have to the contrary?
Again, we get back to the day job problem...
Have you ever seen a wind generation graph for a country or state?
I've seen quite a few. Shouldn't we presume that we both are arguing from some kind of knowledge?
Could you explain why Germany is ramping expensive Russian gas if cheap wind could replace nuclear?
I never said Germany has cheap wind. Your link didn't come through, so I can't comment further.
perhaps the reason such simulations doesn't see the light of day is that it doesn't give the results you want?
There's a reason you mock conspiracy theorists. Don't give in to the temptation now. Utility system operators have every incentive to plan properly - they wouldn't bury a negative study.
Wind came later to reduce coal.
Interesting. Happen to see a source for that?
2% biomass doesn't cut it one way or the other.
A new attempt at the Germany link.
Utility system operators may consider such studies strategic company information. Universities may not want to produce them if the result isn't expected to be in line with what you'd like to see.
Regarding a source for Denmark, please see this.
Yes, I see that Germany is talking about NG. Well, Germany has never seemed like the optimal place for windpower to me. If they want to eliminate nuclear, perhaps they should stick to exporting Mercedes and import power - Spain is probably the best spot for wind and sun.
That article does say that Denmark has been trying to replace coal with wind - it also says that the historic goals of the program were met.
-----------------------------------------------------------------
Here's a bit more data:
"The electricity grid in the western United States could support up to 35% of wind and solar power by 2017, without extensive additional infrastructure, according to the National Renewable Energy Laboratory (NREL).
The US Department of Energy’s research agency issued a study that said the target was “technically feasible” – but would require key changes in how the electricity network is operated in the mountain and southwest states.
Up to 30% wind energy and 5% solar energy penetration could be achieved on the grid with a better coordination of utilities’ distribution activities across a much wider geographic area, the research suggested.
It also recommends operating a schedule of generation or sales more frequent that the current hourly system.
This would allow the system to react to the changes in transmission level from wind or solar projects.
Dr. Debra Lew, NREL project manager for the study, explained: “If key changes can be made to standard operating procedures, our research shows that large amounts of wind and solar can be incorporated onto the grid without a lot of backup generation.”
“When you coordinate the operations between utilities across a large geographic area, you decrease the effect of the variability of wind and solar energy sources, mitigating the unpredictability of Mother Nature,” added Dr Lew.
Western US
The Western Wind and Solar Integration Study offered a first look at how a significant amount of renewable energy could be integrated into the grid in the western US.
It followed a study published in January on the impact of wind farms on the grid east of the Rockies (see this BrighterEnergy.org story).
The Western study looks at the power system operated by the WestConnect group of utilities in the mountain and southwest states.
This group includes Arizona Public Service, El Paso Electric Co., NV Energy, Public Service of New Mexico, Salt River Project, Tri-State Generation and Transmission Cooperative, Tucson Electric Power, Western Area Power Administration, and Xcel Energy.
The research stated that were these utilities to generate 27% of their electricity from wind and solar sources across the Western Interconnection grid, it would cut carbon emissions by 25% to 45%.
It could also decrease fuel and emissions costs by 40%, depending on the future prices of natural gas, the study claimed.
The study called for better use of wind and solar forecasts by utilities, and pointed out that more efficient use of the current grid infrastructure would mean less new transmission systems need to be built."
http://www.nrel.gov/wwsis
"Up to 30%"? Ok, well, good for the US, but we're still talking <50% renewables. That won't save the climate.
1st, they're talking 35% for both wind & renewables.
2nd, look closely: they're talking about what can be done easily with the current grid. No DSM, no storage, very little additional transmission, etc, etc.
Again, they're looking at the planning needed for the medium-term. A grid of more than 35% renewables is so far off that analyzing it is just a waste of time for a utility working group (people who actually do this for a living). That's the province of geeks like us.
Nick,
It seems that a popular method of dismissing renewable energy solutions to peak oil or even peak FF is to imagine what would be required to replace ALL of todays FF use ( and replacing all nuclear) and to envisage absolutely no changes in our present life styles.
A good example is the use of FF oil, which accounts for about 40% of US energy consumption. If we set aside for now the 15% which cannot be easily replaced( some chemical synthesis, essential air and ocean transportation) we basically have to replace most oil based land transportation and some home heating. As you have pointed out the 0.6TW used for personnel can be mainly replaced by 0.1TW of electricity. Replacing the other 0.4TW would possibly require another 0.1TW electricity and a small amount of biofuels(methanol, ethanol or biogass) assuming some type of PHEV to give flexible range for trucks and off-road equipment(tractors, cats etc).
So what about the 15% of oil use that cannot easily be replaced? If the economy is only using 15% of present oil use we can tolerate a much higher price. A lot of airline travel ( budget holidays) just will no longer be used. Some chemical synthesis will use alternatives such as electric arc acetylene, or the products replaced by non- FF substitution so its likely that 15% of FF oil used will decrease dramatically and the few essential uses will use it much more efficiently(for example sea transport at lower speeds).
I would call this BAU, just as our transition from wood to coal or coal to oil was BAU although it involved a complete re-organization of our economy.
I agree.
It seems like we need a new word, doesn't it? We're talking about a future where we largely eliminate fossil fuels and dramatically reduce our environmental footprint, but which is also comfortable and workable.
BAU doesn't sound quite right.
Not that we're slouching directly into that future, of course. We're like the Vikings on Greenland, with Rupert Murdoch saying "don't eat those fish. I know they look abundant and tasty, but they're communist, atheist fish - you won't go to heaven if you eat those fish".
I think I can guess what the (hungry) Vikings response to Rupert would have been! They did actually eat fish there.
Agreed we need a new word, who really want's more of what we have to day - economic instability, all this arguing over whether PO wil happen or not, etc etc.
The goals should be set at a 50% reduction in FF use.
Once we are there, we can try for 50% of what remains.
Trying to shoot for the moon inevitably leads to these counter arguments that it can;t scale.
We already have quite a mix of oil and electricity sources - we just need to start/continue/accelerate changing that mix - instead of caving to the legacy industries that want to maintain it.
And given that said legacy industries seem to hold more influence in the US than anywhere else (except perhaps Australia), It's hard to see the US being the place that does it first. Which is too bad, because whoever does do it first, can export their knowledge to the world.
I'll have to think about what that word might be, but the right word/phrase would make a difference in this age of sound bites
I don't think this is the right approach. If you plan for 50%, you will make investments based on the use of the remaining 50%. Going further means throwing some of those investments out.
The way to go is to aim for 100%. For instance, try to completely de-carbonize the electrical grid. If your system of nuclear, wind and hydro still needs some gas and maybe a bit of coal to keep it humming, it's a lot easier to keep 15% of the old than to tear out and rebuild 30% of something you just installed.
I thought the idea was that the Vikings of Greenland died out because they refused to eat fish. Did I misunderstand Diamond?
The Ecotechnic Future?
That's a great phrase - unfortunately, it's already been applied by Greer to a different vision than I would paint. Greer's vision, as I understand it, is based on long-term energy scarcity, which I think is unrealistic.
Scanning the comments reveals widespread sentiment that evaluating a fully-scaled lead-acid system is a waste of time, a straw man, a gross exaggeration, a damaging message, etc. The final paragraphs of the post make it clear that I'm not saying we can't come up with adequate solutions: just that scaling up lead-acid will not do the job. If you already knew that, you deserve a pat on the back. It isn't common knowledge that we lack the lead for the job.
If I had evaluated and knocked down ideas for storing sufficient energy in giant springs or suspended rocks, I could understand the howls. But lead-acid is the storage medium of choice for most stand-alone renewable systems. People are very familiar with it, and it has some very nice (efficient) properties. The question is natural for any newbie to the subject: could we just use the same proven technology at a national level and call it done?
There is value in mapping out terra incognita. We need to know what "obvious" and familiar solutions are not worth wasting time pursuing. We also need to understand that the solutions won't come easily, so that we devote adequate attention to exploring the alternatives well before we need them yesterday. I love the degree to which the post stimulated thoughts and real analysis on what schemes might succeed. Keep fleshing out those ideas. It's only easy in the rear-view.
That's a great clarification, especially the clear and simple statement "I'm not saying we can't come up with adequate solutions: just that scaling up lead-acid will not do the job." If you revise your article slightly to include it then I'd be very happy with it.
I hope I'm not beating a dead horse when I clarify that a shortage of lead isn't the primary problem with LA. The primary problem is that the large capital cost of LA needs to be amortized over 300-1,00 deep discharge equivalents (perhaps 100 per year). That doesn't fit with a need for extra power on a seasonal basis: perhaps 1-2 times per year. Pumped storage is a more extreme example of high capital cost paired with high efficiency and low operating cost: used daily for 30 years it's extremely cost effective.
What's needed is something with very low capital costs, even if the operating costs are higher. That's why NG peakers and diesel generators are popular for this. In a non-FF world balancing solutions include supply diversity (including negatively correlated wind & solar; hydro; nuclear; geothermal, wave, tidal, etc); geographical dispersion; Demand Side Management; storage of H2, synthetic methane, ammonia, compressed air, etc; modest amounts of biomass (wood, etc).
So, LA or any electrochemical battery is really just the wrong kind of solution - it's like trying to use a hammer to drive a screw.
Nick, Tom, great comments.
Agreed that, obviously, we can;t solve the storage problem with lead acid - or any other kind of chemical battery, but then, we really don't need to.
If we frame the question as "how do we go to a 100% renewable grid?" then the storage problem has to be solved.
If we frame the question as "how do we get to 50%(or even 75%) renewable generation?", then the storage problem can be avoided, in large part, by the use of peaking power plants and DSM.
This is not to say we should not pursue storage options - we should, and we should implement those that are practical, and keep looking for ways to improve them. What concerns me is if we start to see lots of investment being diverted from renewable generation and DSM, to less than optimal storage, to address the intermittency of wind and solar, when the intermittency is not really a problem yet, and won't be for some time.
I would be quite happy to see a grid that consisted of renewables +100% NG backup, where the renewables provide 60+% of the total kWh. That would leave NG at not much greater fraction than what it is today (25%).
In fact, the goal really just needs to be to do enough renewables to get rid of coal (50%).
For now, NG backup is a far cheaper (large scale) solution to intermittency than exotic storage. Since NG backup is a mature, cheap and off the shelf technology, I think we should use that and get on with the job of building the renewables. Storage innovations will get developed, but there's no need to wait for them.
Thanks, Nick. As far as:
The original post already does say this in slightly different words:
"This post does not proclaim that there is no way to build adequate storage to accommodate a fully-renewable energy infrastructure."
...then talks about other potential solutions and re-iterates that lead-acid (referred to as the current go-to solution [for stand-alone renewable systems]) does not scale.
So I will put the pieces together and conclude that you're very happy with the article. (picture smile emoticon here).
Well, we're mighty close to agreement. I'd like to call it settled. The thing is...the article as it stands is significantly misleading.
It suggests that we have a really major storage problem, when we don't. It says that LA is the cheapest way to store electricity, when it's neither the cheapest way to store power for seasonal consumption nor the cheapest solution to seasonal intermittency. It suggests that we might be in danger of running out of time to implement solutions to dealing with renewable intermittency, when we really aren't.
The fact is that the biggest problem standing in the way of alternatives to fossil fuels isn't cost, or technical problems like storage. It's our collective lack of will to find ways to help (or otherwise tame) the obstructionist minority who would be hurt by them: car companies, auto workers, oil companies, petroleum engineers, coal companies, coal miners, etc., etc.
Engineer-Poet, Nick,
the biggest problem standing in the way of alternatives to fossil fuels isn't cost, or technical problems like storage.........
For wind and solar the biggest problem is the need for feed-in-tariffs or tax credits to be able to compete with gas-fired generation. The fact that wind is expanding at 30% per year and solar >50% per year indicates that this is not a major problem.
For nuclear in some countries its the obstructionist majority opposing nuclear, in other countries(US) is the investor risk in building nuclear requiring loan guarantees . For China is the capacity constrains in expanding nuclear as rapidly as rising demand for electricity.
Sure, in many countries obstructionist minorities can block an individual coal-fired generator, nuclear generator or wind farm, but to claim this is the biggest problem for nuclear especially in US is to ignore history.
The investor risk in the USA is mostly political risk. The government of Vermont is attempting to force the Vermont Yankee plant to shut down, despite the NRC's (much delayed) extension of its operating license.
The NRC has never in its history approved an all-new design for a nuclear plant. The risks of applying for such approval are too high for industry to attempt it. The route forward appears to be through one of the loopholes around the NRC's authority, such as the exemption given to the Department of Defense for projects of military importance. Flibe Energy has already announced that it's ready to take this route.
Engineer-Poet,
When elected governments choose to shut down nuclear power, we would expect that they are reflecting the wishes of a substantial part of the electorate. The fact that >100 nuclear power plants are still operating in the US indicates that in many states nuclear power has the support of a substantial part of the electorate.
Eventually the reactors built in the 1970's and 1980's will have to be replaced and another 100-300GW of new reactors built if nuclear is going to make a major contribution to further CO2 reductions. Clearly this is going to require a massive government financial commitment, beyond tax credits applied to renewable energy, even with a substantial carbon tax. I sincerely hope that this happens as soon as possible because of the long planning approval and build time for nuclear.
Meanwhile industry appears willing to continue to make major investments in solar and wind energy providing tax credits are renewed.
Both new renewable and nuclear projects are going to have local opposition at specific sites.
Unless the decision is subject to referendum, that's not a good assumption; pressure from activists and campaign contributors often outweighs the wishes of the voters. Even if it is, voters are often uninformed. For instance, an informed decision requires knowledge about the effect of a shutdown on carbon emissions and electric rates, both of which would rise substantially.
The nuclear industry seems to be going towards factory-built subsystems and even entire reactors. If this trend continues, both financing and construction schedules will be much easier to handle.
I'm looking forward to small fast-spectrum reactors, because we need some way to consume the actinides from the last 70 years of nuclear energy.
Imagine lead-acid batteries as big as a bedroom or a house. Vented with nitrogen? so if overcharged slightly, producing hydrogen gas, could be easily seperated, with no explosive oxygen present? Also have compressors/motors able to switch easily between AC grid and DC. Is anybody doing this?
Since discussion here is winding down, let me wrap up my thoughts on it.
While Tmurphy's calculation was for storage from lead-acid batteries, that's really not the point. The point is to demonstrate that, when we're dealing with the power supply of a major nation, *any* proposed solution has to deal with the sheer amount of material required to build it.
When dealing with national power, you must always ask, "Is there enough X in the world to do this?", whether X is lead, sodium, nickel, steel, aluminum, neodymium, man-hours or gigajoules. The answer may be "yes", but you've got to work it out.
And you can't just handwave "a diverse mixture of solutions" either. That may be turn out to be workable, but you still have to ask, "Is there enough W, X, Y, *and* Z in the world to do this?"
It comes down to the title of Tmurphy's blog: describing a technology isn't enough. You've got to Do The Math.
I'd put that differently: we can't fall into the "silver bullet" fallacy: "this approach can't handle 100% of the problem, therefore it's not useful". Instead, we have to ask instead just how large a role it can usefully play. For instance, a week of power is 2% of total annual power consumption - could biomass provide that much?
Further, estimates of how much resource are available are very often highly unreliable. Many resources are identified in enough detail to ensure that the company or industry can operate for another 20-30 years of current consumption, and no further. There may be 1000x as much available, but it simply hasn't been identified.
So, it's always helpful to quantify things. But let's use those numbers properly, and not push the numbers beyond their zone of reliability.
You're not putting it differently, you're claiming the opposite. You're claiming that hand waving on diversity can replace a viable plan.
Nobody has claimed you need one approach to go 100%. You just need to put together a combination that comes close, without hand waving.
You're not putting it differently, you're claiming the opposite.
Well, I am putting it fairly differently.
You're claiming that hand waving on diversity can replace a viable plan.
I think that claim belongs in a different argument.
Nobody has claimed you need one approach to go 100%.
I'd say that's exactly what the Original Post suggested.
You just need to put together a combination that comes close, without hand waving.
Well, actually, you don't even have to get that close. We have enough fossil fuel to last quite a long time. We really just need something that will work well enough: reduce emissions quickly and sharply while not raising overall costs significantly.
There are quite a few studies that show that renewables can claim a market share of up to about 30%, with no dramatic changes to our current grid. For better or worse it will take quite a while for us to reach that.
It would be nice to do a more ambitious study: it just hasn't been seen as needed by anyone in the industry. Heck, I'll do it if I have the time sometime soon.
You could always store power in rocks, like this. http://www.isentropic.co.uk/index.php?page=storage
Maybe we should also consider that if we choose to negawatt by riding bikes, manual labor etc., each human will need to consume 4X the calories of what is needed by the average desk job. Factor this concept into the model to figure how much more food will need to be produced to support all these people who will be using less carbon based energy and more "food" energy. How much quicker does our soil resource wear out?