Revisiting the Fake Fire Brigade Part 2: Biomass - A Panacea?
Posted by nate hagens on July 24, 2010 - 10:25am
Below the fold is the 3rd in a series of posts providing analysis (and follow up) on the difficulties of maintain our current energy paradigm with renewable energy (generally, 'the fake fire brigade'). The main authors are Hannes Kunz, President of Institute for Integrated Economic Research (IIER) and Stephen Balogh, a PhD student at SUNY-ESF and Senior Research Associate at IIER. IIER is a non-profit organization that integrates research from the financial/economic system, energy and natural resources, and human behavior with an objective of developing/initiating strategies that result in more benign trajectories after global growth ends. The authors wrote over a 20,000 word follow-up to the questions raised in the original posting and we've broken into 4 pieces for readability - the 2nd installment, with a focus on large scale biofuels, follows.
In this post, the authors show that even if we can double current extraction of biomass, this only represents approximately 6% of total U.S. primary energy consumption, and will likely never be enough to serve as a meaningful stabilizer in future energy systems.
The previous essays in this series were 'The Fake Fire Brigade - How We Cheat Ourselves about Our Energy Future' and Revisiting the Fake Fire Brigade Part 1 - General Issues.
Biomass - A Panacea?
In many resource discussions, biomass emerges as a solution that allows us to continue many activities currently powered by fossil fuels: First, to move cars, trucks, machinery and planes when oil runs out or becomes too expensive. Second, to provide flexibility in electric power generation, i.e. when other sources are stochastic and inflexible, biomass would provide the necessary gap-filling power. Third, to heat our homes, after natural gas production declines. There are many estimates of future uses for biomass, and many new technologies that are making their first baby-steps, quite a few of them promising and worth trying.
When we add up what biomass is projected to accomplish in the future, we quickly arrive at large numbers, which don’t take into account the fact that extracting energy from solar flows is not something that can be easily scaled up at our discretion. Below, we look at some key biomass technologies, and also spend some time attempting to put claims and reality into perspective.
Ultimately, there is no doubt we can produce some transportation biofuels, and little stands against burning wood or waste to generate heat and electricity, as many countries already do. However, what we need to bear in mind is that these solutions will replace only a fraction of today’s fossil fuels, and with far less flexibility. Below, we try to present an overview of biomass as an energy source and its significant limitations in the bigger picture.
To do so, we look at a model of the total theoretical biomass potential for energy in the United States, and then compare a number of potential uses. The U.S. is one of the world’s least population dense areas with moderate and inhabitable climate, which provides significant biomass potential on a per-capita basis. Europe, for example, has 3.6 times as many people per square kilometer when compared to the U.S., so any biomass calculation for the “old world” looks significantly worse, even when factoring in that an average Western European country only consumes about 50% of the primary energy per capita when compared to the U.S. (EIA). While we will look at the “best case” in the developed world today, Europe has its own history of biomass limits, which we now briefly explore.
The Middle Ages, a cautionary tale
At the end of the 13th century, Europe’s forest coverage, as historic data sources show and isotopic analyses of sediments confirm, was as low as approximately 10% of its total land mass. Today, 38% of the EU countries’ surface is covered with forests, up from 25% only 50 years ago. At the same time, today’s population in the EU-27 countries stands at close to 500 million, whereas at the peak in medieval times (between the years 1300 and 1350), Europe was inhabited by 70-100 million people.
Back then, the continent was reaching its biophysical limits for supporting humans in a biomass-based economy, and after the last marginal spots of land were put to use for farming, there was little that could provide any more sources for food, fire and construction wood for a thriving and quickly growing society. The discovery of fossil fuels and the New World were still things of the future. A few poor harvests led to famines in the 14th century all across Europe, and when an already undernourished and thus weakened population was exposed to the black plague, Europe’s population shrank by 35-50% (depending on sources) within less than a century. Things only improved once untapped resources in America were discovered, and new trade routes opened. So clearly, back then, there wasn’t enough sustainably growing biomass for a fraction of the people living today.
Crop yields a few centuries ago were equally only a fraction of today’s, because many things known and available to us weren’t. However, current yields – a result of ongoing research and innovation – are not just a product of science and better technology, but also come at the cost of additional energy inputs from (mostly fossil-fuel driven) fertilizers, minerals, pesticides, irrigation, equipment and other technology. Thus, while human labor requirements in agriculture have fallen, net output increases in terms of “energy content in food” are much smaller after subtracting the fuels and embodied energy in the equipment used in modern agriculture. Some authors even outright claim that when factoring all this in, agricultural outputs are energy negative, i.e. require more (non-solar) inputs than they generate in outputs (Steinhardt and Steinhardt, 1974)
How a plant cycle works
One of the key reasons why we were able to dramatically increase yields is the fact that we have been able to better understand how plants grow. By comprehending this process and managing it properly, we are able to emulate nature’s cycles while still extracting significant amounts of biomass for human purposes. We do so by adding fertilizer and nutrients, providing enough water, by eliminating competition through herbicides and pesticides, and by focusing on high yields through breeding, hybridization and genetic modification. To understand the scalability of biomass, understanding some of these basics helps, so we think.
“Primary production” is the term that describes the creation of new organic biomass from inorganic molecules, namely water and atmospheric (or aquatic) carbon dioxide. In green plants, this is accomplished primarily through photosynthesis, and requires the presence of certain macro- and micro-nutrients. We calculate net primary production by subtracting from gross primary production the energy lost to cellular respiration and maintenance metabolism. Net primary production (NPP), then, is the rate at which plants in an ecosystem transform diffuse solar energy into useful energy. Some of this energy is used by the plant to grow and reproduce; some energy is transferred to herbivores and omnivores in the ecosystem, when they eat plant biomass.
Terrestrial primary production varies based on many factors, chiefly insolation, temperature and precipitation. There are 16 identified chemical elements essential to plant growth. In addition to Carbon, Hydrogen and Oxygen available in air and water, macronutrients from the soil include Nitrogen, Phosphorus, Potassium, Calcium, Magnesium, and Sulfur. Other nutrients are required also, though in much smaller amounts. These micronutrients include Silicon, Manganese, Boron, Copper, Iron, Chlorine, Molybdenum, and Zinc.
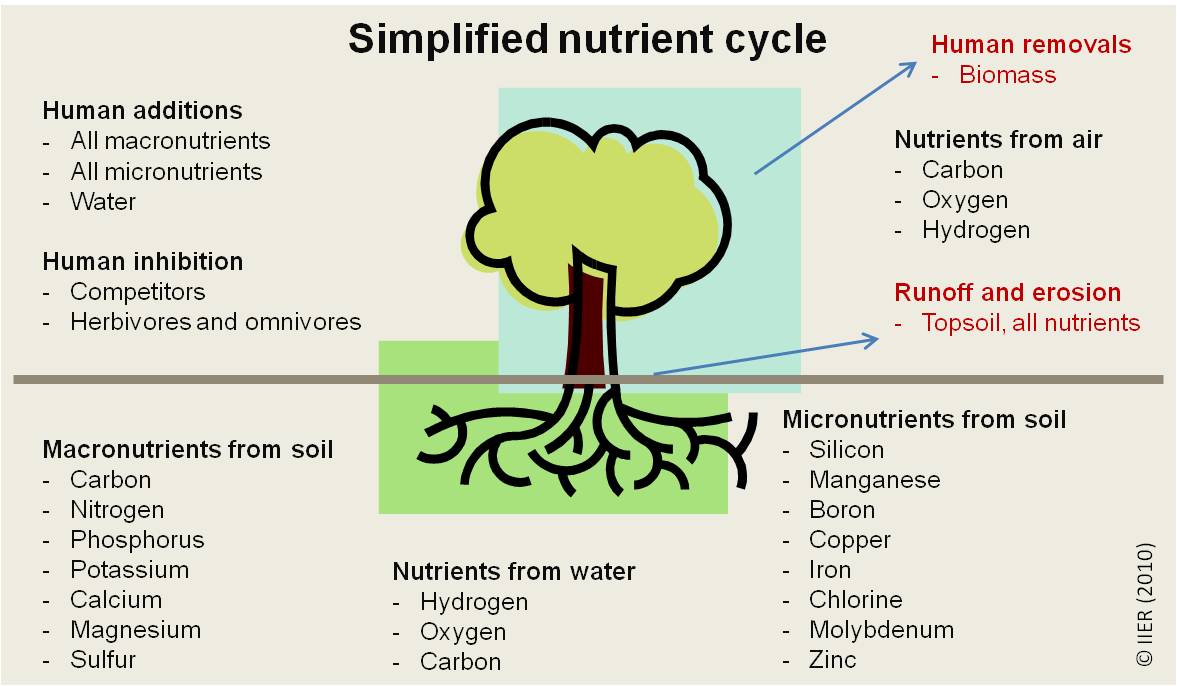
Figure 1: simplified nutrient cycle (IIER)
In adequate conditions, plants grow to maturity, produce offspring, and then die. Under most natural conditions, approximately 0.1% of incoming solar energy is converted into biomass by plants, and 10% of that is assimilated by higher level consumers. The remainder of the plant biomass is shed as litter or returned to the ground when the plant dies. Consumers that eat plants also return their nutrients to the ecosystem by excreting wastes and by their bodies decomposing after their death. Decomposers in the soil (bacteria, fungi, etc.) consume this waste from plants and consumers and make these nutrients available for uptake again (Figure 1).
While all of the nutrients listed above are required for plant growth and respiration, plant growth is a function of the least available nutrient. Liebig’s Law of the Minimum states that if one of the nutritive elements required for plant growth is deficient or lacking, growth will be poor even when all the other factors are abundant. Therefore, in an ecosystem with few losses to the environment outside its boundaries, nutrients are cycled efficiently through the system. In an ecosystem where humans extract large amounts of biomass, however, nutrients can be drained from the soil at a rate greater than natural replenishment. Over time, the quality of the soil degrades, and net primary production falls. Below is an example of a more detailed nutrient cycle for nitrogen.
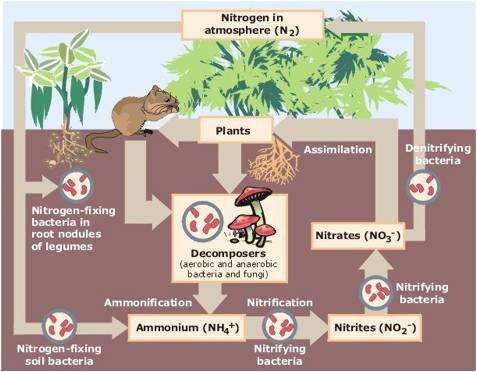
Figure 2 - Nitrogen Cycle (Source: Wikimedia Commons)
It is therefore important to note that over time, land under agricultural production degrades because of permanent extraction from growing crops, erosion, and other factors. A highly productive piece of farmland requires increasing fertilizer and mineral inputs to maintain high yields. Similarly, yields can be raised even on lower-quality land by increasing the amount of fertilizers and minerals used. However, this relationship is non-linear, and at high levels of fertilizer use the response becomes asymptotic. Since the farmlands degrade over time due to nutrient depletion and erosion, any disruption in inputs would reduce yields to a level below the original yields obtained on the site without inputs (Figure 3). In addition, the application of herbicides and pesticides can render the soil sterile and falling humus levels in the soil result in decreased soil moisture and contribute to soil erosion.
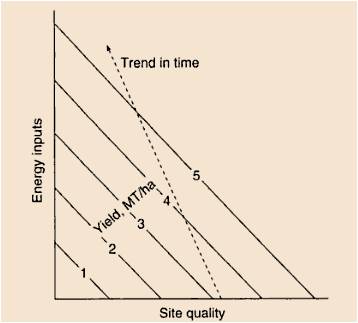
Figure 3 - Isopleths of Agricultural Yield. Source: Hall et al. (2000)
It is relevant to understand that each human extraction of biomass, if not compensated by the return of an equal amount of nutrients, weakens the future potential and capacity of the land. Ultimately, only ecosystems without human inputs and withdrawals arrive at relatively stable situations, where they operate at or close to a 100% regeneration rate.
So simply put, if we plan to extract significant amounts of biomass, this will ultimately deteriorate the soil and affect future fertility of the land, unless we can replenish it. There are multiple ways of replenishment, but all incur energy expensed elsewhere for those replenishments or slow the rate of extraction and make harvesting more complex (fallow periods, frequent switch of plants, multi-plant environments). A good source for more detail is provided here: UBC Soil Web.
United States Biomass Potential
The current extraction of biomass in the United States might provide some guidance regarding future potential. A current practical maximum of sunlight conversion into biomass is approximately 1W/m2 on average per annum. This translates to a maximum of 8.76 kWh (1 Watt x 365 days x 24 hours) per square meter and year. However, this is accomplished on prime land that is flat, low altitude, with sufficient insolation and water, with specific crops and with at least some fertilizers and technology use. Therefore, assuming even half (0.5 W/m2) as an average is probably generous for any larger country, as many locations don’t meet those ideal criteria due to elevation, weather, latitude, shades, absence of sufficient water, lower quality soils, etc. A look at many sources, including a recent FAO documentation supports this assessment.)
For the United States, this assumption of 0.5W translates to a total energy capture of 29’765 TWh (or 101.6 quadrillion BTU* (short: quads) for the continental U.S. for all cropland, forests and pasture areas. For comparison, total oil consumption in the United States amounted to 41.4 quads in 2008, and the country’s total energy use was 99.4 quads. In 2009, total energy consumption in the U.S. was down to 94.6 quads, mostly related to the recession. So ultimately, what is theoretically possible to be annually produced as biomass from U.S. land almost exactly equals today’s total energy consumption.
*The assumption of 0.5 W/m2 and a theoretical maximum production of 100 quads is significantly higher than available in other scientific publications.
Biomass uses
When looking at the above theoretical maximum of slightly above 100 quads, there are quite a number of uses for this biomass:
- Food for humans
- Nutrients and habitat for all wildlife (wild and domestic feed too)
- Soil regeneration (crop residuals are the basic ingredient)
- Other ecosystem services (carbon fixation, soil stabilization, etc.)
- Cellulose for construction material, paper production and other cellulosic material uses
In the future, others have proposed to use more biomass for the following purposes:
- Biofuels (Ethanol, Biodiesel)
- Cellulose for non-oil based plastic materials
- Electricity production (peak load and balancing of other renewable sources)
- Direct space heating
To understand the feasibility of this objective, and the scale at which this is possible, it is useful to look at aggregate numbers from an ecosystems perspective.
Current biomass extraction
In 2008, total agricultural biomass outputs (including animal feed and base for fuel production, excluding forests) equaled to approximately 6.6 quadrillion BTU (quads) . This calculation is based on the energy content in retrieved crops, where quantities can be found in the FAO’s agricultural database. Of those, approximately 20% (1.4 quads, mostly from corn) went into biofuels, producing fuel with an energy content of about 640 trillion BTU in the form of ethanol and biodiesel (using additional energy inputs from fossil fuels).
Additionally, in a meat-intensive society like the U.S., a significant portion of agricultural biomass (approximately 2.9 quads or 44%) is used as animal fodder. On top of that, animals feed from pasture land, which – at a relatively low conversion efficiency, but with very limited inputs – indirectly adds another 1.8 quads to the human food chain. Together, these 4.7 quads produce about 570 trillion BTU (0.57 quads) of annual animal products (meat, milk, eggs, cowhides, etc.). It is difficult to estimate the total quantities extracted from pasture land through grazing and haying, but as the above calculation translates into an 8:1 conversion ratio between fodder input and animal protein output, it seems reasonable. It is important to note that a significant part of the pasture land isn’t feasible for other uses, due to soil quality, insufficient water availability, topography, or size.
On top of that, about 0.1 quad goes towards other uses (tobacco, fabrics), so ultimately, from all arable land, about 2.2 quads are processed for human food directly, supplemented with another 0.6 quads from animals, and about 0.02 quads (20 trillion BTU) of biomass extracted from water bodies (fresh- and saltwater), which we will ignore due to its insignificance.
From U.S. forests, about 300 million dry metric tons of wood and byproducts with an energy content of approximately 5 quads were removed in 2008 (derived from FAO data). Of these, a significant portion of approximately 2 quads (EIA) went into energy production, mostly in the form of waste from saw mills, paper production and others already, which already today get mostly converted into electricity or process heat – in most cases providing the energy to process the wood and its by-products.
Table 1: Biomass extraction in the U.S.
So in summary, Americans extract approximately 13% of the gross biomass potential of the country, of which we ultimately use about 9%, after converting a significant portion to animal protein. Other sources say that this extraction is closer to 25% of total land potential on a global scale, so we might be too optimistic about the initial potential.
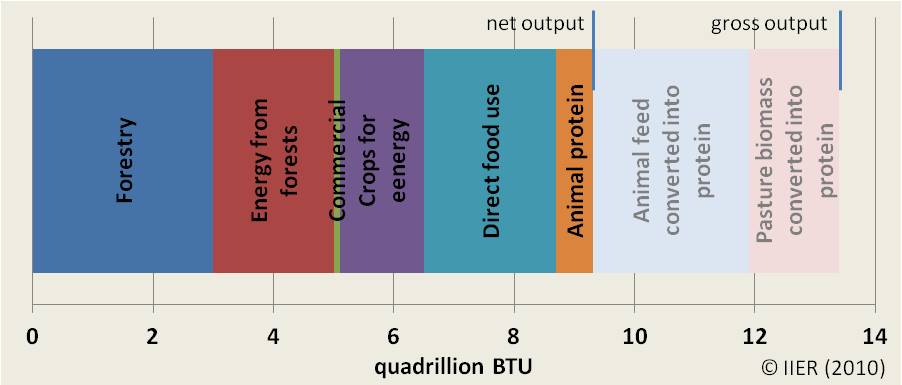
Figure 4: U.S. biomass outputs (2008)
Energy input requirements
Agriculture and forest use have been areas of high innovation during the past decades. If not for the “green revolution”, which included the optimized use of fertilizers, pesticides and hybrid or genetically modified “designer” crops, we wouldn’t be able to feed almost 7 billion humans on Planet Earth the way we do today.
Together, those human biomass extractions amount to between 9% (net) and 13% (gross) of the already generously calculated maximum of 100 quads of total annual biomass production in the U.S. All this is accomplished after a few thousand years of trial and error, with most of the gains in the past century from the application of inputs based on fossil fuels. Therefore, the above numbers do not include the significant energy inputs into extracting those 13.4 quads of gross output.
Should we have to provide external energy inputs mostly from biomass, it would further reduce net outputs. We have only limited knowledge about the exact ratio, but there are some indications. Pimentel (2007) calculates an EROI of 4:1 for corn (the crop itself, not the ethanol product). A 2004 estimate by the Congressional Research Service, estimated that 1.7 quads of inputs are required to produce the agricultural part of the biomass (6.6 quads gross, or 4.3 quads net after conversion to animal protein). The latter results in a similar net energy return ratio (EROI) of 3.9:1 (gross, generously ignoring pasture) or 2.5 (net, after conversion of fodder to animal protein).
Net energy extraction from biomass
All energy we use can be initially measured as primary energy. To do so, we count the raw energy content of each source contribution to our energy systems. The concept of primary energy allows us to compare what would otherwise be“apples and oranges”. For example, 1 kWh of directly usable electricity produced from hydropower is counted exactly the same as 3’413 BTUs (equivalent to 1 kWh) of theoretical heat value in coal, which has to be converted to something useful like process heat or electricity at a loss. To continue with this example, if we had to replace 1 kWh of hydro-powered electricity with coal, we would have to use 3 kWh of embodied energy in coal, converting it to electricity in a power plant with a typical 33% efficiency. So in order to serve the same purpose (in this case electricity), we would need three times as much coal to make the final amount of electricity available to society.
This brings us to one of the key problems of biomass, which doesn’t just apply for uses in agriculture: when it comes to primary energy content, we need more primary energy per unit of usable energy from biomass relative to equivalent fossil fuel based energy. The key reason for this is because biomass undergoes a lower conversion efficiency when turned into the energy forms we want to use (electricity, transportation fuels, etc), due to its low energy density, its state (solid, not fluid or gaseous) – both of which lower the effectiveness of converting the primary energy into usable fuels.
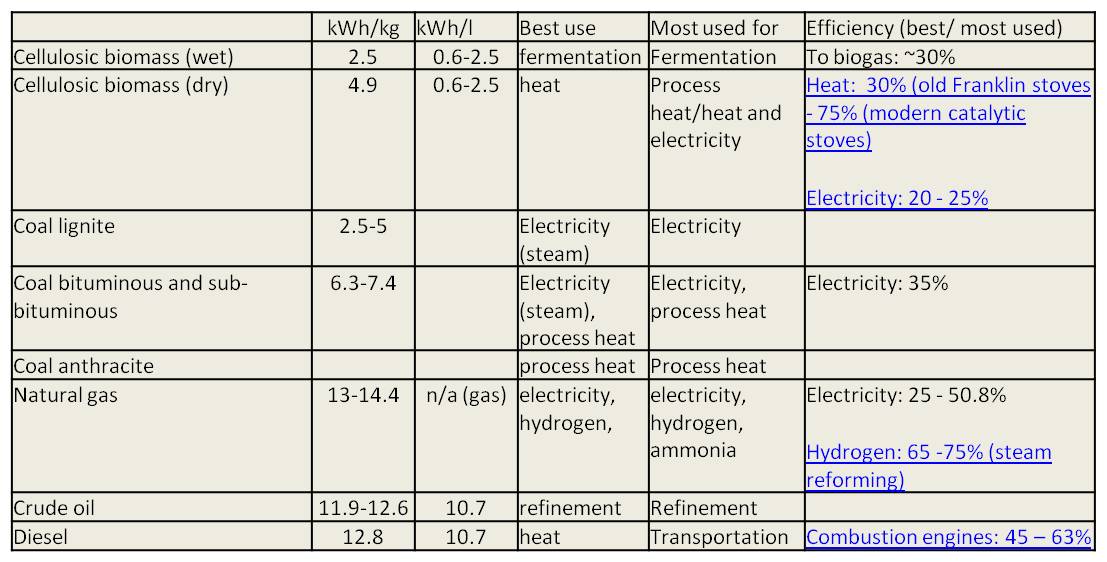
Table 2: Energy density and conversion efficiency of multiple materials
This translates to a different energy delivery profile (lower heat, less flexibility), and thus lower overall efficiency. The only place where biomass achieves a relatively good result is in heating, where relatively low temperatures are required. However, bringing biomass into individual buildings would require major refitting, which will reduce overall efficiency again.
To arrive at useful energy inputs, these original biomass inputs undergo a conversion, which creates significant losses. On average, many of those biomass-to-useful-energy conversion processes operate at efficiencies between 30 and 50% to arrive at the desired energy quality that is on par with comparable fossil fuels (coal, oil, natural gas). Below, we present a few approximate conversion efficiencies for multiple technologies and compare them with traditional pathways to produce the same final output. All examples are gross conversion efficiencies, not taking into account the energy used in the equipment, which mostly is more complex for biomass.
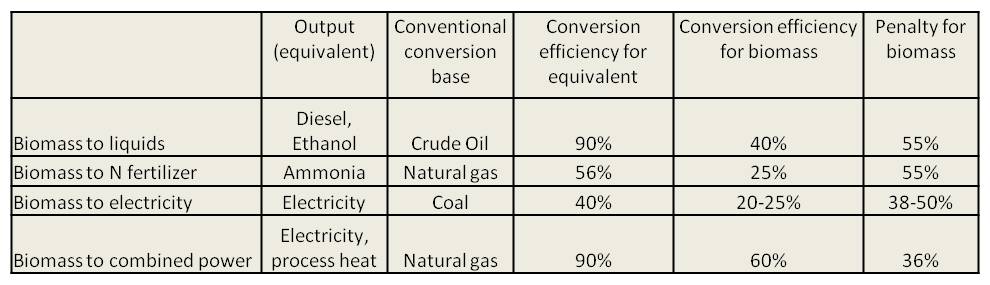
Table 3: Penalties for desired biomass solutions (IIER)
In order to understand possible consequences, we applied the lower efficiencies of biomass conversion to gross consumption of fossil fuels in the U.S. in 2008, based on end use. Approximately 143 quads of biomass are required to replace the 83.34 quads of primary energy consumption of fossil fuels – coal, natural gas and crude oil (Table 4). This handicap is not due to lack of appropriate technology, but simply related to the chemical properties of the materials involved, e.g. state (solid, fluid, gaseous) and energy density of the different sources. With significant shifts to better pathways, for example by heating more homes with wooden biomass directly, we might improve the situation slightly, but never fully, and always at the cost of significant technology investment.
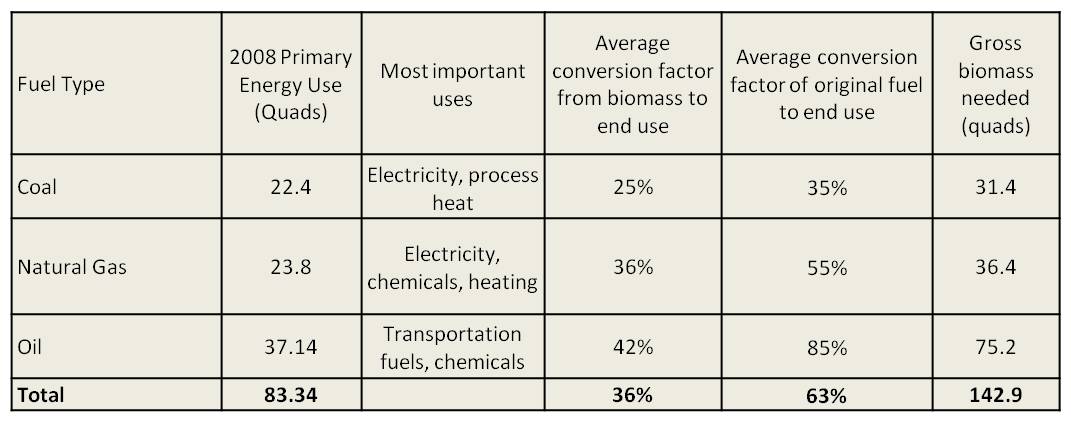
Table 4: Estimation of gross biomass needed to replace the useful energy from fossil fuels
Reality-check – how would self-sustained energy from biomass look?
If we take the more generous EROI ratio for biomass (3.9:1) and assume that the ratio remains unchanged when scaling to higher levels of biomass extraction, we can estimate how much biomass would be required to produce the same (or more) outputs from biomass in a self-sustained system where farms produce their own energy. In order to use biomass to provide the power to grow and harvest additional biomass, we also need to convert it into a usable form, e.g. to run a tractor, we need Biodiesel or Ethanol, to produce fertilizer we would require methane and or hydrogen, to mine and process nutrients, we would need fuels and electricity, and so on.
Thus, when looking at the 6.6 quads of total biomass from crops, an additional 1.7 quads of energy inputs would – at a 50% conversion rate – actually require 3.4 quads of agricultural biomass as primary input. So in that gross input model, for each net unit of energy extraction, input worth 0.51 units would be required.

Table 5 - gross energy requirements for agricultural output when energy is self-provided
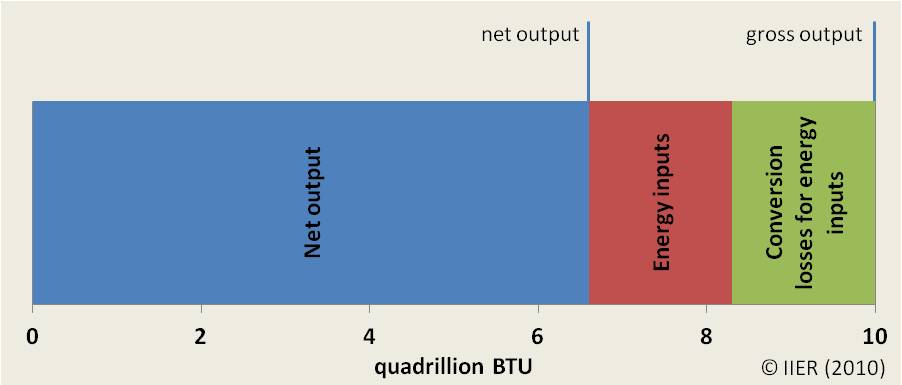
Figure 5: U.S. cropland biomass outputs and energy input requirements
It is probably fair to assume that forest related extraction is slightly less energy-intensive, maybe operating at 5:1 for the gross energy retrieved. Inputs to forestry include: management expenditures, planting new trees, harvesting, and transporting removed biomass. In this case, assuming a 40% efficiency of converting cellulosic biomass into useful input energy (which is very ambitious), we would need 0.5 energy units for each final unit of energy extracted (0.2 fossil fuel inputs for each unit of final wood biomass would require 0.5. raw units of biomass before conversion to useful energy).
These examples show that a re-introduction of a biomass-based society will likely use a significant amount of the biomass itself in order to steadily reproduce the same quantities.
Future extraction potential
One indication of how much biomass could be extracted comes from an otherwise very optimistic renewable energy study conducted in 2000 by Pimentel et.al. where the authors assume that total biomass extraction for energy could be grown to 5 quadrillion BTU in 2050, about 50% above today’s. However, other scientists and energy planners believe that today’s achievement of diverting about 10% of the annually generated biomass to human use can be easily doubled, tripled or quadrupled within a few decades. One of the most ambitious estimates was published in a combined study by the Department of Energy and the U.S. Department of Agriculture in 2005. This study assumes that the U.S. would be able to sustainably produce gross 1,366 million tons of dry biomass per annum available for energy purposes by 2030. This quantity would amount to 23.5 quadrillion BTU, or ¼ of current U.S. energy needs, about 23% of the theoretical maximum biomass net production. To our knowledge, this is the most ambitious estimate available; most others suggest various fractions of it. When adding current uses for food and industry, total extraction would amount to almost 37 quads, or 35% of total maximum potential. This means that we are to triple our biomass extraction from nature within a few decades.
Below, using these estimates, we build what we consider an optimistic model for the United States where biomass is produced with a generous input to output conversion ratio of 5:1 for biomass production (not including solar radiation), assuming future technology improvements from today’s levels. Then, the part of outputs going back into the production of biomass needs to be refined and converted to its final use (such as methane for fertilizer production, fuels for machinery, feedstock for electricity), which we assume will happen at an average conversion rate of 50%.
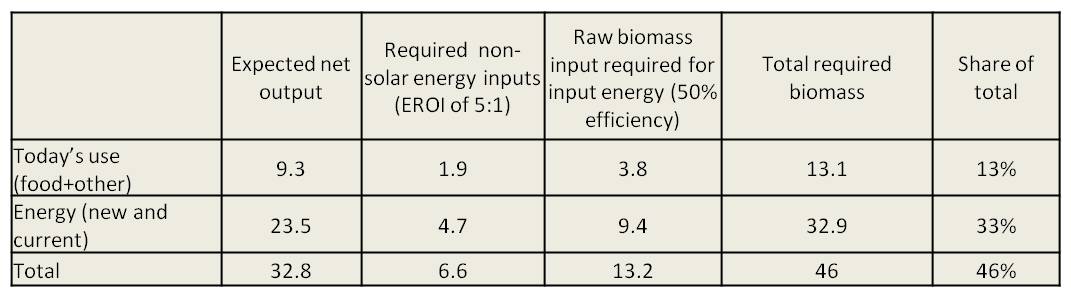
The above calculation shows that in order to net 32.8 quads worth of food and energy within those two decades we would have to extract almost 50% of the total annual U.S. biomass production for human use, compared to approximately 10% today. This is not something we consider feasible.
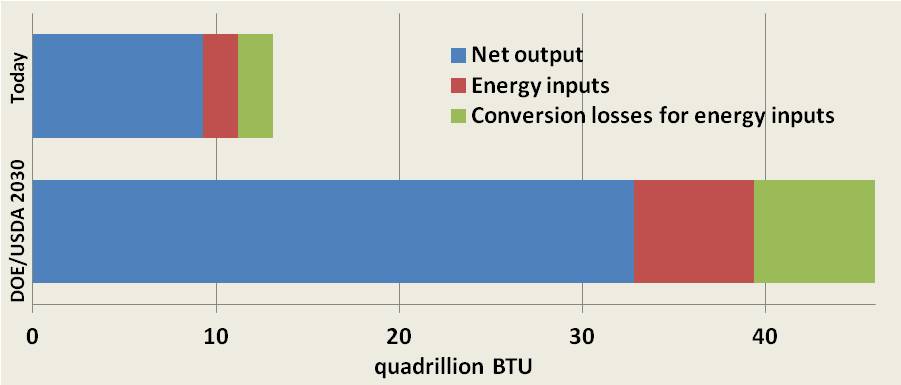
Figure 6: Energy output from biomass in 2030 DOE/USDA scenario
We could go into further detail regarding those projections, for example analyzing the assumption made in the above EIA/USDA paper that we can safely remove about 2.6% of all biomass from forests every year without adversely impacting the ecosystem (current removal rates are at around 1.5% in the U.S.). Knowing that even the fastest growing pine plantations have a cycle time of 30-35 years, and other trees around twice that much, makes this objective of extracting an average forest’s biomass every 38 years ambitious. These projections also ignore events like forest fires, pests, droughts, and other things leading to bad crop years.
All in all, it seems highly unlikely that we will be able to triple our output and divert almost 50% of total annual potential U.S. biomass production towards humans. Particularly since we know that this system is built on a cycle, which in the long run expects almost the same input as it produces.
One potential elephant in the biomass room – Phosphorus
Phosphorus is one of the key macronutrients required for plant growth. Unlike other limiting nutrients, phosphorus may be the most difficult to replace. It cannot, irrespective of crop, be fixated locally, and is relatively rare. To maintain or increase yields, we must replenish the soil with phosphates whenever we extract biomass. Phosphates are required by almost all species – not just plants – to build DNA, and if it’s absent, yields shrink relatively quickly.
Unfortunately, of all the ingredients needed for plant growth, phosphate rock is the one with the smallest amount of known reserves globally, which are geographically concentrated to just a few places on earth. Currently, phosphorus, which is mined in the form of phosphate-bearing rock, has known reserves of about 16 billion metric tons worldwide (USGS) , which represents about 100 years of current use. However, already now, the “half-empty glass” problem becomes increasingly visible, as phosphorus content of mined phosphate rock is decreasing rapidly, and prices are going up accordingly. Some people are talking about “peak phosphorus” within less than 30 years, but again, this is a moving target, depending on exploration and effort. But what is clear is that phosphorus, once it becomes scarce, will become more, and potentially very expensive to extract. 2008 provided a brief glimpse of what a world of limited phosphorus could look like. Shortly before the economic crisis hit, phosphate rock prices rose from $30-40 to 400$/metric ton within less than one year. With the crisis, they came back down to about 70-80$, still twice as much as before the increase .
If we plan on extracting two or three times as much biomass from our soils, one would expect that we will have to increase phosphorus use. So in order to produce those 32.8 quadrillion BTU, we likely would need three times as much phosphorus as today, which will pull the end of easily accessible phosphorus closer, maybe as close as 10 or 20 years.
This leads us to the conclusion that our current agricultural systems aren’t as “renewable” as they may seem.
True potential and technologies
After covering the entire system’s potential, we see only limited opportunity for any significant biomass output growth. However, current plants used for ethanol production aren’t ideally optimized for inputs (a vast majority in the U.S. is corn), which has led to the discussion of replacing them with other plants with better growth profiles. Among them are a number of perennial grasses, like Miscanthus, or switchgrass, which both produce cellulosic biomass. Sugar cane, the key energy crop used in Brazil, which produces high shares of carbohydrates, unfortunately doesn’t grow in most U.S. regions due to its need for high moisture levels and temperatures. Importantly, these grasses (as is sugarcane) are capable of fixating nitrogen directly (due to bacteria in their own rhizome), which greatly reduces nitrogen fertilizer needs. In addition, they produce – under ideal circumstances – biomass output close to the aforementioned 1 Watts per square meter. The downsides are that the produced biomass is cellulosic, which requires a much more difficult-to-manage fermentation (or gasification) process to turn it into a useful combustion fuel like ethanol or biodiesel. As of yet, no meaningful and scalable processes have emerged with decent conversion efficiency. What works is to burn cellulose for the generation of heat and electricity, which is the predominant use of wood residuals, but even there, conversion efficiency is low, particularly for electricity.
Within biomass for energy, we basically look at three types, with different characteristics: lipids (oils), carbohydrates (sugars, starch, etc.) and cellulosic materials (wood). They are different in their chemical structure, and come from different parts of plants. As humans can’t digest cellulosic biomass (unlike cattle), our food mostly consists of lipids, carbohydrates and proteins. Humans utilize grazing animals to convert cellulose to usable biomass in the form of milk and meat. In order to understand the potential of biomass further, it is worthwhile looking at the potential of each plant category. We won’t go too much into detail, but do provide an overview. In the table below we show the characteristics of each group and their usefulness for energy crops.
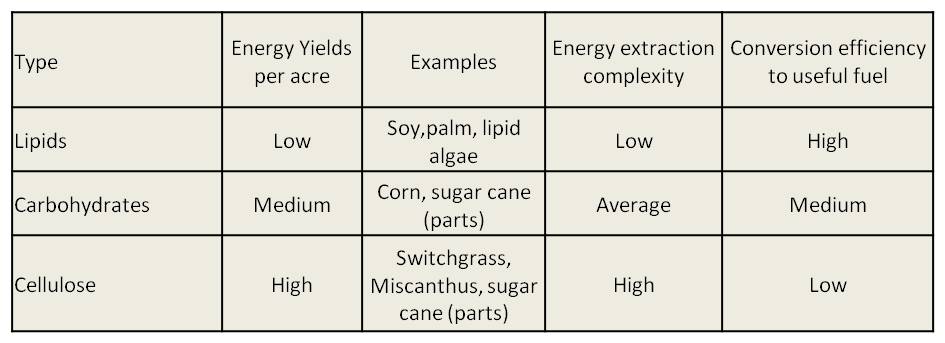
The fact that cellulose is so hard to “digest” into something that is useful as a liquid fuel, led to a focus on biofuels that either come from lipids (Biodiesel) or carbs (Ethanol). The generation of cellulosic ethanol proves to be much more complex and energy-intensive when compared to fermenting ethanol from carbohydrates, which is the key route taken today in the U.S (mostly from corn) and Brazil (mostly from sugarcane, where the bagasse also provides the process heat from its cellulosic material).
Algal biomass?
There are several reasons that algae are seen as a promising future source of renewable energy, especially as a source of biofuels. First, algae grow at a high conversion efficiency (theoretically up to 4 W/m2), with a large share (up to 60% of total mass) of easy-to-process lipids and proteins. Unlike corn ethanol, sugar cane, and any energy crop that requires higher quality arable land to grow, algae can minimize or avoid competition with food crops. Many plans involve using desert locations to maximize sunlight use. Also, algae can use waste water and saline water inputs and can be fed from stationary sources of CO2, turning sources of waste (such as carbon-or natural gas-fueled power plants) into energy and recycling carbon emissions (DOE 2010). The problem with algal fuels is that their production has never moved beyond small scale trials (relative to consumption), so scalability is still an unchecked issue.
One might think that this is normal with a brand-new technology, however, it seems important to note that – like most other things discussed as alternatives today – the concept of turning algae into fuel is not a new one. Research began as far back as the 1950s, but did not gather true momentum until the first oil shock of the 1970s, when the U.S. government funded the largest research program related to algal biomass, which ran from 1978 to 1996 (the Aquatic Species Program). The results were disappointing, with open pond systems often failing due to the starting strain of microalgae being overgrown by other, less energy-producing species that contaminated the pond. Open ponds, particularly in places with high insolation, also require massive amounts of water. A hectare sized cultivation system can require 2 million liters of water (530,000 gallons) to fill and around 50,000 liters a day to replace evaporative losses (DOE 2010). Since most of the evaporation is pure water (H2O) only, the pool cannot be endlessly replenished with wastewater, because this would slowly increase concentration of toxins and other materials in the pond. Providing freshwater, on the other hand, is energy-intensive, particularly in the locations where insolation is high. These are some of the reasons why things haven’t worked out so far. None of the companies suggesting open-pond systems seem to have answers to this problem, and to us, it looks like one that doesn’t yet have a solution.
Closed-system approaches, where algae are grown in a sealed tank that is supplied with carbon dioxide and nutrients in a controlled fashion have produced promising results in the lab, but have equally faced difficulties scaling up to a meaningful size. The key problems continue to be stability and long-term returns, plus the high cost of creating a clean and closed environment, which can be compared to gigantic petri-dishes with a lid. The key issue with closed-system approaches is that the only input we don’t have to provide from the outside the system is the sunlight; everything else needs to be produced, supplied and managed directly. Producers must use inexpensive sources of nutrient supplies, especially N, P, and K, to keep biofuels from algae cost-competitive. Higher quality sources may compete directly with inputs into our agricultural system. Closed systems require constant monitoring and precise application of nutrients as well. Too little of an additive and the crop will fail to produce; too much may render the growing medium toxic – or lead to issues with waste water disposal. Recycling the growth medium and wastewater from algal plants is possible, but expensive and energy intensive. Usually, this doesn’t bode well for EROIs, despite potentially higher yields and lower environmental impact.
These are the two approaches tried for algal photosynthesis. As with any yet unproven technology, promises are high, but equally are risks, and most seem insurmountable to us, or only at the cost of large energy inputs to maintain stability in a scaled-up facility. One other use of algae is not so much for the creation of new biomass (autotrophic), but instead for a heterotrophic way where algae do not need sunlight but instead are fed carbohydrates (from plants) and convert them into lipids, thus creating an almost fuel-type input. While this system might actually increase the efficiency conversion of – say – corn to a biodiesel product, it does not change the primary requirement for growing more biomass on land. It is important not to confuse the two approaches, as this one doesn’t overcome the limits of growing biomass on land. And again, it will suffer from similar problems with scalability. Links to algal biofuel information:
National Algal Biofuels Technology Roadmap (DOE 2010)
Dimitrov (2007) – A Case Study
What about waste?
IIER’s European offices are in Switzerland, the country that has abandoned most landfills, and instead burns more than 95% of its non-recyclable flammable waste (including outputs from wastewater treatment) to generate energy or process heat. Overall process efficiency for combined heat and electricity generation (for which about 70% of total waste is used) is approximately 42% (in German), which includes energy for internal use of the waste power plants.
Using Switzerland as an example, from the original energy content, 35% can be extracted as energy for external use (24% as heat, 11% as electricity), about 6% are used for the operation of processing facilities. Technology feasibility studies show that net electricity production might be slightly increased to probably 15% of total energy content. What is important to know is that those waste plants work best and most efficient when producing base load at a high load factor of close to 90-100%. They are – like other entities burning biomass – not really capable of modulating easily below a 60% level, as total fuel is so heterogeneous and needs a constant level of heat to burn properly.
When applying today’s Swiss technology to the U.S., converting 232 million metric tons of waste to energy at the above rates, this would deliver approximately 119 TWh (0.4 quads) of base load electricity (at 15% efficiency), which is nothing more than 3% of today’s electricity consumption, and 0.65 quads of usable heat energy (at 25% efficiency).
So what is the true biomass potential for energy?
Based on all the above assumptions, we have tried to establish our own best case for future potential biomass extraction. We assume a significant realignment of land for growing energy crops (or algae), steady doubling of outputs from some of the switches, and a much more efficient energy conversion process compared to today’s best-in-class technologies. Our model operates with the following assumptions, which even require hard decisions by people, e.g. the reduction in consumption of animal protein: - Reassignment of all corn land used for biofuels (mostly corn) to a better biomass producing crop, doubling the output per acre
- Reassignment of 1/3 of animal fodder producing cropland to a good biomass producing crop with a doubled biomass output per acre
- Reassignment of 10% of the best pasture land yielding biomass similar to today’s corn output from crop land (or alternatively assigning land for algal biofuel)
- Raise meaningful forest energy extraction by 0.5 quadrillion BTU
- Conversion to usable fuels comparable to today’s fossil fuels at an average 50% efficiency
- Biomass production with a total EROI of 6:1 (based on net final fuel inputs)
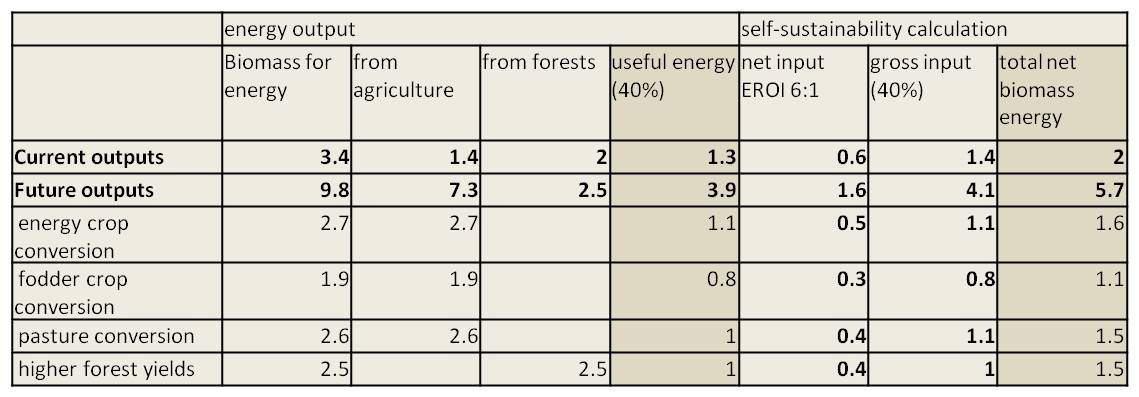
With these rather radical steps, we would expect today’s biomass gross energy extraction could be increased from 3.4 quads to 9.8 quads. However, as this energy is in “low quality” form, converting it to useful inputs (like combustion fuels or methane) will likely yield only 40% of that gross amount, e.g. 3.9 quads in this scenario.
The above 9.8 quads (gross) or 3.9 quads (net) are what we can expect as biomass output per annum. This does not take into account the required energy and material inputs into production. In a future scenario where farms are self-sustained, these inputs would have to come from biomass once again, which would reduce net available energy even further. The 9.8 or 3.9 quads are only available as long as we mostly have fossil fuels as inputs into agricultural production.
Flexibility of biomass in electricity generation
Biomass (and waste) have been repeatedly quoted as a source of flexible electricity generation. As already described in the section about waste, biomass is – when burned directly – mostly capable of providing base load energy, as the flexibility of burning wood at relatively low temperatures is very limited. Also, a storage problem will make it unrealistic to keep biomass as a flexible source for (long term) bridging of gaps in other renewable production. This is due to the low energy density of cellulosic biomass when compared to its fossil competitors.
One feasible alternative is the conversion of biomass to methane. However, this process that uses two steps (fermentation first, then burning) shows low conversion efficiency, turning an already small amount of energy in biomass into an even smaller amount of electricity.
Conclusion
In this part of our series of posts, we have revisited biomass potential as a contributor to future human energy systems. Until now, we only use biomass as a by-product of other uses and/or in subsidized ways (both by money and fossil fuels) at very small scales compared with total energy consumption. Increasing our biomass production is going to be problematic in many ways, and not just in the conversion from biomass to fuel, as with cellulose, but more so with the generation of enough biomass in the beginning, where we think it farfetched to produce much more than we do today. Overall, we come to the following conclusions:
- Theoretical biomass production potential is high, but can only partly be (further) diverted towards human uses and is limited by inputs, diminishing returns on marginal land, competition with food production
- Even if a meaningful production of biomass can be achieved, this is at the expense of high inputs (low net energy extraction), and the resulting output requires refinement to usable fuels in processes with relatively low efficiencies
- In a world with expensive and scarce resources, farmers may need to return to providing a portion of their own energy to grow crops. This may result in improved sustainability of farming, but limits the alternative uses for this biomass-derived energy.
So even if we can double current extraction of biomass, this only represents approximately 6% of total U.S. primary energy consumption, and will never be enough to serve as a meaningful stabilizer in future energy systems.
Series continues in The Fake Fire Brigade Revisited #3 - The Biggest Part of Business As Usual - Electricity>
Outside of Greek mythology there have never been nor will there ever be any panaceas.
Personally, I despise that word, when used in the context of rational discourse about any of the myriad dilemmas we face today...
Have you got anything to say on the post!?
As for a rational discourse, this is one part of a series in discourse around our complex FF based system and how serial substitution by renwables seems to be based on flawed rational.
And yes, in a wider context, renewables by governments and concerned bodies, they do seem to think of them as panacea making up for signicant FF shortfalls.
www.eia.doe.gov/conference/2010/?featureclicked=1&
www.zcb2030.org/
www.decc.gov.uk/
I just did! Sorry you didn't like what I said or didn't understand my point.
Framing anything as a "panacea" is pointless, if you start from the premise that there are *NO!* panaceas.
The closest thing to a panacea that I could see, would be finding a way to reduce economic and human population growth. I guess one might argue that reducing human biomass is relevant to the discussion...I'm all for that!
The strange thing about this thread is that I've read both your and oldfarmermac's post, which I find generally articulate and thoughtful.
And I agree, human population is the problem, especially in the more developed world. If there is a panacea, at the risk of falling into another pit of semantics =:-\ it would be a cultural one.
I just feel that the authors should be given a bit more courtesy for their time and efforts.
Sunnata, I wasn't trying to be discourteous at all. When the title of a post is: Biomass - A Panacea?
and my personal position is that there is no such thing as a Panacea I think it important to make that point of view known.
I was neither attacking the authors nor even claiming that the post did not have merit. Very simply put I feel strongly that words and how we use them are important. Which is why I posted both the current definition of the word and its historical roots in Greek mythology.
If I came across as being discourteous to the authors by clearly stating a personal view then I apologize. Though I'm not quite sure in this case how it was that I came to offend.
FMaygar's comments are perfectly on topic;it's just that he left out the intermediate explainations of why these proposed levels of biomass energy production cannot be achieved or sustained-reasons perfectly obvious to biologists or similarly technically educated folks such as yours truly.
He just forgot that sarcasm doesn't often register with the typical audience here -technically savvy and well educated but not always in biology related fields such as ag.
We can be rather literal minded when it comes to written communication.
I must say that I agree whole heartedly with his sarcastic remarks in respect to maintaining bau on biofuels.
We either ramp up solar, wind,and nuclear, or it is curtains for life as we know it in the rich countries..
Let up pray(figuratively, FMaygar!) that progress in these fieldss is fast and furious;else all the little girls that make life so delightful for thier old uncles are going to be stooped, half starved old hags when they are thirty-if they live that long.
Of course I'm slow on the keyboard and you beat me back with a response!
LOL!
Sunnata;
So far, all three parts that we've seen have carried the same problem in framing this question, and you repeat it in your post.
"..how serial substitution by renwables seems to be based on flawed rational."
Each time, it is couched in the assumption that these are supposed to be Silver Bullets. If the problem is Cornucopians and BAU, then say so, and make the Topic Title reflect this imbalance.
I had thought we were going to see an article on Electricity Generation next up.. but we get 'BioMass', which is of course one of the 'Renewables' that's fraught with limitations and problems, environmental footprint, water supply, soil quality, countless ongoing FF inputs, Pesticides, Fertilizers, Trucks .. but today, This get's to carry the banner of "The World of Renewables", which gets roundly debunked as NO SILVER BULLET once again.
Strawman Argument.
From the previous post:
"Our next follow-up post will deal with the potential of biomass as a source for future energy systems."
(Electricity is next up - please be civil and reasonable in the meantime. People here shouted 'straw man' at the first essay which is why I asked Hannes et al to write a follow up analysis, which they have done, in short order and in length - I am increasingly embarrassed at the treatment that guest posters get in this forum)
Thanks for letting us know what to expect. I wanted to shout 'straw man' again at this article since I know few people who expect future bio-mass to cover a much larger fraction of current global energy usage than it does at the moment. (See David MacKay, 'Sustainable Energy — without the hot air', available online) In defense of others who shouted 'straw man' earlier, one really should get to the heart of the matter relatively quickly. The question of whether human society can maintain current standards of living and energy usage beyond fossil fuels is not about the generalities addressed in part 1 and or limits on biomass addressed in part 2. The renewable energy sources with large growth potential are solar and wind, with a few other smaller ones that will probably be significant, so a long discussion claiming to explain 'difficulties of maintain[ing] our current energy paradigm with renewable energy' really should get to the main issue. The question is largely whether the energy and materials available are sufficient to build the solar, wind and other energy collectors (The answer seems to be barely but yes as long as you mean current global energy usage and not current US per capita energy usage for everyone on the planet), whether we will start building the infrastructure in time (Here the answer seems to be no), and whether human society will be able to remain stable through the transition (here the answer seems to be that anyone who thinks they can predict how humans will respond to the dramatic changes ahead is overestimating their foresight).
This was a comprehensive blog article on biomass potential, shortcomings, and risk. Obviously, Hannes has done his homework to support his thesis and conclusions.
On the subject of algal fuels, I would note that his shortcoming of evaporation in the deserts is not an issue in the humid, sunny southeast, and his mention of the need for N-P-K inputs misses the obvious human sewage input available. Of course, algal fuels still have many challenges to surmount to be considered a truly viable alternative fuel, so the primary thrust of the article rings true for what we can do today and in the near future.
I think you missed this line:
This comment is not cryptic at all. What jumped out of the article at me is the phrase "this only represents approximately 6% of total U.S. primary energy consumption." Of course, 6% of total U.S. energy consumption is absolutely huge. Several "mere" solutions like this and the problem would be close to solving.
- The study as a whole straddles several cultural 'niches'. It's not (the study so far) is not a polemic which makes it hard to figure out which 'side' the authors are on. I personally don't see this as a problem, the authors have a lot of valid points and can provide background materials so that interested readers are able to come to their own conclusions.
- It's impossible to leave out BAU since it dominates the political, cultural, social and economic establishment which dominates everything else. This establishment is both powerful and insecure, not a good combo.
- The authors fail to emphasise the primary stumbling block which is the current complete commitment to industrial agriculture. Whether a percentage of output can be diverted to machine fuel subsititutes (all food is fuel) is not really as important as whether there is enough 'slack' in the agriculture system as a whole to allow an increasing percentage to be shifted away from FF dependent production to versions less dependent.
I would say empirically that the answer is absolutely not because of all the prime productive land and water tied up in cash, monocrop fiber and starch production which cannot be easily shifted to the necessary multi- crop, unconventional (organic) regimes. Money losses to conventional producters could not be borne by the system as a whole. (Systemic risk similar to subprime mortgage failures jeopardizing the entire mortgage business).
- The conventional ag system is inherently fragile at a point where its complexity turns on itself. SOME input failures (such as too much rain, too little or rusts/fungus) can be absorbed but the 'system' will fail completely if the input failures reach a certain - unknowable - level. Conventional agriculture is a hyper- complex 'system' that is vulnerable to a relatively minor breakdown that resonates throughout and wrecks the whole.
- The American urban- suburban centers are surrounded by 'farms' which do not produce anything that humans can eat. They produce instead cotton or tobacco or feeder corn, or the ag product is CAFO livestock. The real product is subsidy dollars. This is a gamble, can you eat your money? What if the grocery stores run out?
This compares to agriculture during the pre- war period where all farms were subsistence first - garden vegetables, mixed livestock, starches and other grains PLUS the important cash crop. These farms were next to cities so that people could be fed in the case of a breakdown (such as the Dust Bowl drought). Los Angeles was adjacent to the Central Valley and its produce, vegetable and nuts. LA itself produced citrus. Cattle were raised in the valley. Miami/South Florida produced vegetables, citrus, exotic fruits and meat, dairy, sugar and sea food. New Orleans/South La produced vegetables and seafood, New York area produced vegetables, orchard and dairy products and meat/seafood. Chicago was the world's butcher (for better or worse) and a central processor of the nations' grain production. America has never had a problem producing enough varied food for itself ... until now.
Bere are my questions (let the cars find their own food!):
- Much of our edible food comes from Mexico and Chile, what happens with a diesel or bunker fuel shortage? Does this study suggest leaving these long supply chains intact?
- How do we transition to a multi- crop regime - when all the land, water and production inputs are committed to conventional monocrops? Who goes broke first? Who HAS to go broke first?
- How do we give hands- on organic farming experience as well as actual good land to farm on when all that good land is in the hands of corporations, subsidy- farmers or speculators? Farming is an art, where are the teachers, where is the material (land)?
BTW, it looks like NyMex crude is making another run @ $80/barrel. Look for another sickening slide in the stock markets ...
As it should be. We are interested in the truth, not fighting a propaganda war.
And what is says shouldn't be controversial. There is some exploitable resource, but it is way to small to support BAU on its own. The interesting thing to me is the tradeoffs:
(1) source of liquid fuels.
(2) biomass for load following.
(3) Biomass for chemical feedstocks.
(4) Go easy on nature, limit the biomass to preerve/heal the natural world.
And even if we only picked one of them -except for #3 the volume isn't large enough to come close to satisfying either 1 or 2. So future society will have to determine its priorities.
So lets talk about converting home heating to biomass (wood pellets and similar pellets--most people being too lazy to manually fill a wood stove with logs).
Sound public policy?
You mean converting biomass (wood into pellets) for home heating?
Without heating oil and natural gas, most areas of the USA would be clear cut within a few years
I remember my father stoking the coal furnace and hauling out coal ash. Nate, do you think we might go back to heating houses with coal over the next twenty years? Or are we likely to move in the direction of electric heat--with much of the additional electricity generated by coal?
Coal hauling is restricted by rail capacity at present, but rail capacity can be increased over time. I don't like what it means for CO2 in the atmosphere, but in the U.S. I can see King Coal making a big comeback, whereas biomass will always be a niche market.
I think its likely - as it will be lesser (and cheaper) of several evils -deforestation, freezing or pollution.
I agree with you Nate, here in Pennsylvania with lots of bootleg holes in the anthracite seams, and small mining companies, and family companies, coal is still used for heat, and it is growing. That same area also has mountains covered with trees, so firewood is used a bit more locally per farmermac. I prefer wood for various reasons but maybe the biggest one is that limbs and trees fall down on my 70 acre farm.
Hi schoff, and anybody else with acreage burning wood:
We used to burn all the dead wood on our place, until I went away and learned some woodland ecology and some basic forestry.
So long as you have adequate land available, it is best not to use natural deadfalls for firewood if you are interested in providing good wildlife habitat.A good many species of birds depend on dead trees for thier living, and bats, squirrels, racoons, opussums,and even rabbits(trees that are down in the case of rabbits) depend on dead and or hollow trees for nesting sites and shelter.
Hawks,owls, and snakes are more common in and near such natural woodland areas, and these three species are death on rats and mice.
There is plenty of good evidence that a local healthy wildlife community is good for farmers, especially in the case of the bats and certain birds.They won't stick around to help with bugs in the fields unless there is a season long or year long food supply available for times there is nothing in the fields for them to eat.Dead trees are a major source of the insects they depend onfor food.
Nowadays we select a good healthy tree here and there that is not well located or one of a less desirable species-and cut it down a few months ahead of need.
This way the wood is always perfectly sound and well started on seasoning.Deadfalls are usually well started on the road to decay and the wood in them is generally not nearly as high in heat content as it would be otherwise due to this decay..
By managing our woodland this way, we enjoy better hunting and more wildlife in general as well as some reduction in problems with rats, mice, flying insects,and so forth.
It is accepted as a general principle that the more biologically diverse a local area is, the less likely it is to suffer an explosive increase in the population of some particular species, which in the case of farmers all too often proves to be a pest.
You are right farmermac. I've seen the German forests. My situation is a bit different. I cut it when it falls on my outbuildings, roads, and in the middle of my fields, which for the most part I cut (the fields) in December to maximize nesting. My hedge rows are 100 feet wide, and I have everything from blue birds, to hawks, to turkey vultures, owls, red headed woodpeckers, egrets, you name it. And i'm surrounded by suburban track housing. But if you really like bird life, shoot on sight any ranging cat, i've seen startling numbers on how much wildlife a cat can take per year. I did salvage 4 cords of maple when PENNDOT decided to rape a village of 200 year old trees to widen the road. What I recommend to my friends starting in the woodstove world is to buy a cheap tarp to keep in their car and when they see a pile of wood by the side of the road to be bold and knock on the door and ask. works like 50% of the time. nothing like the efficiency of going to the store and also picking up 4 days of heat for free in the same trip. But this is central pennsylvania, lots of trees.
Home heating with the wood coming from a source very close by is not a bad option, but Nate is right-we don't have the amount of woodland needed , or maybe even ten percent of it, to go to a wood based bau economy.
Hauling wood long distances is energy prohititive.
But I have high hopes that if the crisis arrives in relatively slow motion, we can work wonders in upgrading our houses and buildings in terms of energy efficiency.
Personally I will probably be spending my last years in a sun room that needs no heat inputs at all during the daylight hours on sunny days, and huddled next to a very small wood burner in a very well insulated room the rest of the time.
If I were young, I would build a zero net energy house and count on selling the output of our woodlot at a good profit-enough to hire the gardening chores and housework done at least.
A Fully Insulated Passive-solar home with a Masonry Heater and Geothermal Support (Baseload Temperature Control) ..
You should be able to build one in Maine that uses less than 2 cord a year.
Just don't skimp on the first part.. and pick your location well.
I built my first biodigester yesterday out of two 55gallon drums, I'm loading it with chicken manure from my 90 chickens and grass from my lawn, I also negotiated for next to nothing a used 2500 sealed plastic barrel for the next step of upsizing. I intend to "use" it in conjunction with a new greenhouse i'm putting up on my organic farm. While i've been familiar with biodigesters since the 70s what moved me now was a factoid that came out in my online research. The liquid slurry capture substantially more (40-140%) nitrogen and phosphorus then my composting, somehow I had missed that over the years. That really changed the calculation for me, I'll use the methane to supplement the heating of the greenhouse (I just need 35 degrees) for sure, but getting more effective fertilizer is a huge bonus. I agree with the other poster that we need to conserve more of our energy, and then work on alternatives is the right order. I installed a lot of powerstrips to rid me of phantom power, and an emonitor to make me depressed on how much electricity i use per circuit and begin modifying my lifestyle.
But, I do wonder as an engineer and budding agriculturalist how much bio energy and assets can be recouped through some design changes and "routine" changes. I can make a lot of methane out of the suburbanites grass, and while i kick myself for not separating grey water from the black when i built this house in years of old, some do, and they have an ability to use a bioreactor with that input if they want gas for heating/cooking just like the millions of installations in India and China. I realize there are code issues here in the US, but I've found commercial versions of this in England. Meanwhile I'll at least save the transportation costs for the phosphates that I hope to gather for a fair semblance of free.
Which is why I think we desperately need sources like solar and wind to work out for us.
I thoroughly enjoyed this analysis. I hope that a future topic might be a critical analysis of burning biomass for direct conversion to electricity (especially woody forest material). The area where I live is experiencing a resurgence in the idea that this is going to get us off of foreign oil. I have never seen biomass or co-generation plants have all the inputs and outputs fully analyzed. Especially considering CO2 emissions and other high-nitrogen air pollution, coupled with the reduction of biomass returning to the soil cycle, I just don't get the warm feeling that these "green" energy producer seem to have.
Generally I think we've tended to be overly liquid fuel oriented in our thinking. Biomass and derived fuels are certainly part of the future, but can't be close to the whole picture. Solar plants are a far more efficient way to convert sunlight to useful energy. Electricity can perform most of the tasks we use oil for in a more direct manner. Electricity running heat pumps can replace oil furnaces, electricity can run transit systems, it can haul long distance freight. Electric car and truck technology is coming along well, especially good if combined with long haul rail options. Put your car on a high speed train in California and drive it off in the midwest. The one other component we will need is a national/international super conducting power grid. Being able to average out supply and demand over wide areas should reduce the need for storage greatly. Smart charging or even optional paid discharging of electric car batteries could additionally smooth over peaks in demand, or short term supply short falls.
The biggest use for biomass I see is as a feedstock for the petrochemical industry. Ideally much of this could be waste materials such as rice and corn stalks rather than materials diverted from the food supply. It may also provide a good store of energy for meeting any exceptional peak power demands (national heat wave).
I wonder what the total EROEI, considering all inputs such as growing and harvesting, might be, of producing Bamboo charcoal, a fast growing giant grass, in a fresnel lens solar furnace to be used as a supplement to heat a well designed passive solar house on those days when it's really cold and the sun isn't shining?
Anyone out there capable of developing an Iphone app to do such calculations on the fly? >;^)
I've got a yard of crazy bamboo, and I've been wondering how I could use it as a heat source? (It's right next to the woodpile, too! But there's not a heckuvalotta mass in that Biomass!)
I've been toying with using a chipper and making the stuff into a BioGas composting pile instead, like dear old Jean Pain! I also really like the idea of making a big steaming pile of compost that is built up against a properly made North Wall of a greenhouse or house, to support the heating of that structure. Maybe use Cinderblocks and sealant to create 'hoppers' for the material, and blanket the other side, water occasionally. http://journeytoforever.org/biofuel_library/methane_pain.html
I could probably start a local business of getting rid of Bamboo and Kudzu for neighbors and the city! Then I could finally justify converting me a Pickup to EV!
(This here's an OEM Ford Ranger EV Pickup, on 3phase AC and Nimh batts, 60mi range) http://www.evalbum.com/3387
('96 Dodge Dakota- Series DC on Lead Acids, 25mi range) http://www.evalbum.com/3381
Agree on the liquid fuels obsession....
BTW, I am a VERY recent newcomer to this site (knew aobut PO generally for a long time but only stumbled on TOD after the spill) but it seems so much of the discussion on here are alnog the lines of...
* solar cant replace oil so we are DOOMED
* wind cant replace oil so we are DOOMED
* nuclear cant replace oil so we are DOOMED
* conservation cant reduce oil so we are DOOMED
But when we have each one of these analysis in isolation, are we not making a fundamental error in assuming each is the only viable option? Is it not possible that put together the sum of solar, wind, nuclear, biofuels, and conservation are enough to equal the oil decline?
BTW, to stay true to my nickname Im compelled to point out that the deforestation article assigns an artificially low value on the efficiency of wood burning processes and value of softwoods for fuel. Done right (many don't) wood burning can be very efficient and clean.
my wood/heat analysis indicated in the assumptions that current technology/efficiency was used and also that # years of heat would be nearly doubled if softwoods were able to be used. But as you know we aren't quite set up for that (yet)
"my wood/heat analysis indicated in the assumptions that current technology/efficiency was used and also that # years of heat would be nearly doubled if softwoods were able to be used. But as you know we aren't quite set up for that (yet)"
Efficiency:
I saw the 55% efficiency number... where did you get that from?
Its about right for the old "smoke dragon" airtight stoves of the 70's that folks would choke down and smolder all night billowing smoke for miles... But anything built since the new US-EPA standards went into effect 20+ years ago is capable of at least 70% when operated properly. I use a Vermont Castings catalytic stove (not the best by far) and one big armload of wood will burn overnight if I turn it down low, all the while you see nothing but a few wisps of steam from the chimney.
Wood gassification boilers are even better. Units such as the GARN and TARM have efficiencies in the 75-85% range. They use forced air and highly insulated reburn chambers to burn off the smoke at 2000F and then very long flue passages to extract the heat into the boiler water. These units have exhaust cool enough to stick your hand into the stack without getting burned - just like a mod-con gas boiler.
Softwoods:
Its a common misbelief that burning softwoods is what causes creosote. Creosote accumulates when the unburned volatiles in wood smoke cool below about 250F and condense on the walls of a chimney. The true culprit in creosote formation is burning wet wood - boiling off the water content creates a large percentage of steam in the flue gasses which cools the chimney down below that 250F condensation point for creosote formation.
There are two ways to avoid creosote - keep the chimney hot or don't make smoke. All modern efficient woodstoves and boilers reburn the smoke at high temperature to achieve high efficiency - this reburn eliminates most of the volatiles that lead to creosote formation. So long as its properly dried you absolutely can burn softwood without significantly higher risk of creosote. In the pacific northwest and Canada a lot of folks heat with wood burning nothing but Pine, Fir, ett and seem to get by just fine. They just burn more wood.
The downside of wood of course is that operating these units to potential is VERY labor intensive and most folks don't have the desire or even know how (many get bad info from the dealers who sell them firewood) So they burn green wood, make a lot of smoke, and contribute to the reputation of wood heating as dirty and inefficient.
Granted all this doesn't change the fact that we would need an prohibitively large area to convert every home to wood, but I think its doable to convert some small percentage (~10% ?) with high efficiency units and sustainably harvested woodlands (assuming 3-5 cords/house/yr and 1-2 acres per cord of annual harvest). I haven't done any math just a WAG.
[Ive probably just repeated half the comments in the original article but oh well...]
Hi Wood Heat. Welcome!
I've been thinking about making a Solar Woodshed lately, as well.. use Passivesolar design to help cure my firewood a bit more thoroughly..
My Mom built two houses in Maine with Masonry Stoves in them, very efficient, kept the demand for cutting/splitting and carrying (and ash removal) way down! Mix that with proper Solar Design and Insulation, and you'd barely need to run the stove..
Bob Fiske
Portland, Maine
johukl,
If you have a sunny spot you can build a very effective shed /dryer for your wood very easily and cheaply indeed.
We store our wood in farm sheds built out of rough cut posts and lumber covered with salvaged roofing/siding sheet metal;cover the ground with gravel , plastic sheeting or whatever is handy to keep siol moisture away, leave a six inch vent space around the bottom of the walls, and couple of inches around the top.Make barn type swing doors of the same materials.Paint it any dark color, we like red and green for aesthetic reasons.
The idea is to have slow but steady passive air circulation;interior temperatures will go to forty degrees F over outside ambient after a few hours of bright sun in this area.
Stick length wood will dry in a few months-well under a year- to the point of equilibrium-moisture content will actually increase slightly during extended rainy spells, as verified personally by wieghing individual sticks on sensitive scales.
I suspect wood is one of the few dense-mass products which can be placed in a reasonably small drying shed. The aformentioned bamboo would require a larger shed for the same amount of mass, or a taller shed. I think the issue of drying biomass is a big issue. Even in biogas generation a wet gas is primarily the product.
Jumper,
You may be correct, but I am not in possession of the figures or the expertise needed to run them for other biomass.
Air circulates adequately around firewood sticks, either split or still in the round, and the sticks have adequate surface area in relation to the volume or mass to dry fairly fast.
Wood sheds are generally twelve by twelve by eight or smaller in this area, and the heat gain from exposure to the sun is ample to dry the wood, considering that on low humidity days it dries without assistance anyway.
Circulation and heat gain per unit volume of wood might be a problem if the sheds were to be much larger.A twelve footer holds an adequate to ample annual supply for most folks in this area.
Actually most are enclosed only three sides and the wood still drys just fine in six months are less.
Such sheds cannot be used to dry wet or green crop residues or hay baled too wet;these materials rot due to inadequate circulation and may catch fire due to spontaneous combustion.
Thanks for the thoughts, Mac.
I might just use the bamboo to make some whistles for the tourists.. Maine seems to be getting all the folks who didn't manage Europe this year, although a significant Builder just went Chap 11 today, and the LNG terminal lost its funding. Just keep the tourists coming.. we're about to send the next chunk of rail up to Brunswick (and there's working track up to Rockland, I think)
Get your whistles here! AU-thentic Maine Train Whistles from sustainably harvested 2nd Generation Maine Bamboo!
try a Rocket Stove ...
http://www.aprovecho.org/lab/index.php
http://www.richsoil.com/rocket-stove-mass-heater.jsp
It's definitely on the list. Waiting for the opportunity to try it out.
I have been reluctant to bring any bamboo onto our place, seeing that it is as hard to get rid of as kudzu once established.
But one of my buddies down in the piedmont is using it for garden stakes, poles and temporary fencing.
He just makes sure it is dead, dead, dead! before he moves it to a new spot.
It lasts a long time and he is well pleased with the ease of working with it for these purposes and may try building a chicken coop or other small open farm building out of bamboo soon.
It burns just fine but you can't chop it with an axe or anything else once it is dry and hard.
But you can cut it to stick length quickly and easily with an electric reciprocating saw of the sort used by carpenters, plumbers and other trades people.My buddy uses a fourteen teeth per inch soft back metal cutting blade for this job and he burns any leftovers in his wood stove.
I have no doubt that when the day comes we can't buy diesel and all the stuff they sell at Lowes we will find bamboo as useful on American farms as it is in Asia.
Bamboo sprouts are also very good eating.
In answer to your question, there has been some work on this.
See Stabilisation wedges
for a discussion regarding stabilisation wedges and Professor David Mackay's work on sustainable energy
Sustainable Energy - Without the Hot Air
"But when we have each one of these analysis in isolation, are we not making a fundamental error in assuming each is the only viable option? Is it not possible that put together the sum of solar, wind, nuclear, biofuels, and conservation are enough to equal the oil decline?"
Agreed. I think that this site lives off the fears of people imagining the worst. Despite the negativity, there still is a lot of good information on this site.
Liquid fuel demand and building space heating/cooling energy consumption can be 1/4th of what it is today in the US and we can still have a thriving economy. Getting there, though, will take a lot of adjustments to our way of life. It seems that fuel prices will need to drive us to make the changes because I keep seeing too many people who want to live business-as-usual.
Retsel
"Agreed. I think that this site lives off the fears of people imagining the worst. Despite the negativity, there still is a lot of good information on this site."
I sense a lot of pessimism here, but I don't know if I'd go that far. Its not LATOC ;)
But I think the pessimistic attitude is a handicap when you try to convince the general public about the reality of these issues. The sad fact is that you have to expose people slowly... almost subconsiously. Most folks are so ignorant they just cant digest this information. Hit them with the worst case scenario all at once and the reaction is denial or "nothing I could do would make a differnece so why should I care" (heck today at lunch we were talking about the oil spill and I actually found myself explaining to a couple of coworkers why fossil fuels are called "fossil"... they had no idea where gasoline comes from... and this is a tech company)
That being said I think this place serves a critical need. These are issues that need to be discussed. We need to be working on solutions. Its going to be a while before society at large takes them seriously but I suspect that when our backs are up against the wall we will be amazed at how fast people can change and how fast technologies/alternatives will be developed (the often mentioned WWII arsenal of democracy buildup example). When that day comes we will stand a better chance if we already have the ideas ready to be tried.
The sad part is that it will take a crisis that literally puts our very survival at risk to bring about this revolution.
East of Eden, said by Sam Hamilton (?) from memory.. 1982
'When your dog has eaten strychnine, take him out back with an axe and chop his tail off, the shock will either kill him or force him to throw up the poison.. ' (In telling Adam about Kate)
http://www.pinkmonkey.com/booknotes/monkeynotes/pmEastEden47.asp ..
Me thinks diggressingly political domination is what lives of fears of people imagining the worst. Also there are loads of valid fears; fear is reasonably part of human biology and the proposition is to be scared right.
Dunno, this part sounds like quality advocacy mostly done by the book. No one is claiming genocide yet...
Back to diagonal reading.
... for the unaware, sociology professor Jean Ziegler did it. A butchery. Oops, apologies to butchers.
We either ramp up solar, wind,and nuclear, or it is curtains for life as we know it in the rich countries..
Well said.
However, I don't think we can wait for policy to do this. Individuals need to bite the bullet and do it themselves to the best of their financial ability and as individual circumstances allow. Lazy doesn't count. Then, when the next swing occurs people say, "do you see what ______ has done? I think we should do that". Or, "why aren't more doing____?" If you have knowledge of this subject....PO, and most do not beyond bitching about gas prices, then your example can really help.
I do like the solar tractor! And I do think it should be light weight!
I want to put in a windmill visible from the road that goes to our village and local port. Every time people see it they will notice and comment. There is a small windmill on a helicopter hangar up the coast and CBC did a special on it.
Paul
Individuals would be much better off substantially cutting their energy consumption (insulating house, moving closer to work, better gas mileage car, lifestyle changes...) than in trying to develop their own energy sources.
I agree wholeheartedly...except when it comes to solar power, which is something individuals can add to their homes with relatively the same effort and benefit as any of the things you've suggested.
False Choice.
You need both. You need power to heat homes, cool food, cook food, wash, communicate, have lights etc.. AND we very seriously need to learn how to do these things with much less overall power than we have been doing them in recent decades.
Neither is impossible.
No one believes that biofuels are a panacea. They will become an important source of transportation fuel as oil prices increase, and therefore the type of calculations presented here are necessary. I can't speak to the EROI assumptions, except to point out that they depend heavily on process and technology. Burning biomass for heat or electricity is probably an inefficient way to use it, unless there is a highly efficient CHP system. At present, wind is competitive for that market, and of course coal and natural gas, but the GHG and cost issues will become significant soon.
Conversion of biomass to cellulosic biofuels via biosugars potentially offers higher efficiencies and GHG reductions. See the Kammen and Wang LCA links at http://www.generalbiomass.com/globalwtech1.htm . A couple of tech areas offer the prospect of improvement. First is the catalytic polymerization of 5- or 6-carbon sugar molecules to form renewable gasoline, diesel and jet fuel, or intermediate feedstocks like gamma-valerolactone. The idea here is to retain the C-C polymers already produced and join them together, vs FT gasification which blasts them apart. See Virent and Dumesic lab at Univ. Wisconsin. Fermentation of biosugars to produce drop-in biofuels (e.g. Solazyme, Amyris) is also possible, but not yet feasible in North America due to a lack of cheap biosugars.
The other area for improvement of sugar-based systems is pretreatment, the process of preparing biomass for enzymatic hydrolysis. Current pretreatments, e.g. steam explosion or dilute acid prehydrolysis, use a lot of heat to make steam or heat water to 180-200 degC. These can potentially be replaced by milder methods using advanced enzymes. Enzymes in general are very energy efficient, which is part of their evolutionary function.
Thank you, thank you for this great work. It needs wide dissemination, especially to Congress and to Greens and good government groups. It is solidly researched and clearly scientific. However, the institutional parasites feeding on the Biomass Humbug are already writing their furious replies. Standby for assault
First: Biofuels. Among the many alternate energy schemes to replace petroleum as a fuel for our massive ICE auto-fleet, biofuels are surely the most malevolent.
A big part of the reason as this report demonstrates is net energy. None of them have enough net to make them truly viable. Some small-scale projects for farm and forestry uses can and do prove useful. But there is a huge petroleum subsidy in all of the large-scale biofuel schemes. Total production costs figured in BTUs and not dollars are the only honest way to measure the true productivity of any fuel. Dollar measurements are a shifting illusion, a shell game because the “dollar value” of differing fuels are constantly shifting. Measured honestly by the strict metric of EIEO, no biofuel scheme is productive enough to justify the resources we are pouring into them. And let me get way on the limb, here: because of the 2nd law of thermodynamics, few, if any, will ever be much better than “break-even” propositions.
HOWEVER, that said… there is now a huge bureaucracy in the state and federal agriculture departments and universities, in alliance with and supported by large industries, and big and little “agribusiness,” and therefore by congressional and legislative bobbleheads to provide a strong claque of “non-partisan” support for this on-going insanity. So the vain pursuit of Biofuels will continue to waste resources on a phantom solution to the search for an alternative fuel for our beloved cars. And in truth our biofuel delusions are probably no more stupid than the miles of highway we continue to build, or the auto company bailouts, or a long list of other BAU boondoggles we are determined to pursue.
But the biofuel delusion is worse than simply a waste of money, time and energy.
Beyond the fact that biomass-fueled cars are an energy resource illusion, there is an even more potent reason for derailing this institutional insanity. Quite simply as parts of this report discuss, a huge part of our present agricultural practices mine the soil. They are, therefore, neither renewable nor sustainable We will turn this planet into a desert even faster than we are already doing if we pursue many of the biomass gimmicks now being advanced – especially if we try to run the global auto fleet on “biomass.”
That won’t keep us from trying.
The latest hot biofuel lunacy to marshal the bobblehead support of bankrupt governments is “cellulosic ethanol.” There are few honest foresters these days, but if there were they would tell us that many of the proposed forestry practices are neither renewable nor sustainable. This report confirms that. Instead of returning forest wastes to the forest floor we are going to cut the trees and sweep the forest clean. Oh really? And what is going to sustain the growth of the next generation of trees?
Are we becoming such a nation of self-deluded humbugs that cynical boondoggles don’t even rile us anymore? What is sad are the legions of empty-headed Greenies who have been docilely led into cheering for “Biomass” as a renewable/sustainable energy resource. It is not!
A sure recipe for turning this nation into a denuded wasteland is to try to run our vast auto fleet on pine plantations, and hardwood forests, and “trashy scrub underbrush,” and corn, and switch grass, and algae and eucalyptus and lawn clippings.
In the first report some clowns were even advocating …animal fat! Buy pork bellies, bubba! We gonna run our pickups on ‘em. Yeehaa!
Are we insane? Yes.
God help our grandchildren.
Cap’n Daddy
xxx
Excellent post, Cap’n Daddy; you aren't exaggerating or merely using rhetoric when you call bio-fuels "insane." The idea of, in effect, burning food as we bebop around town to Walmart, or drop the kids off at soccer practice, or commute our 60 miles to our busywork employment is so monstrous in its absurdity that no other word will do.
"We can't burn food when there are people are starving!"
"We're providing for the energy needs of mankind." And they blink.
"We can't deplete our topsoil further! The fungal and microbial processes that created it took centuries to work!"
"We're saving the planet." And they blink.
The USDA has determined that the USA has a sustainable biomass potential of 1.3 billion tons of biomass or about 19 quads of input fuel.
They also have determined that we can get 1.9 billion barrels of oil equivalent or 10.6 quads of liquid fuel out of 3.5 billion barrels equivalent of energy input(19 quads).
If the liquid fuel is cellulosic ethanol only ~10% of the input of the input energy comes from fossil fuels.
http://www.nrel.gov/biomass/pdfs/39436.pdf
As usual EROI doesn't distinguish between fuel uses and so btus for heating houses are ranked equal with btus fueling cars. Dumb.
First we in the USA don't need 100 quads of energy to maintain BAU we need about 18 quads of gas for heating, 14 quads of electricity, 28 quads of liquid fuel for transport and 6 quads for products(plastics fertilizer, etc.) The rest is energy loss.
If we double the efficiency of our transport and building heating/cooling systems we go from 66 quads to 43 quads of energy we use.
If we raise our share of electricity from renewables from 9% to 25% we would save an additional 8 quads of energy without sacrificing our lifestyle(other than junking the SUVs, hot rods and McMansions which are going away anyways).
Our reduced input would look like this;
10 quads(per year) biofuels + 11 quads of oil + 15 quads of gas
+ 18 quads of coal +9 quads of nuclear fuel +3.5 quads of renewable electricity = 66.5 quads input
to get 14 quads of electricity, 9 quads of heat and
14 quads of transport fuel=37 quads output. Non-sustainable fuels(fossil plus nuclear) are reduced from 93 quads to 52 quads. CO2 emitting inputs drop from 82 quads to 43 quads.
In this more efficient world, 75% of the input energy(31.5 quads out of 43 quads) goes to making electricity which has huge inherent, unavoidable losses(20 quads).
These losses are due in part to our desire for instant power always available, something renewables can't help us with. It's the reason we build huge power plants, dangerous nukes and climate changing coal plants.
At that point we get off the grid with local storage by batteries and hydrogen and go 100% renewable but that can wait for a century as we have enough fossil energy to last us till then. Europe will have to go down this road sooner.
Forget your strawman expectations.
Renewables can't replace fossil fuels?
Fossil( and nuclear) fuels can't replace fossil fuels (and nuclear)either.
It's called overshoot.
One lonely brave dissenter, Biodoc. Even Majorian agrees that we cannot maintain BAU by burning down the biosphere.
The easiest argument here is the energy hierarchy, and the case has been made previously. Many calories of solar energy are required to make a calorie of plants or phytoplankton, and many calories of plants are required to make a calorie of consumers or animal plankton, and many calories are required to make a calorie of the next critter, and so on up the food chain and then into human systems. These transformation result in decreasing flows of available energy in each successive transformation, and increases in quality (transformity) with each step. The figure below illustrates the relative transformity of each step on the basis of sunlight.
http://dieoff.org/page170.htm
As you can see, the relative value of animals, proteins, and soils are lumped together as a value of 10^6 to 10^9, while human systems transform at a level of 10^12 to 10^32 for the internet, perhaps. This means that unless we can do much, much better than Mother Nature, and are able to not only supplant Mother Nature in all that she does in terms of ecosystem services that we rely on, but also to improve on her abilities, we will be unable to have any of the trappings of modern man. Here is a journal article from a group of people who clearly have not done the math, do not understand their energetic limits, or are in denial.
http://www.greenaironline.com/news.php?viewStory=206
Anyone who thinks we can replace anything remotely resembling modern civilization with biofuels doesn’t understand the limitations of energy laws and limitations of material cycling. And as the authors of the OP point out, a transition to even partial reliance on biofuels would cause fairly immediate immolation of the planet’s biosphere in a massive conflagration of heat and displacement of material cycles, due to the human population overshoot. And our overshoot is massive, due to the fact that our potatoes are now made of oil. Any delusion about biofuels is being currently corrected in the case of fossil fuel decline, as those contributions of mother nature in terms of primary energy production are being increasingly requisitioned to feed the population rather than fly our planes. We are backed into the corner, with no way out. The authors may be too optimistic in their conclusions because of these issues, particularly overshoot.
One small issue that I would like to elaborate is the interchanging use of the term primary production of energy. The authors use the term to describe both nature’s production of biomass and also human use of fossil fuels (Europe as example). This is confusing, as it suggests that there is no hierarchy which human systems are subject to—that man can escape the energy laws and make its own rules because it has found this secret treasure trove of fossil fuels.
At least Nate's strawman fooled you, Iaato.
It took millions of years of sunlight to create fossil fuels which BAU will gobble up in a couple of centuries. How could a years worth of solar energy (all renewables comes from the sun)completely offset a thousand years of ancient sunshine?
Does that mean renewables can't satisfy
a reasonable level of consumption?
Of course, not.
In fact there are nice countries on Earth today that use 10% of the per capita energy of energy-gulping americans.
What Nate and Hannes are doing is putting words into the mouths of renewable supporters.
It's really a disingenuous method of argument, reminiscent of Lomborg.
The real discussion should be about what is a reasonable, sustainable level of energy use.
Instead we get a Faux-style discussion about those wacky renewables guys who can't add.
May I offer some food for thought here?
Your first point about the millions of years of sunlight drives our point home.
Now if you read all the papers that circulate about the potential of renewable energies, look at newspaper articles about the "green economy" and see where we spend billions of dollars, euros, pounds and what not, I would say that almost all the effort of societies now goes into the idea of "keeping what we have, but without fossil fuels".
We have no doubt that renewable energy is the thing of the future, but not likely even remotely in a way we use energy today, neither in quality, nor in quantity, and even 10% of what the U.S. uses is going to be a stretch.
So while this knowledge might be in your head, it isn't in many other people's, who currently want societies to spend money they don't have, on things that won't work. That's what we're trying to debunk, not the idea that we will have to make do with what's left.
But unfortunately, before you can seriously discuss what that would be, you need people to get real. As long as there is common belief that we can do what we both know we cannot, there is no chance that enough effort goes towards those unattractive things that really work.
You're wrong again.
Renewables will be part of our energy future and people need to work on them.
This is the opposite to your 'debunking/ reality therapy'.
You don't represent anything positive.
Your paper gets a zero for creativity and communication.
It's also true that we're all going to die someday.
Why can't you understand that that particular fact is 'irrelevant'?
It's an important discussion and it needs the major debunking this article provides.
The University near me is building a major research center to house about 300 scientists to investigate and further bio-fuels.
Tens of millions of dollars are chasing this concept. The University is not about to publish an analysis similar to what's been provided here... there's simply too much grant money is at stake.
Once you bias the University scene, it gets a lot harder to find useful direction.
http://host.madison.com/wsj/news/local/environment/article_7b19807c-9200...
A good question. But easily answered. The efficiency of the geologic system, sunlight=>biomass=>buried organic sediment=>remains trapped for millions of years, is quite low. Each => representd at least a factor of ten reduction in quantity. I guess the creator wasn't concerned about the efficiency with which his planet created usable fossil fuel. The planet just wasn't optimized with that in mind. All the fossil fuels on earth amount to far less than a month of sunlight. The trick is collecting it.
Rapidly increasing the death rate of our species BEFORE we draw down resources would greatly extend our ability to manage long term our resource base. This is just a raw fact whatever the implications this statement entails.
We're well stocked up for the first few months at least of siege, famine and mad max in general, including plenty of fuel, staple foods suited to long term storage, and lots of ammo-hopefully for trade but for actual use if absolutely necessary.
we have a well as well as gravity fed spring water.
I'm still hopeful that such preparations will prove to be unnecessary over my remaining life span , but ......
Are you ready?
I don't understand this philosophy: if things get so bad they break down as you think possible I don't see how they will get fixed.
So in other words you need to prepare for living not a few months of crisis but for the rest of your life that way.
Yes-the first few months will be the worst;epidemics , civil wars, riots, famines, and all sorts of FOUR HORSEMEN type stuff tend to burn out according to natural processes.
After a year or so, I expect we can probably work the fields in reasonable safety in a small group with one of us taking turns as an armed watchman.
If we- meaning my family- make it thru the first major upheavals I can go back to farming like my Great Grandfather did, and we will be able to feed our own grandchildren and ourselves.
Since I had a strong taste of the lifestyle as a youngster, I think we can handle it, although the younger family members may have to be left to starve a few days to convince them of the necessity of working with thier hands and backs.
Like I said before, I hope this does not come to pass-but only someone who does not grasp the entirity of the economic and ecological situation could believe that it cannot happen.
My guess is that the odds over the next decade or so are as serious as the odds of my having a heart attack personally,or getting a fatal cancer.
The longer term odds are appalling in the opinion of most biologists and other scientists who have studied the issue.
I'm not so sure about your view of the chaos lasting only for a few months. I'm reading a book called "A Little History of The World" by E. H. Gombrich. It's an interesting but rather sad read (so far), limited resouces and productivity has seen the ambitious and cunning exploit the week. They claim the land, force people into slavory/serfdom, raise armies, plunder and kill. This happened for thousands of years, perhaps it's still the case. I don't feel very free being forced to work to pay taxes, but I am aware my standard of living is very good, but this is the result of FF. I don't see much point in trying to build a house/farm to weather the storm, not when a bigger group of better armed people will turn up and take the place by force. Humans are not the most pleasent of creatures.
The WORST of the chaos will probably only last a few months.After that the odds of being shot at random or kidnapped or robbed for your possessions will decline dramatically as the population falls off fast and the transportation system collapses.
I'm not much worried about starving people from New York walking to southwest Virginia in great numbers. A lot will start, but not many will get here.
I agree with the rest of your comment, generally speaking.
If there is a general collapse, and we survive the initial chaos, I will have to make an alliance with some local leader who indeed may be a warlord.I Just hope I choose wisely, or get lucky, and pick a winner.
Hopefully we can be a step up from peasents in the new power structure;one thing is for sure.
Being old, I don't have a lot to lose, personally.So long as I and a lot of others of my kind possess a few good weapons, a nickel and dime local warlord will necessarily have to refrain from the worst of abuses.
Old William Tell or Robin Hood had to get pretty close to use a bow, but any capable marksman can kill a pesky warlord from a quarter mile away with a good deer rifle.
Or I might just keep my mouth shut, a job in his stables working for my gruel, and burn him out some windy night when it is nice and dry..There won't be any fire trucks....
Scots Irish hillbillies have long historical memories and I have read way too many history books and novels. ;)
Of course after a while the sobs will have everybody cowed and licking thier feet again, the way it is now in most of the undeveloped world.
But by then I will be dead.
Following the naming conventions, it might sound inappropriate to suggest that Robin Hood constituted one of the 'Good Warlords' ..
That term draws us towards Mad Max imagery pretty quickly, but aren't Bush and Obama Warlords as well? What leader with an Army at their command is not one?
'Ultimately, all is Character'
Most of human existence has been brutal and short, why do you think it will ever revert to what you consider the norm today?
Another way of comparing energy sources is by land use:
(Graph from Energy Sprawl or Energy Efficiency: Climate Policy Impacts on Natural Habitat for the United States of America (McDonald et al/PLoS ONE))
Biomass not looking good...
I just looked at the McDonald paper, and would note that one person's Energy Sprawl is another person's Distributed Energy. Yes, giving up fossil fuels will require using more land area. This actually has a number of social and economic advantages - it creates local jobs and reduces energy losses due to distribution (I squared R heat loss for electricity, no matter how smart the grid, and diesel to transport coal, etc.).
The issue is how to balance the tradeoffs and improve efficiency in every part of the system. Solar is still very expensive and has no intrinsic storage mechanism. Liquid fuels from biomass inherently store energy like their fossil counterparts in C-C and C-H bonds, they fit existing infrastructure, and conceivably can be profitable, allowing growth, jobs and investment. To ignore biofuels is to accept BAU, and we have already run out of time re peak oil and global warming. See my earlier post in this thread on new technology developments which could improve the efficiency of biomass to biofuels conversion and discussion at http://www.generalbiomass.com/globalwtech1.htm . These technologies (catalytic conversion of biosugars, low energy pretreatments) or others have the potential to change the length of the bars in the McDonald graphs. It won't happen without innovation, investment and a willingness to work with the problems that new technologies always present.
Finally, as a biologist, let me note that "inefficient" green plants produce hundreds of billions of tons of cellulose every year, and millions of other organisms consume that cellulose and dead biomass for energy and materials. We can tap into that system in a nondestructive way and give some of that material a trip through our transportation system, sparing the use of very large amounts of oil.
Strawman argument!
Disclaimer, I work with Solar PV. I disagree. The problem as I see it is incorrectly set expectations and a tendency to continue thinking within the box and expecting solar to work in the context of BAU. That of course will never work!
I'll give a link to one example. Personal transportation in our current BAU world is a huge waste of energy. If you want Solar to power 5 passenger sedans that drive 300 miles at a charge that is a completely unrealistic expectation!
However if you think like this:
http://www.pnwlocalnews.com/sanjuans/isj/business/89546537.html
It becomes very realistic. The problem is if you present such an alternative to most people they balk and call it unrealistic. They say things like the average American will never go for something like that... Personally I'd like to hit these people upside the head with 2 X 4's! Who do you think is really being unrealistic?!
The concept of profits and growth *IS* the epitome of BAU and is patently unsustainable on a finite planet. I don't think I should have to explain why to a biologist. It most certainly can not be sustained with biofuels with current human population and continued growth.
I grew up in Brazil during the time they were implementing the sugarcane ethanol program, I benefitted from it personally and drove 100% ethanol powered vehicles for many years, so I am not opposed to biofuel usage per se and see a place for it in the overall energy mix, however having said that if we don't find a way to change the paradigm of growth being the goal and being a good thing and if we don't get serious about the population problem then I'd say we are FUBAR and everything else is moot.
I'd like to see some NEV penetration myself. In fact I'd like to see a slightly expanded NEV2 authorization
that allows them on 35mph roads. In pennsylvania my understanding is that a souped up NEV that does 35mph is legal, meanwhile for the farm and neighbors I use the Polaris EV 4x4. It is a reasonable solar charging device as it appears to only eat 1kw of AC, with a proported 50 mile range (and on 35mph roads) that would be
about 95% of my driving. I've only had it for two weeks, but the interesting test will be this winter in Central PA.
I respect your contribution to the debate. I'll restrict myself to picking on one point, albeit rather a severe and fundamental criticism, and there was much in your article that I do agree with - in particular the myth of cellulosic transport fuels.
My question is, where on earth did you get that biomass to electricity conversion rate of 20- 25% from? I mean, what on earth can have led you to believe that biomass has an intrinsic conversion efficiency of half that of coal? Are you working under some 4th law of thermodynamics that no one else knows about?
In Europe we have for many years been co-firing biomass at 36-44% net conversion efficiency. Some of those boilers have already been converted to burn 100% biomass, with an efficiency loss of about 1%.
Yes, conversion efficiencies of very small boilers are low (just as they would be if fuelled with coal), and they do account for a lot of historic biomass power, but that is mainly because they use very low steam temperatures and few or no reheats, due to their scale. Proper scale biomass boilers (200MW+) use steam temperatures in excess of 550 degrees C and have several reheats. Biomass is not yet ready to go supercritical but it likely will be within a decade or so and will then achieve up to 45% net efficiency.
Large new Circulating Fluidised Bed boilers being designed now will achieve >40% net efficiency. Companies such as Dong Energy (national Danish energy champions and global pioneers of co-firing and offshore wind amongst other things), RWE and EOn (both German - largest and third largest energy utilities in Europe) and GDF Suez (huge French utility, 35% state owned) all have large dedicated biomass projects under development (the GDF one is financed and under construction now) based on single boiler/steam turbine trains of at least 200MW. Do you think all these companies are stupid? The economics could never even possibly work at those efficiencies.
I guess I've made my point now, so will stop, but......honestly.....
Is it possible that you're looking at combined cycle (heat+electricity) when talking about 40-45%?
Okay so just to review... the author of this article thinks biomass to electricity conversion occurs at 20- 25% efficiency... and co-generation tops out at 45%...
I think that pretty much sums up the scientific validity of everything that has been posted on TOD by Mr. Kunz.
Umm...No - that's not how it works. But with this much data Id be surprised if there weren't errors. If so please point them out and try to be constructive. Are you a biomass to electricity expert? If so share some facts/links on what is missing.
Okay Nate if that blatant slip up isn't enough for you to dismiss it all feel free to waste your time systematically disproving all of it piece by piece if wish. It is all junk science born out a preexisting point of view followed up with a string of poorly sourced (often incorrect) numbers chosen by the author to arrive at the desired (and bunk) conclusions.
Well the author is certainly NOT wrong about the abysmally poor rate of solar-energy-to-fuel-energy conversion of the photosynthesis process, compared to eg. Solar-thermal-electricity plants or even photovoltaic PV installations. Given that FACT, I see no reason to keep large-scale biomass on any table for consideration in any forseeable future. So what was it you were pitching again?
The inefficiency of photosynthesis is irrelevant. In temperate climates the EROI of well designed biomass to co-firing or co-gen operations are 10 times the best EROI number you will ever get from PV and solar thermal electricity.
Why exactly is it you think that efficiency of conversion of solar energy to desired energy form is irrelevant? That seems to me to be a very strange position, given the RELEVANCE of 1) land area required to produce a given level of output 2) resource inputs increasing as land area required increases.
The bottom line is, if you want a given amount of electrical output from an energy project, you have among others, the choice of installing 1 unit area of solar thermal collectors or 300 unit areas of biomass. Same output.
Are you claiming that this combination of assertions is true even if the harvesting of biomass is sustainable? (i.e. harvesting doesn't reduce the prevalence of the ecosystems that produce biomass?)
Agroforester - I am not a biomass expert (and neither is Hannes). However, I have worked closely with the man and his team during the last year and he is one of the smartest people I've ever met (and I include alot of big thinkers in that list). He also is co-author with me on 2 papers on energy. Despite his intelligence he sometimes makes mistakes, (though far fewer than I have) which is why I invited you to spell out exactly what was missing with that part of the analysis. To say that he lacks scientific credentials is pretty absurd. But I did expect this essay to raise the hackles of those with vested interest in scaling forest products for energy and it obviously has. I am no longer surprised at the vitriol expressed in these threads.
I think the point of the article (and series of articles) isn't that biomass/nuclear/wind are bad, but more of how coal/ng/oil have been energetic picie dust and new ways or organizing our lives will have to take precedence over building solar based mousetraps. And to be clear, I think biomass will play a vital role in future societies - it just won't be at the global scale that is till being planned. At issue isn't whether you or I can enjoy/be successful at forest gardening etc but whether it can scale (with other things) to continue a growth based culture. I don't think it can.
Agroforester, I share your anger, but the only way to harness it is to stick to a rational discourse and see it through. We can get there, one hard fought, bloody cold, rational argument at a time.
Nate, to be fair, I have read articles of yours for some time and your desire for the world to be a certain way shines through loud and clear. Is it possible that you should reconsider when accusing others of bias? Heck, is it even possible that Agroforester has a point? You know the gentleman who wrote this article, can you really, honestly, state, that he came to it with no preconceptions at all?
No - I can't state that - but I can state that even if the author(s) were completely wrong on their efficiency assumptions on one piece of this analysis it wouldn't invalidate other aspects of their scientific research as was claimed by Agroforester
And I'm the first to admit I'm biased - but I'm also right..;-)
Im not the author of this piece and all Ive written here in the past year or so is not analysis but closer to philosophy - as I think that is what is needed. But as someone who has volunteered thousands of hours on this site in order to host a civil discussion about energy and our future I feel I have the right to defend guest posters, irrespective of whether they are colleagues or not.
Sure, no one's denying rights, freedom of speech cuts all ways and shouldn't need to be brought up in an internet conversation. It’s a great website and it is valuable to hear a different perspective whether one agrees or not, just not so valuable when data presented as well researched fact are just wrong. Thanks for your honesty anyhow.
I'm also right
I respect your good intentions, Nate, but your basic assumptions are highly unrealistic.
We do not face "Peak Energy" due to any physical limits. Wind, solar, nuclear, etc are scalable, sufficiently high E-ROI, and affordable. The cost of dealing with intermittency is relatively minor if you do it with things that make sense, like Demand Side Management, overbuilding, geographic diversity, and minor biomass or synthetic hydrocarbon backup, rather than inappropriate strategies like utility-scale batteries.
We are happy to look at those numbers, but unfortunately, I can't find anything that's near the above assumption suggested by Hoover.
Dong Energy works - to my knowledge - on CHP plants for waste disposal (which is close enough to biomass overall, and mentioned in our article). They get to about 60% net efficiency (after heat and electricity used for the process), which is what we state in table 3). But that usually includes gross available heat output, which typically can't be used due to the fact that there isn't enough demand in summer.
It is true that co-firing a certain percentage of wood with coal doesn't significantly diminish the overall efficiency of coal. Having said that, coal is also not overly efficient, on average, we generate electricity with around 31-35% thermal efficiency from coal.
So simply put: We would love to review those processes producing electricity from biomass at >40% efficiency, please provide us with some links or papers. We are happy to admit mistakes and look at avenues not yet analyzed.
Hannes, thank you for being open minded. You will not find much of that data on the internet, companies tend to guard their power station's efficiencies quite jealously, and treat it as commercially sensitive information, especially when pushing the boundaries in a new field like biomass. That's where real life industry expertise comes in. This may be a shock, but there are limits to what an academic can achieve with a web browser.
There are a few things I can help with a little.
Firstly, modern supercritical coal boilers have an efficiency of up to 46% (for advanced super critical). No new coal plant would be built at 31% efficiency, those are legacy plants, even in China the norm is well above this, search back a few days on TOD there was an article by Euan Mearns on Chinese coal with quite a lot of debate about efficiencies. Otherwise, here is a paper which I googled:
http://web.mit.edu/mitei/docs/reports/beer-emissions.pdf
Good coal plant can get to about 40% even without being supercritical. Drax in Yorkshire will be almost 40% after its turbine replant and that is 30 years old, and not supercrit:
http://www.draxpower.com/corporate_responsibility/climatechange/efficiency/
To get data on Biomass will be more of a struggle. The oldest large scale CFB that can burn 100% biomass and can fully condense is Alholmens Kraft in Finland.
This is quite a nice report:
http://www.biocap.ca/files/reports/MPB_Study_Finland_Trip_Report.pdf
It is a far from perfect example since in operation the plant burns a mix of peat coal and biomass (for economic reasons), and is also optimised around combined heat and power rather than full condensing for power. Nevertheless, the report clearly states that the plant is performance tested at 100% biomass (and I have been there twice and can confirm), it has a gross efficiency in full condensing mode of 38 - 39% and parasitic load of 14 MW, giving a net efficiency of 33 - 34% but I stress that is for a plant that is designed for CHP and therefore has no reheats and does not optimise its potential for power generation. The important thing is that it can burn 100% biomass at steam temperatures of 545 degrees C in the superheater. Once you have that you can achieve the same efficiencies as a big modern coal plant (like Drax). More, actually, because there is hardly any sulphur in the wood and therefore not the energy cost of desulphurisation (1 - 1.5%).
In this paper by Foster Wheeler
http://www.fwc.com/publications/tech_papers/files/TP_CFB_04_01.pdf
you can see that a 20 MW biomass boiler can reach 36% (gross) efficiency with only one re-heat and at only 480 degrees C. A 300MW boiler can have 4 or even 5 reheats and steam temps of up to 545 degs C, maybe more soon.
Here's another very good paper by Foster Wheeler on CFBs:
http://www.fwc.com/publications/tech_papers/files/TP_CFB_03_01.pdf
Unfortunately it doesn't tell you what the net conversion efficiency is because FW only make boilers not steam turbines. You will have to find someone you trust who knows something about steam turbines to translate the steam parameters into efficiency.
It will be several years before the projects being developed now are commissioned, and probably several years after that until the academic community wakes up to what is happening. By then the first supercritical biomass plants will be on the drawing board. This is the scale and the efficiency on which biomass will be useful as a mid-merit power source in the non-fossil future, providing balancing and ancillary services to a complex grid also comprised of wind, solar, nuclear, hydro, demand response and power storage.
Hoover, thank you for the documents. We will look into them carefully.
I wouldn't group all biological materials together as a single biomass, and I wouldn't pick technology losers any more than it is smart to pick technolgy winners before research and development are done on a scale as significant as the problem we face.
The fact of peak oil is important, and I think energy independence is a noble goal that can be reached in time that peak oil will not provide an economic problem for the United States. However, meeting this goal can’t be done by disregarding technologies that some say will never be viable. We also can’t rely on technology alone and must change our view toward energy conservation and support viable technologies toward that end.
I have started a list of energy sources that I have heard “will never work” because they can’t produce even 1% of our energy needs, can’t be stretched any further or can’t be used cleanly. Note that I think “biomass” shouldn’t be in one group as there are many subcategories that are useful and provide byproducts that are starting materials for others.
1. wind
2. waves
3. tides
4. ocean thermal
5. photovoltaic solar
6. solar collectors
7. passive solar
8. geothermal
9. large-scale hydroelectric
10. small-scale hydroelectric
11. algoil
12. biomass – suitable for burning
13. biodiesel
14. biogas
15. bioethanol
16. biomethanol
17. biohydrogen
18. coal gasification
19. Fischer–Tropsch Synthesis gases
20. geobatteries
21. waste digestion
22. shale oil
23. tar sands
24. natural gas
25. natural gas hydrate
26. nuclear
In my view, the least of these could quite realistically be brought to the >1 or 2% level within the near term. Others are already there, save the need to clean them up, which could also be done in the near term. A well-stocked energy tool box will need a variety of alternatives.
Now, a list of things that I have read can’t be done practically to significantly reduce consumption.
1. waste less
2. increase mpg
3. fast lane priority and other perks pegged to fuel efficiency
4. telecommuting
5. Diesel in place of Otto
6. Diesel hybrids
7. ceramic engines
8. Stirling cycle engines
9. microturbines
10. fuel cells utilizing biogenerated fuels
11. mass transit
12. rail transit
13. bicycle paths
14. localized electricity generation
15. ease local restrictions on solar and wind
16. tax incentives related to entropy – Entropy Resulting From Use (ERFU)
17. smart electric distribution grids
18. eat less
19. local farmers markets
20. shade trees
21. green building design
22. cradle to cradle design
23. smaller homes
24. waste heat exchangers for preheating air and water
25. vines juxtaposed to exterior building walls
26. roof gardens
27. grass and paver drives and parking lots
28. wall insulation
29. window insulation
30. natural ventilation
31. smart glass to manage light transmission
32. energy efficient appliances
33. smart building energy management
34. LED and other high efficiency lighting
35. off-peak power storage
36. superconductors
Again here, I think that they can all be done and could save 20 to 30% of our current energy use. Some, like vines, would be modest contributors for a relatively small percentage of homes; but others, like Diesel hybrids, could save 50+% when compared to current Otto engine applications. Why even mention Diesels when they will burn foreign oil? The fact is, they won’t the other fuels identified in the first list can fully replace liquid petroleum from the ground. Moreover, the move to some of the other technologies and energy sources will greatly reduce demand for conventional Otto and Diesel engines.
The points that I am trying to make are that: we need a wide variety of energy sources, including a large number that contribute only 1 or 2% of our energy needs; and at the same time we need to rethink the way we use energy. We can easily become energy independent within ten years or so by a pursuit of technologies that are currently available and can be improved with research and development.
I wholeheartedly agree!
Minor quibble, though, unless you are dead you can't possibly be energy independent >;^)
Right. A point I failed to consider fully.
But, now that you mention it, death is not without entropy consequences. The question remains whether that is an energy penalty that must be considered for a true EROEI value or if some formula might be developed that offsets entropy costs based on death (ECBOD) against the entropy costs of continued life (ECOCL).
I think that, maybe, I should have been more clear that I was discussing energy independence for the United States as a whole. I might have also pointed out that I was not trying to be numerically precise.
I thought, however, that the last point should be clear to most because this discussion topic is in reality all theory and philosophy based on numbers having no meaning outside of our personal views of the current economic paradigm.
Actually that raises an even more interesting question. I don't have a permalink handy but a while back WHT, of fat tail fame, had written a comment in which he accidentally coined a phrase, "entrophic decay" an amalgam of "eutrophic decay" and "entropic decay," at the time we laughed about it but figured there might actually be a valid use for this newly minted phrase.
We discussed that a good use of "entrophic decay" might be to describe how a previously complex organized system had now been simplified and broken down into its basic constituent building blocks and would therefore be available for a new system to organize it into something different.
We even speculated that WHT could calculate the fat tail distribution of the "entrophic decay" of a system such as an entire civilization and that the delta "entrophic decay" of such a system could be accurately graphed and that it might be able to give us some insight as to where in the progress of a system from complex and ordered to simple and disordered we find ourselves.
So being able to study the "entrophic decay" of our moribund civilization might prove interesting indeed. Though the real question would be what kind of detrivores might aid in the decomposition of a society and if it has already decayed enough to be considered collapsed or dead, what kind of reorganization if any can be created from what is left?
I am reminded at some level (very deep pun intended) of Chapter 11: Prelude . . . Ant Fugue, The Mind's I by Douglas R. Hofstadter and Daniel C. Dennett
http://themindi.blogspot.com/2007/02/chapter-11-prelude-ant-fugue.html
I'm working it. I think categorizing certain types of energy sources as highly entropic, such as wind, or entropically disordered such as cheap PV is the fist step. Whether these death-spiral into entrophic decay remains to be seen, but I can see the possibility -- as you can only extract energy from disorder for so long.
Excellent article. No matter what else, the large agro-industrial lobbies need to be hammered continuously with this fact:
That's 0.1% net potential low-grade thermal energy. For comparison, a solar thermal installation can readily achieve 15% (tower/trough) to 28% (stirling dish) of incoming solar energy converted into electricity. With comparatively little water use, simple basic materials inputs paid back in energy in about 3 months operation, no depleting nutrient inputs such as phosphates, and no competition for prime food-growing land.
Why on earth is anyone still even talking large-scale biomass in ANY form?
Bimass is attractive to govt and planners in the near term because it is less cost prohibitive up front, and because it solves the storage problem inherent in using solar generation diorectly.
But speaking as a farmer professionally educated,I agree with going the direct solar route as fast as the plants can be built.
We can learn to live with the costs up front,and the intermittency problems.
We might not survive the effects on the environment of allowing the biofuels industries achieve steamroller size.
A modestly sized biofuels industry capable of supplying enough liquid fuel for emergency transportation and agriculture is one thing;collecting the so called crop wastes and sawing down the forests of the world is another altogether.
It's really very ironic that you should say that Lengould, since the main thesis of the authors is that solar thermal for electricity generation won't work (or wind or wave or tidal or any other renewables) on any scale.
They realised they could prove this thesis (within the normal weak boundaries of the definition of "prove" that apply to academia), if they could show that biomass won't work. This is because power grids simply can't be kept stable in any economically feasible way without a certain proportion of predictable, flexible and part loadable plant - namely thermal combustion plant - and that there are no candidates for thermal combustion fuels other than biomass in the post fossil world .
So, if you agree with them that biomass is a waste of time, you are really also agreeing that solar thermal for large scale power generation is a waste of time.
I suggest if you want to push forward your agenda of solar thermal power production, you start to be a bit less dismissive of biomass since the two are complimentary and will (at least in hot sunny places) require each other to be successful for their own success (biomass alone, as you point out won't be able to provide all the energy required, and solar thermal won't have a grid to generate into without help from biomass to keep it stable).
Hoover,
I wouldn't grant them the premise that biomass is necessary to a stable grid.
I agree that biomass is the obvious, and workable, candidate for the job of providing backup for seasonal lulls in wind & solar production. OTOH, it's not necessary.
You could simply expand some of the other solutions to intermittency: overbuilding; site selection to reduce covariance and maximiz negative output correlation; production of ammonia or other synthetic hydrocarbons with surplus electricity; compressed air storage; pumped storage; nuclear; overbuilt geothermal; etc, etc, etc.
There are a number of workable solutions to intermittency. Some are cheaper than others, some combinations are more optimal than others, but there are wide variety of ways to skin this cat.
I think what you're saying is that we agree on the main point, which is that since biomass will be available for use as balancing plant, we likely will use it.
As to whether it is possible to run a grid without any thermal combustion plant at all, I can easily imagine such a system. It involves lots of flexibly running fast breeder nuclear reactors, and quite a lot of power storage systems and has a mighty reinforced transmission system.
Even if we take away the nuclear and just have solar and wind I can still imagine it. In this system vast supercooled superconductors (each shadowed by two or three redundant lines for back up) transport almost limitless amounts of power across the continents and oceans so that where it is daytime can transport its solar energy to where it is night and where it is windy to where it is still. Huge battery packs, each the size of the pentagon, several in every city, create local storage and provide balancing services and local minute to minute adjustments to ensure a stable frequency.
Technically, I can imagine it, but practically, I don't know how society could ever pay for it.
Yes, we agree on the usefulness of biomass as a backup to the grid.
OTOH, if aliens were to steal all of our surplus biomass, we wouldn't need to overbuild an enormous system of superconducting long-distance transmission lines, nor would we need to overbuild central utility battery storage. There are far, far cheaper choices.
Variance isn't the same thing as unpredictability/unreliability. Wind output can be predicted to a large degree, which allows planning, and reduces or eliminates a need for spinning reserves.
As we add multiple windfarms, presumably with output either non-correlated or only partly correlated, the ratio of variance to mean output falls sharply. Also, many windfarms are negatively correlated, so that careful site selection reduces system variance.
Geographic balancing between parts of the grid only requires transmitting balancing amounts, not the whole load. A cost optimized grid will not have world-girdling, massive transmission lines.
Only a small % of a region's wind power would need to be transferred between regions in order to provide balancing, and possibly not as far as one might think. Sometimes it's just a matter of a number of sub-regions getting their power, on average, 100 miles from their west, rather than 100 miles from their east, and in effect you've transferred power from the western edge of the overall region to the eastern.
We really don't need much more peak capacity - perhaps none at all for many years, with good time-of-day pricing and DSM. That renders most of this argument moot, at least as a boundary: we can use existing generation if we have to as a backup.
Wind farm peak capacity credits are a little like getting a dog to talk: the interesting thing isn't how well the dog talks, but that it talks at all. The fact that even a small cluster of wind farms can have a 1/3 of average capacity credit, or wind at a regional level have an average 55% credit, is important.
I see local wind capacity credit as solving roughly 40% of the diurnal intermittency problem; long-distance transmission solving about 30%, and DSM solving the rest. DSM alone could make an enormous contribution: think 220M EV's (with 2.2TW peak demand or output) doing a dance of load balancing with the grid. This doesn't even touch the legacy peak capacity which could provide backup - this we'd want to minimize to minimize CO2 emissions, but that wouldn't be hard with DSM as a short-term factor: we'd only need it for very unusual, long-term lulls.
Biomass would be enormously useful for grid stability. Biomass is the obvious, and workable, candidate for the job of providing backup for seasonal lulls in wind & solar production. OTOH, it's not necessary.
Solutions for seasonal lulls in renewable production include overbuilding; production of ammonia or other synthetic hydrocarbons with surplus electricity; compressed air storage; pumped storage; nuclear; overbuilt geothermal; etc, etc, etc.
One important utility/grid management tool that has been neglected is overbuilding.
The current grid is overbuilt*: the US has about 1,100 GW of capacity, for an average demand of about 450GW.
Obviously, it's cheaper to overbuild generation that has a low capital cost, like natural gas, but some modest overbuilding can make sense. France has done it with nuclear, and a grid with no fossil fuels would be likely to do the same with wind and solar. Unlike nuclear or solar, wind power can be turned down quite easily, so it could load follow. Just a small % of overbuilding would provide a dispatchable margin using wind power for most of the time.
On the other hand, the surplus power from an overbuilt wind and solar resource could go into various forms of storage: pumped storage, CAES (air), EV batteries, ammonia, hydrogen, synthetic liquid fuels: the list is pretty long.
The combination of an over-built wind resource and Demand Side Management using electric vehicles might eliminate the need for most other forms of generation for load following. The primary need for other forms of generation would be for supply diversity and seasonal variation. The combination of stored power (discussed in the previous paragraph) with biomass would provide "filler" for the occasional seasonal problem.
*Technically, one can't say a grid is overbuilt unless its capacity is substantially greater than its peak demand - I'm using the word slightly unconventionally. the US grid is not substantially over-built from a traditional perspective. I don't mean to say it is.
Nevertheless, there is much more capacity than is needed to handle the average load. There are costs associated with that, and we accept those costs as reasonable to deal with demand variation. I'm suggesting that a similar strategy to deal with the production variation associated with wind and solar (aka intermittency) is also perfectly reasonable. In moderation, and in combination with other strategies, it is likely to be very cost-effective.
Would be an interesting thing to model, if somewhat academic.
Would be an interesting thing to model
Yes. One of the reasons I comment on TOD is a hope to redirect analysis like this away from sterile visions of TEOTWAWKI.
if somewhat academic
Sometimes very theoretical things make a difference. For instance, it really seems to make a difference if one sees the universe ending with a whimper, in a heat death. We're modeling a 100% renewable grid that is very far off, but it seems to make a difference to people.
Why look at a scenario of a grid without surplus biomass?
To clarify that a low/zero-FF grid is resilient: it can be built in a number of ways, with a number of energy sources, and it's problems can be solved in a number of ways.
Hello all,
I like this discussion, and the historical approach of the article. But from my experience with gardening, and as irrigation engineer I could say that the authors make at least one big mistake that I would like to clarify here: it is on their assumption that the nutrient cycle and carbon cycle are following a more or less parallel path in the life cycle of biofuels.
They say that the historical limit of producing biomass was an eventual lack of one or more nutrients after structurally taking biomass out of the system. However, if you take from an ecosystem just plain tree trunks, or other material containing just cellulose and lignine (straw, seed hulls) it is the carbon you take, nutrients are normally locally recycled. To make an ecosystem more productive in terms of carbon, I wouldn't say that the so-called green revolution has an historic victory to claim. Basically, it turned into a greater harvest of monocultures per hectare while wasting a lot of energy, water and top soil. Production in terms of carbon will be a lot more if you try to combine plants with different root depths, different leaf cover, different seasonal activity, etc. etc, like in a natural ecosystem in fact.
French agroforestry researchers of the INRA institute in France found that for example if you plant a maximum of 60 trees per hectare on farm land (in many combinations, in fact very few combinations don't work), competition in sunlight is quite limited, and if you force tree roots do find their water and nutrients in deeper layers than the crops by annually subsoiling or ploughing close to the trees, there isn't a competition at that level either. On the contrary, the tree roots will use drained (irrigation) water and fertilisers, otherwise lost in the cycle. Needless to say that the trees or doing very fine in a density of just 60 trees per ha. For example the researcher found for the wheat/walnut combination (http://www1.montpellier.inra.fr/safe/images/image17.jpg) 30% higher yield than separately cultivated. Can you imagine how many trees you can plant on the enormous (corn, soja, wheat) fields in the USA, Russia, Brazil, etc etc, considered to be already the most productive???
In order to artificially speed up the nutrient cycle, there is a new technique tested out in Canada, France and some African countries, the so called ramial chipped wood (RCW). The ramial wood contain most nutrients, especially just after the tree dropped its leaves. The technique consists out of chipping maintenance wood and spread it on and lightly incoperate it in the topsoil of cropland. It will add lignine-consuming-mushrooms to the soil, who are a real stimulators of soil energy and biodiversity! They buffer water and nutrients, and are base of the food pyramide. The collective garden of our association is covered with 2-3 cm of RCW (the soil "consumes" about 1 cm of RCW per year), and the salads are still looking great after 3 weeks of drought/temperatures above 30 degrees and very limited watering...
See: http://www.rebelfarmer.org/jardin-solidaire-chartreuse.html (in French).
Concluding I would like to say that if the point is to produce biomass, it is better to imitate an ecosystem than than to impose a monoculture. The people in the middleages also had quite productive agroforestry systems. In fact they had cut the forest to cultivate crops, but left for them usefull trees on the land. The fact that Europe was deforested had more to do with wood as construction material (castles, later ship fleets!). It is true that they monocultures they did use (wheats) were 10x less productive than the green revolution ones...hence the great need of land. They also hadn't subsoilers and chippers :-)
Menno
Like Grouch Marx asking for timber to put it in the train's boiler after running out of coal, we have started to look at biomass as a substitute for fossil fuels.
Did I say started? History books say that two thousand years ago, a squirrel could cross the Iberian Peninsula from South to North jumping from tree to tree, without touching the ground. Watch it now...
Easter Island... you've seen the film, no need to explain.
Ireland was also once covered by trees, before loosing half of its population in the nineteenth century (see post in TOD)
In those times we were far fewer, we used much less energy per capita, but we still destroyed the forests while using their biomass.
The Earth is by no means capable of providing, through biomass, a significative part of the energy we use today.
It is entirely possible to get to approximately the same conclusion about biomass energy in a much more straightforward fashion.
A study by Pimentel showed that 1 acre of corn can yield 338 gallons of ethanol (gross). Assume improved technique yields 500 gallons per acre. Ethanol has an energy content of 77K BTU. This give us an assumed gross yield of 3.85 x 10E7 BTU/acre.
In 2008, the USA imported 27.56 QBTU of petroleum (EIA figure). To simply replace oil imports with ethanol on a gross energy basis would require 7.16 x 10E8 acres of farmland. We are a little short of that. We only have 4 x 10E8 acres of arable land (CIA's World Factbook), and it would be a good idea if we reserved some of that for food production.
Biodiesel at 200 gallons per acre and 120K BTU per gallon is even less able to replace imported oil.
The Bush II administration did a study called "The Billion Ton Harvest" which claimed that an annual harvest of 1.3 billion tons of biomass was a feasible goal. At the 1.7 x 10E7 BTU/ton of biomass which they used as an average figure, this would give us ~22 QBTU of gross energy in the raw biomass. Assume a 30% net energy when this is processed into useful fuel, and we would have ~6.6 QBTU net.
Our total energy consumption runs about 100 QBTU per year. The net energy in the 1.3 billion ton harvest - which in my humble opinion is not sustainable - would give us something like 6.6% of that total. To those who would prefer to ignore net energy and talk only about gross energy, I say that makes about as much sense as a business plan that ignores net profit.
Same conclusion; shorter path. Not as much interesting material about historical events and, sorry, it still has some math. let me know if I've managed to drop a decimal place somewhere.
Today I saw a Ted talk about among other things, fast work by mycelia
http://www.ted.com/talks/paul_stamets_on_6_ways_mushrooms_can_save_the_w...
Also, some preliminary figures (imbedded in a paper on another focus) on the fabled productivity of Amish farms
http://acwi.gov/monitoring/conference/2002/Papers-Alphabetical%20by%20Fi...
Here's a resource I just found which I will be looking at.
The Alternative Farming Systems Information Center (AFSIC)
http://afsic.nal.usda.gov/nal_display/index.php?info_center=2&tax_level=1
Phosphate is available in human waste. We seem to have a lot of that being put to poor use, although I expect to see improvements in the phosphate retention of various technologies.
(I see a modern day Dustin Hoffman trapped at a cocktail party. A friend of his father leans in and whispers "crap" to Dustin)
Plus, there is a lot of energy either discarded or gone to landfills. Much is sold overseas via recycling centers. Worth considering in transitional phases of readjustments.
http://www.vancouversun.com/news/Making+something+almost+nothing/3230628...
What does it take to produce 13 terawatts of carbon free energy- the amount that has to be generated if we are going to keep atmospheric CO2 at below 450ppm in the next 25 years?
2 TW of photovoltaic (15% efficient)-100 square meters every second next 25 years.
2 TW solar thermal (30% efficient)- 50sq meters highly reflective mirrors every second
2TW bio fuels- 4 Olympic size pools of genetically engineered algae every second
2TW wind-a 300 ft turbine every 5 minutes
2 TW geothermal- 3 100 MW turbines every day
3TW nuclear- 3 reactor 3 gigawatt plant every week,
Surface area for all that- about the size of America.
Source: Steve Brands- Whole Earth Discipline- quoting Saul Griffiths
I think this is the most persuasive case that alternatives are in fact the "false fire brigade".
We don't have to produce 13TW of carbon free energy, just replace work performed by 13TW of FF, about 4TW.
By your logic we could never manufacture 60million motor vehicles per year, or build millions of homes or hundreds of millions of mobile phones......
Just one example the world economy produces 1.2Billion tonnes of steel/year, enough to build 12,000GW of wind capacity per year(100tones/MW) which would produce 4TW average electrical power, sufficient to replace >80% of FF used for transport, heating and electrical generation. WHAT COULD BE BUILT IN ONE YEAR.
Google says, 'No results found for "corn-powered harley".'
(edit): We do have a charcoal-powered motorcycle, though.
http://www.youtube.com/watch?v=t93QlgBu4Is
Question about Water Requirements for Algal Biomass
Nate, I imagine that closed and open system's for growing Algae require large amounts of water. What would the impact be on fresh water use in a place like the United States? I've thought of looking at rice agriculture as a possible comparison. It seems like competition between food agriculture and large scale Algae agriculture would be a problem. What are your thoughts?
My thoughts are that water will be as or more a limiting input as energy. Here is a paper we wrote on the topic -Energy Return on Water Invested.
As to algae, I am not up to date on the specific water requirements - perhaps others are.
The main issue with producing biofuels from algae is the scalability of the entire process.
A SHORT COURSE ON SYNTHETIC GENOMICS [7.30.09]
The Edge Master Class 2009
George Church & J. Craig Venter
http://www.edge.org/3rd_culture/church_venter09/church_venter09_index.html
So even if we can double current extraction of biomass, this only represents approximately 6% of total U.S. primary energy consumption,
True but this is not very relevant because solar, nuclear and wind energy are going to provide most of FF replacement.
and will never be enough to serve as a meaningful stabilizer in future energy systems.
Not true, small amounts of biomass can make a significant contribution, for example PHEV, using mainly electricity but biofuels could be critical in range extension even if only providing 6% of VMT.
I think that is true as long as by stabilizer we want the end result to be not too far from BAU. If we are willing to accept a lot of demand management, including only running energy intensive industry when the power is available -i.e. we go far beyond the easy demand management low hanging fruit, we can get by with it. But, don't try to sell that to the currently spoiled populations of the developed world.
My assumption (and I've done the back-of-the-envelope math to check it) is that it would be feasible for the US to develop a 25 quad renewables-only energy economy, with biomass energy of any and all types supplying about one-third of the total at most. That is about all we can do without driving the citizenry to starvation. Even at that, they are going to have to develop a much greater appreciation for the culinary possibilities of legumes. The rest would come from a mix of other renewables, with wind alone supplying maybe around another third and all other renewable sources making up the balance.
I don't know if we can do better than that or not. I am pretty confident that we could at least achieve this much within a reasonable time frame (at least several decades up to the better part of a century, or so). I am also pretty confident that a 25 quad economy would be able to maintain sufficient minimal industrial infrastructure to sustain the renewable energy systems on an ongoing basis. I'm not so confident that anything lower than that could do so. Nor am I confident that a renewables-only econony with no biomass at all in the mix could do so; we actually will still need to burn a little bit of something.
Twenty-five quads is about a fourth of what we are using now. We could probably get down to about 50 quads (i.e., cut our energy usage in half) if we got really serious about energy efficiency and put in a sustained effort to drive it down over the course of a couple of decades. Cutting out that last 25 quads is going to be a lot more difficult and a lot more painful, for at that point you are cutting meat and bone rather than fat. Nevertheless, if we've got a few decades in which to do it then it could be done.
This is just a very broad-brush plan. As they say, the devil is in the details, but I don't have the time or the inclination to get into the details to that extent. I don't know if something along the lines above is something we can do or will do. I don't think it is impossible. I DO think that a 100 quad all-renewables economy probably will end up proving to be impossible. I also doubt that it is really necessary to go there, for we can reduce our needs if we really work on it.
WNC,
To replace 100QUADS of FF used in US would probably only need 30QUADS of electrical energy assuming BAU( ie some improvements in efficiency, driving 200million EV/PHEV rather than ICE vehicles, more train long distance travel, less road and a lot less air travel). Just replacing coal(24QUADS) and 80% of oil(32QUADS ) will require 16QUADS of electricity.
Your statement:
Wind is presently a half a quad, but that is ramping up pretty quick. Are four doublings out of the question before we level off at our 25 quad target, over a course of maybe 50-75 years? That doesn't sound too extreme to me, yet that would bring us up to 8 quads from wind. Challenging, but possible IMHO.
seems very pessimistic, considering the US has gone from 16GW capacity in 2007 to 36GW in 2010, and 300GW capacity is in planning stages waiting for grid priority access. At a 30% per year increase we should see 8QUADS of wind( approx 250GW av)in less than 12 years(4 doubling). I see no reason for growth to stop in just another 12 years, even if only 10% growth from 2022, could have 32 QUAD of wind by 2036.
Yes, 8 quads for wind may seem pessimistic. However, I was really reluctant to craft a scenario where any one renewable resource supplied more than a third of the total. More than that seemed like too many eggs in one basket, especially for something as intermittent as wind. Besides, many of the best sites also have scenic value. While I don't think we want to see civilization go totally down the tubes just to save a view, I do think it is something we are going to be very conflicted about for a long time. Best to not count on TOO much from wind, at least for the time being.
The point of my scenario was not to set a ceiling, but rather a floor. There seems to be an argument out there to the effect that reaching 100 quads with renewables is impossible, therefore either a) forget renewables, they're hopeless; or b) we're all going to die! What I am trying to show is that 25 quads is clearly a feasible and achievable target, both on the supply and demand side. There might be scenarios that end up higher than that, in which case great, but if we end up worse than that, it won't be because 25 quads was impossible, but simply because we failed to even minimally get our act together.
A way that I see to boost biomass energy is to 'supercharge' it with hydrogen. For example via intermediate steps Sabatier methane amounts to C + 2H2 = CH4 a product which is easily purified and can be used in anything that runs on natural gas. The combustion products are recycled within the biosphere. Highly fungible methane has double the energy density of carbon (charcoal) for just a 33% increase in molecular weight based on (12+4)/12.
If the carbon is extracted and other plant wastes discarded onsite there should be no need to bring in new phosphorus, just hydrogen. The economics will depend on the cost of hydrogen and the capex of processing plant. Perhaps DoE could fund some research along these lines.
Interesting article, and well argued, but sometimes over-pessimistic.
For instance, the EIA/USDA annual target of 2.6% of US forest biomass is not at all challenging. Southern loblolly pine plantations can be harvested at 25 years, so with an even spread of plantation ages, the average age = 12.5 years. Let's simplistically assume a linear biomass growth rate = X tonnes/year = maximum sustainable harvest.
Then the standing biomass is 12.5X (Not 25X!!) and the ratio maximum sustainable harvest : standing biomass = X/12.5X = 8%.
Of course, further north in the USA, forestry harvest intervals are longer than 25 years. But even in Scotland we average about 50 years, giving maximum sustainable harvest = 4% of standing biomass.
I am not sure I fully understand your logic. If a tree takes 25 years to grow, you can't take it out every 12.5 years. We are talking about sustainably extractable biomass, and that cannot be cut in half. So with a growing time of 25 years, it's 4%, with 50 years 2%. And that's only if nothing gets damaged, or lost, or destroyed, etc.
And unfortunately (actually luckily, for biodiversity), not all forests are monocultures of pine.
The biomass has to be averaged across 25-year-old stands, 24-year-old stands...all the way down to 1-year-old stands. Obviously there's negligible biomass in a 1-year-old stand! It's true that growth rates of a tree stand are not linear, more like an S-curve, but average standing biomass is nonetheless approximately half what it would be if all the stands were 25 years old.
As a Swiss resident, you are probably misled by your familiarity with the forests of Central Europe. For many years, felling there has been way below maximum sustainable cut, so the forests are tending towards a mature (some would say over-mature) age profile. As a result, standing biomass is far above the level it would be if managed for maximum sustainable yield: standing timber volume in Switzerland and Austria averages 300 cubic metres/hectare.
But in Sweden - where the forests are managed more for industry than for tourists - the standing average is 133 cubic metres/hectare, and the annual harvest is about 2.6% of this, as far as I can make out from the Swedish Statistical Yearbook of Forestry (downloadable from www.skogsstyrelsen.se/statistics). Surprisingly high, considering the long hostile Swedish winter doesn't favour high growth rates.
Swiss forestry is based on continuous cover - der Dauerwald - so the concept of harvest cycles may be alien to you. But, like it or not, the clear-fell/regrow harvest cycle is what produces most of the world's timber.
Forests are rarely damaged or destroyed in such a way that no biomass can be salvaged. In Scotland our main risk is windblow: on average 15% of our trees get blown over before harvesting. The trees can still be salvaged, but are difficult and expensive to extract from a tangled mass, so the owner gets a disappointing financial return. But the power station still gets its biomass delivered.
And yes, not all forests are monocultures of pine. Mine are mostly spruce, because that grows quicker. Eucalyptus grows quicker still: if a Brazilian eucalyptus plantation took as long as 25 years to reach maturity, the manager would be sacked for gross incompetence.
One quick comment (in DC, just finished preparing for a meeting tomorrow).
Hannes Kunz quickly discounts bio-sources of methane, without considering the efficiency increase that methane has (with existing technology) over solids that burn.
Methane can be stored and then burnt in combined cycle natural gas power plants (GE H series is 60% efficient, all energy output is electricity) and in CHP power plants (electricity plus heat output) (best Danish 95% efficient).
So creating bio-methane for high value bridging of gaps in other renewables & nukes is a more viable option than his analysis presents because of the conversion efficiency gains possible.
Alan
Methane (the key component in natural gas) has indeed a relatively good efficiency in CHP plants (provided someone needs the heat).
Unfortunately, on the way to methane (or biogas), biomass needs to be fermented, which happens at around 50-60% efficiency (net available energy content that gets converted from the original biomass), which reduces overall efficiency significantly, even below the direct conversion of (cellulosic) biomass to electricity via burning.
I'm suggesting a thermochemical pathway to methane that doesn't involve fermentation
water splitting 2H2O = O2 + 2H2
pure oxy burning C + O2 = CO2
Sabatier CO2 + 4H2 = CH4 + 2H2O
Combining these reactions we end up with an oxygen surplus. The real world net energy will depend on heat transfer and efficient catalysts, but mainly the cost of water splitting which might have to be done with nuclear energy. The payoff is the fact that several forms of methane can be blended, there is an existing gas distribution network and there are already 11 million NGVs.
US paper consumption is about 100 million tons per year. Paper has an energy content of about 7500 BTU/lb. So the paper used in the US contains about 1.5 Quads/year.
To make a pound of paper requires about 3 pounds of forest product. However, at least some of the 2 pounds that does not wind up as paper is burned for cogeneration to power the papermaking process, which is very energy intensive.
If we eliminate 50% of paper use, (e.g. newspapers, magazines, books, junk mail, catalogs, and non-essential packaging) we can burn the 2.25 Quads worth of forest products to get biomass energy. Plus, we probably save about another 0.75 in the reduced industrial energy demand for papermaking.
The result is that a 50% reduction in paper consumption would result in about a 3% combination of reduced demand and increased energy availablility for the United States.
Most paper used in the US is produced on a relatively small acreage in the southeastern US.
I have been talking to a person work has done some work making cellulosic ethanol from Georgia pine trees. He says that quite a bit of paper production has now been shifted overseas--to SE Asia, so we now have excess wood in the South East that can be used for cellulosic ethanol. My guess is that the imported paper is not at all sustainably harvested--perhaps may even be clear cut original growth. Even if this gives a bit bigger supply of ethanol, I am not sure it is good for the world.
By the way Hannes, thanks for a very nice article!
Hi Gail.
A lot of paper production is now taking place in Asia, mainly because it is then used as packaging and exported back to the US with goods inside. There is not much actual paper being shipped back your way, although there is some from South America.
However, paper is not made from trees, paper is made from pulp and pulp is made from trees. Pulp is a globally traded commodity, the US is an exporter as are Japan, Brazil, Chile, Uruguay, Sweden, Norway, Finland and South Africa.
Please don't worry about the Amazon, whatever drivers there are to destroy that, they have nothing to do with pulp production which takes place mainly in south of the country. The yields on Brazilian forest plantations are spectacular and can reach up to 45 m3 per ha per year, well over double what can be achieved in the US.
Environmental criteria for forest plantations in Brazil are recognised as some of the best in the world. Plantation owners are forced to buy, preserve and protect (and in some cases even improve) areas of natural vegetation on a similar area of magnitude to the plantation. Almost all of these plantations are FSC accredited. Chilean and South African plantations are also almost all FSC accredited.
Pulp companies are businesses with shareholders and stock prices. How could you think that they would just destroy the resource that they rely upon? What would they do afterwards? Haul radiuses are only about 50 miles, it wouldn’t take them long to chew through that if it wasn’t being replaced.
In addition to the pulp trade, there is also quite a lot of wood chip trade, where chips are shipped in special high cubic panamax class wood chip carriers to make pulp. Japan is the main importer here, as they have built a lot of port based pulp mills, China is starting to as well. Chile, Australia, New Zealand and South Africa are large exporters. Almost all of it is FSC accredited.
Within Asia itself, Indonesia and Vietnam have the biggest forest plantation areas, and Indonesia has built its own pulp mills. In Vietnam the forest plantations are a part of a 3 million hectare reforestation programme of which about 1 million is trying to re-establish natural forest. To do that you have to have money to protect them and jobs for the locals so they don't just chop down a tree as soon as they see it. The forest plantations provide both (revenues and jobs). I don't have any specific knowledge on the Indonesian plantations but as I understand it, they are FSC or PEFC accredited.
Just one last point, almost none of the forestry in the US is there for paper or biomass production. It is there to make timber, the most sustainable building material in the world. Pulpwood and biomass are co-products that are produced firstly by the forest thinning (which is necessary to get the remaining trees to grow big enough) and then by the thinner top ends of the trees (and any weedy trees which didn't grow fat enough) during a clear cut. There are very few pulp (let alone biomass) orientated timberlands in the US. The recent massive bust in housing starts and recent pulp mill closures are devastating for these landowners, most of whom are small family affairs.
Another excellent big-picture overview, Hannes. You and your colleagues are making a significant contribution to understanding the real breadth and depth of our energy crisis.
To those who feel moved to respond with anger and derision: Please, try to step away from your attachments to particular renewables, and especially from your certainty. Try to look at the "game" from a vantage point high above the playing field. Forget your favorite learned strategies, just for a moment. Imagine that we might not win. Pretend it's our first game, ever, and that we need to learn the rules, and the shape of the field, before we start.
The Zen masters might call it beginner's mind. Others just call it science.
try to step away from your attachments to particular renewables, and especially from your certainty.
If only the authors of the Original Post had listened to your advice before they wrote with such certainty unrealistic things like this: "even if we can double current extraction of biomass, this only represents approximately 6% of total U.S. primary energy consumption, and will never be enough to serve as a meaningful stabilizer in future energy systems."
I think you have the positions reversed. Kunz & Co.'s case appears wedded to particular renewables used in ways to fit into the existing system. That is not unlike trying to process petroleum into feed suitable for horses, or charging a 1909 Baker Electric with oats. The idea is absurd, and I am not alone when I say that this is, at best, a severe case of inside-the-box thinking.
Anybody knows anything other than biofuels to have the P word be dealt with? Yikes, I'm ripe for this anti-academic culture shocking red towel throw into the nursery.
ICRISAT is one organization working on obtaining biofuel from marginal lands and on rehabilitation of degraded lands by means of biofuels cultivation.
A bit of pain for the oilwo/men now, sorry its the topic. Yurop had taken out of production more farmland at huge expense over the last decades than it uses now for fuel; almost all of it with inefficient first generation technology. It has been given to biofuels to grow and get beyond first generation. Biomethane is by now the first second generation fuel on the go, others are in the demonstration phase/loop. Agronomic potential is with things like increased yields, alternative crops (eg. sweet sorghum using less water) and others, see the hidden equidae. True, long time no go for biochar on TOD, in the meantime a German says he has an operating business plan.
So be biofuels forced unto the compassionate as the curse, I'll take responsibility. Murder one, get me, murder a million, get a pillory for an American Football star, but give the poor a chance to learn how to grow crops. Be the smart one to turn biofuels into a P saver, it can move the skewed humans towards their destiny, but not in this world, and spare me from becoming literate.
Now to get going:
yaya, algae might solve the horse problem, but would require a reformer for the Baker.
Yes, Yurop can, according to believer's high command: http://www.greenpeace.org/international/en/publications/reports/renewabl...
Unsure if the line below is original research, but anyway:
Either the Vulcans will save us in 63, or they'll at least be glad about the warm reception.
Yet another answer to questions not asked is that this was ast message 45.
It's the economy, my friend.
I'm late to the party, I see. 103 comments already! I'll limit myself to a few points that I don't think have been raised.
First though, I'll say that this was a really excellent article! No glib, unsupported generalities, but good quantification and citations. I thank the authors for writing it, and Nate for posting it. It goes into my "keeper" file.
In a way, it's a pity we even have to bother talking about biomass as an energy resource. The 0.1 to 1.0 W/m2 says all we should need to know about it in that role. Even mediocre flat-panel PV will deliver 30 W/m2 in a sunny location.
However, while it's a lousy energy resource, biomass is actually a pretty good carbon resource! Worldwide combustion of fossil fuels releases 5.5 gigatons of carbon per year into the atmosphere, while photosynthesis fixes 60 gigatons per year into biomass. Of course, there's a balancing release of 60 gigatons per year from decay of dead plant material and metabolism by herbivores. Nonetheless, if we could manage to sequester 10% of the annual production of biomass, it would offset our current use of fossil fuels.
We probably can't capture and sequester anywhere near 10% of total biomass production -- certainly not on a worldwide basis. But if we take the biomass that we can harvest and then use hydrogen and thermal energy from more efficient resources to convert it, we can probably make enough carbon-neutral liquid fuel to keep at least a trimmed down aviation industry alive, and to fuel off-road heavy equipment.
One note that I don't think anyone has mentioned rearding sustainability: one of the nice features of switch grass is that it's perrenial, with a deep root system. When it's harvested in winter, its sap and most micronutrients have descended into the roots. The dry stems that are harvested are almost entirely cellulose. They carry very little of the micronutrients that the plant needs when it's growing, so make-up fertilizer needs are minimal.
Yes, thank you for doing all this work! I appreciate how much effort it takes to put something like this together and I'll be sure to cite it in my work so that it continues to provide value to others (many a good article becomes invisible once it's below the fold of the first page here).
BTW, though I'm interested in hearing what you learn about the supercritical plants mentioned above, I am fairly certain it won't change the conclusions presented here.
That would be a very mediocre PV panel. I install panels that get ~141 W/m2 max power. (Even on an overcast day they'll do 60 W/m2 or better.) And they are not the top of the line brand for efficiency.
The author has presented a good overview of the problem, although I could wish his tables and graphs didn't come across with 4 point Squint type.
Partial solutions:
1. Algae pretty much have to be done in closed environments. If you select an alga to divert energy to oil production it doesn't reproduce as fast. Any foreign alga that comes in will out strip the local one with time.
I suppose you could kill off and reseed if set up in an environment where foreign alga were uncommon -- e.g. dessert.
I suspect that alga get moved around a lot by birds.
2. Water: It will likely require a semi-open system. One in which water is flushed through, but organisms are kept out. E.g. Consider a desert by the sea. Pump filtered sea water into the alga tank to replace evaporation, but also dump some concentrated tank water back to the ocean. If for every liter of evaporation you pump 5 liters in, and 4 liters back to the sea, the saline concentration would increase by about 20% over normal sea water at steady state.
With clever design the fresh water may be reclaimed as a secondary product.
3. Temperature control:
A closed system has significant temperature issues. Any sunlight that comes in has to be either reflected, or used to power the alga chemistry, or it heats up the water. There is a good reason that greenhouses typically have an air exchange every 90 seconds, and even at that, are unusable in summer in warm climates. Indeed, a high insolation environment may able to produce more energy through solar thermal than through alga biomass.
Phosphorus:
In any biomass production scheme the phosphorus is not going to disappear. The P that goes off with the vegetation is not going to appear in the biofuel. Since fermentation is a first step in most schemes, the sludge can be returned to biomass plantations. This sludge would contain most of the P. If burned directly, P becomes P2O5 in the ash.
Storage is the key. It's not clear whether eeStor has a product or it's all snakeoil, but the product is plausible. If throwing a billion or two in research would bring this product to market, it solves a lot of problems.
In a nutshell eeStore claims a prototype of a box that can store aboaut 50 kwhr of energy, can cycle at least a half million times, has a cycle conversion efficiency of better than 90%, and costs between 2 and 3 grand to produce. The box is about the size of a bar fridge. Charge/discharge rates are limited by the ancillary electronics and wire gauge.
50 kwhr will run a non-electrically heated/cooled house for a couple of days. Installed in large banks, at wind farms, this technology can be used for load levelling. Installed with smart metering in residences, households could draw power at off peak times. This also makes off grid construction far more feasible.
So for example: Suppose you wanted 4 day load leveling capacity for a wind farm: Typical a wind farm delivers 30% of rated capacity. So a 3.3 MW turbine on the average delivers 1 Mw. In a day that is 24000 kwhr. So for 4 day capacity you would need 100,000 kwhr storage. This works out to about $4,000,000 if eeStore's figures are on track. This is a cost comparable to the cost of the turbine itself
4 days is probably excessive. 48 hours is enough to bring a coal fired unit from cold to full operation.
High altitude wind is another potential source. This technology requires serious engineering, and may require some breakthroughs in cable design.
The author has yet made no mention of solid carbon fuel cells. Currently in prototype in various labs, SCFC has the potential to double the efficiency of electricity generation from coal, and as a bonus provides a nearly pure CO2 stream for sequesterization. There's still lots of details to be worked out to make it commercial, but it shows at least as much promise as biomass.
The reduce component of energy use is one that also needs to be examined. As a Canadian I admit to that my nation is one of energy hogs. We have one of the largest per capita energy usages due to two factors:
* We are spread out fairly thinly compared to other first world nations, so transportation eats a bigger share.
* Our climate is chilly, so we use more fuel for heat.
Never-the-less we could achieve significant energy savings and very little change in standard of living:
A. Make even more stringent air exchange and insulation requirements for new construction.
B. Encourage multiple unit dwellings.
C. Re-examine our zoning laws and figure out ways to keep work and home closer (on average) together.
D. Work out economic incentives for people to drive less using more vehicles, with the extra vehicles being more specialized. E.g. One person commuter cars with room onlly for a single person and some groceries.
I find the “Fake Fire Brigade” series informative and fascinating; I am grateful to Hannes Kunz, Hannes Kunz and the unnamed others who put it together; and to Nate Hagens for posting it.
Being new to The Oil Drum, it is wiser for me to read more and comment less. But in view of some of the hot kerfuffle in earlier comments, I lay aside all claims to angel wings. I find myself too much in agreement with opposing opinions there. It appears to me that biomass-sourced energy, like much of “alternate sources of energy”, is utterly incapable of supporting either a business-as-usual future or even a severely cut-back version thereof, with all the gingerbread knocked off. But as the essay acknowledges, it certainly can play a role! I suggest that biomass energy be viewed as one of many firefighters for battling a conflagration that threatens to become a holocaust.
Indeed, this can be said of virtually all “alternate sources of energy” (at least those that I am aware of). Like in a little kids’ campfire song, “You Can’t Get to Heaven” (sample verse: “You can’t get to heaven on a pair of skis. You'll shuss right through Saint Peter’s knees” and similarly with a Kleenex box, a miniskirt, etc). I am sure there are many TOD folks know a gazillion more verses. But unlike in this song, we CAN get to sustainability (in lieu of earthly Heaven) by employing all such artifices.
Along with virtuous abstinence from excessive energy consumption, of course. (How do we define “excessive”?
I was musing today that it's likely a contemporary psychotherapist, upon hearing the basic details of society's intent to make transporation fuels from biomass, might suggest such an effort indicated something more serious that a mere neurosis. Perhaps even something between neurosis and insanity.
G
I have to agree again with Alan Drake: the framing of "Fake Fire Brigade" and "Panacea?" sets up a a strawman: nobody that I'm aware of is proposing a society based solely on biomass.
Another related major flaw: the assumption that agricultural inputs would have to come from biomass. In the kind of diverse energy system that any sensible person would plan for, tractors would be primarily powered by electricity (from wind, nuclear, solar, etc, etc), and hydrogen for fertilizer could come from wind-powered electrolysis.
Could biomass provide an important niche supply? Of course. For instance, most land transportation is very likely to go to electricity. An EREV (an EV with a liquid fueled backup generator, like the Chevy Volt), could reduce fuel consumption by 90% compared to conventional vehicles. This means that the 230M vehicles in the US could be replaced by a fleet of EREVs, and that fleet's liquid fuel needs could come from our current 10B gallons of ethanol!
Precisely the same approach will apply to our long-haul trucking and water-borne freight: most trucking would move to rail, and the remaining 20% might get 20% of it's energy from liquid fuel, for a net of 4% of the current truck diesel. Water-borne freight would get 50% of it's energy from wind (kites, already in use) and solar (PV), and most of the rest from batteries - perhaps 10% of it's total energy might come from liquid fuels.
As noted above, biomass is just as efficient as coal for electrical generation. More importantly, only about 5-10% of our electricity would be usefully provided by biomass: the rest would come from wind, nuclear, solar, etc, etc. Minor overbuilding of wind (perhaps 15%) would allow it to be dispatchable; EV's would provide diurnal Demand Side Management (envision 230M EVs able to draw 10KW as needed: that's 2.3TW of demand, available as needed to absorb whatever peaks of wind production might happen - alternatively, think of it as 230GW that could draw for 8-16 hours, as needed).
Finally, Phosphorus limits are greatly exaggerated: For decades, it has been said that the phosphate in Florida could be mined for about another 25 years. Technological advances and market changes, however, have continually lengthened the expected life of phosphate mining, allowing mining of rock that wouldn’t have been mined in previous years.
The Hawthorne Formation, which contains much of the Florida phosphate deposits, covers much of the Atlantic Coastal Plain of the southeastern United States. In the heart of the Central Florida phosphate district, the Bone Valley Formation overlays the Hawthorn Formation. The two are separated by a limestone layer of varying thickness. It is the Bone Valley Formation that has produced the majority of mining activity in central Florida to date. The Hawthorne Formation is being mined in North Florida. It is also the Hawthorne Formation that is being mined in the southern extension of the central Florida phosphate district.
Florida phosphate reserves alone contain about 10 billion tons of soluble phosphate rock. Based on the current mining rate in Florida, this would last more than 300 years.
http://www1.fipr.state.fl.us/PhosphatePrimer/0/D180A63B2294832985256F770...
It appears that the USGS resource is understated, if the footnotes on the 2007 report are accurate:
"Large phosphate resources have been identified on the continental shelves and on seamounts in the Atlantic Ocean and the Pacific Ocean. High phosphate rock prices have renewed interest in exploiting offshore resources of Mexico and Namibia."
Offshore resources would certainly be more expensive, but they'd create a buffer to prevent overshoot - a zone where consumption became more expensive, and pushed us towards recycling.
After all, consumption could go down, with improved farming practices and recycling - roughly half of US ag production goes to livestock - recycling their phosphorus shouldn't be that hard, in the grand scheme of things.
I'd say we have quite a lot of time to set up recycling.
Hi Nick;
It's funny how intensely I've clenched onto this framing question for the series. But I do think it's a real issue in this communication challenge around PO.
I have appreciated much of what they are working on, and as far as the Biofuels article here, the hidden costs and externalities are certainly pieces that we have to be very cautious not to dismiss or overlook.. so I don't at all disagree that Biofuels are frequently poor choices, and particularly for really large scale applications.
But it's like the real target of their article OUGHT to be 'BAU and Cornucopianism' are too high a goal for ANY fuel, but it keeps coming out like 'Watch out for Renewables! They're a waste of money and effort!'
Personally, I've pretty much decided that I'm surrounded in my life by people who may be trying to get to their Green Shangri-La, (whether they acknowledge it as such or not), but which is 10,000 miles North and unreachable, and doesn't even exist, .. but since we NEED to be heading to Bangor, which is just 866miles north and possibly reachable, why get them to stop taking any steps that pushes them north? (And again, I'm not arguing that Biofuels in any significant way will 'Do the job' .. but that there are a handful of Renewables that SHOULD be getting positive, practically Obsessive attention at this point.. but are getting lumped in with known losers. Primarily, I lean towards Glass and Mirrors. PassiveHaus Solar, Hot Water, Troughs, Heliostats. Then PV, etc.. for a big push to get a chunk of such power installed broadly)
Bob
Me three.
Me four. (It was Hannes who admitted to failing to make his point.)
Speaking of which, Hannes never answered my question about British wind data for Figure 5.
Thanks for the pointer.
> assume that the "fire brigade" really fails to deliver.
Who would sell an insurance policy for NPP? But then again, Castel Gandolfo stands on the rim of Tambora, be quiet.
For the wider argument, sorry, can't compute, maybe I'll succeed in working that out tomorrow. Does it mean to say that TOD is a cosy world?
PS: Neither of both, but Mumimax dosis plus overtime. The cogniscient would be able to explain, itou, but like when its shrouded in mystery. And I have the weakness to think that it was warranted. As an added benefit, the linked album contains a good hint at the risks of an e- energy monoculture.
PPS: @WHT, #563518, that mention of ergonomy and human comfort in the same breath was awesome. As for #687004, the solution to how many times the cannon balls fly must be flowing in the net*
the real target of their article OUGHT to be 'BAU and Cornucopianism' are too high a goal for ANY fuel
For any single fuel. As part of a diverse grid, wind, solar and nuclear could certainly provide all of the power we might need.
I suppose that might be possible, but I'm fairly sure that we'll hit a number of other ecological walls due to our numbers and appetite at this point, so I have been expecting that we may be due for anything from a 'settling' to a 'culling' in our population numbers one of these days, and that there will soon be some very different 'usuals' in whatever the future BAU holds..
I think RE might come up to 30% of our current uses, and we would have to be ok with that.
I'm worried about Climate Change, but that won't hit soon. I don't see any other likely ecological walls.
Wind is cheap, easy and fast to build - I just don't see the problem...
Generally, I'm looking at potential Black Swans in the Ocean Fisheries, which are really teetering from what I've heard, or a very 'flukey' year that crushes an inordinate amount of the crop yields, that sort of thing.
Climate Change and other forms of Ecosystem Collapse may be gradual, like boiling the proverbial frog, but might just as likely be quickly tipped (against our purposes anyhow,) by a string of unfortunate events. Like an oil spill in the GOM trashing a broad range of major regional species by knocking out a key species or three.
I'm all for wind and solar, and a hearty and immediate build out of them.. but we are really not ready for a large-scale switch over to such a different way of sourcing power. I think we're vulnerable to a great number of problems during this (necessary) transition.. even nearly unrelated problems that would otherwise be solved with scads of energy, when those scads are no longer quickly available.
I'm all for it, but I don't expect it to be easy or bloodless.
potential Black Swans in the Ocean Fisheries,... or a very 'flukey' year that crushes an inordinate amount of the crop yields
I agree that there are some risks here. OTOH, potential agricultural productivity is far higher than current consumption. Hunger in the world isn't due to limits to agricultural productivity, and that would be the case even if agricultural productivity were to drop by 2/3.
I'm all for it, but I don't expect it to be easy or bloodless.
I agree. That's not really a matter of physics. We humans do manage to shoot ourselves in the foot, don't we?
And we can always use a bigger gun... Big Bang investigators want new atom smasher
http://news.yahoo.com/s/ap/20100726/ap_on_sc/eu_france_atom_smashers
Though I'd much rather invest the 13 billion in pure scientific research as opposed to bombing people back into the stone age in another stupid war.
I'd much rather invest the 13 billion in pure scientific research
Yes. You always learn something...
Sad that no gaucho has been defending his horse yet. Maybe wondering how much carbon dioxide they produce gets them started.
Besides, cherrypicking cos not enough resource to go after it all.
From some reading done a couple years ago I got the numbers for the area dedicated to biofuel crops. Close to 20 million ha for this year's world harvest of 79 Mtoe final energy. It can be argued it contributes to keep the NYMEX in check.
Lost trace of the sources, and too lazy atm to go search. Will do if called wrong.
Referring to the land degradation claims, and even without knowing agronomy, it can be noted nonetheless that some 94% of world biomass energy is not biofuels and almost none of that comes from dedicated agriculture. Only some agricultural byproducts are transformed into energy, straw or waste for instance. There is huge unused potential. Watching biomass in Austria, Sweden or Finland, you sense a braver world than home indeed, and I'm pretty positive that their forests are not shrinking. On the contrary. Also off the cuff, all of these have extensive national parks with woodlands left pristine. And being a bit beasty again, Austria beats Switzerland 16 to 8 on biomass energy share. [1]
As up to now only electricity and biofuels are contenders for moving people in the long run beyond scifi like compressed air from geothermal, I'll need to get behind that phosphorus thingie some time. Strange that it gets mentioned so little, ignorance here certainly makes you want electricity for world domination. And again comes the note that, except for some anaerobic digestion, biomass electricity is not using up phosphorus resources as far as I know. Very little electricity is from biodiesel and zero from ethanol.
[1] As usually, according to the BP energy statics conventions with 50% efficiency for geothermal and solar heat added.
Phosphorus ore is notorious for its radioactive constituents. The risks it poses are most threatening to people who come into direct contact with it - eg. Foresters, organic growers, etc. However, organic growing organizations seem to be oblivious to these health hazards - despite the information having been available for many years.
Where there is uranium in natural rock formations, there will also be all its carcinogenic decay rate products; such as radium, radon, radioactive lead, polonium, thorium, etc.
There are also toxic metal contaminants such as beryllium, manganese, arsenic, lead, mercury, cadmium and vanadium.
Elemental phosphorus plants extract pure phosphorus from ore for use in the chemical industry. There are eight (5 operational, 3 standby) elemental phosphorus plants located in four different states. However, two plants in Idaho are the major emissions concern.
Phosphate ore is high in uranium and its decay products. These decay products, especially polonium-210 and lead-210, become airborne through volatilization during the extraction process and could potentially enter the atmosphere in large concentrations. Exposure comes from inhaling the radionuclides, with the lungs receiving virtually all of the dose, causing an increased risk of lung cancer.
Again, I find that this series of articles misleads by a heap of half-truths.
Yes, it's true that BAU will not go on. Yes, it's true that limited NPP means that biomass cannot possibly replace fossil fuels. Yes, it's true that there are very large losses between biomass and e.g. any drop-in substitute for current motor fuels.
All of these claims mislead by omission. The authors misdirect the reader to e.g. calculate the BTU value of synthetic heating oil made from wood waste and determine how many houses can be heated by Maine's forestry industry. Of course, this is not going to happen. Homes will switch from fuel oil to wood pellets, electric heat pumps or some combination of the two. Instead of pellets, wood may be converted to pyrolysis oil near the site and trucked or even piped to the point of use. Pyrolysis oil can feed gas turbines and re-heat cold air in CAES storage, for 80% fuel-to-electric efficiency even in non-regenerative systems. Between offshore wind, biomass and nuclear, New England's winters can be kept warm.
I addressed the need for a radical re-think of current energy systems almost 4 years ago here on TOD, with Sustainability, Energy Independence and Agriculture. Yes, it's true that most of the well-publicized efforts are aimed at support of BAU. This doesn't matter as long as the important work gets done behind the scenes. Solid-oxide fuel cells running on gasified chicken litter, floating "spar" style wind turbines needing only to be towed into place, anchored and wired, compressed air storing days or weeks of electric power in any cavity which can be blasted, dissolved or dried of water in its pores... these all have a logic of their own and will advance no matter how many innumerate pols are dazzled by visions of a nation of Hummers running on alcohol derived from cornstalks.
That's the thumbnail sketch. I will be back to address specific points tomorrow.
Going into the post in detail and hitting some of the omissions:
There is no argument that the technology of the day was only capable of sustainably supporting some tens of millions of people. It relied on the Net Primary Productivity of the plants, and used large amounts of it inefficiently.
The Industrial Revolution and the advances in the use of coal and then petroleum did change everything. However, Kunz & Co. assume we have stopped there. While most current human energy consumption does come from fossil hydrocarbons, there are very large and almost untapped resources waiting for us. These are nuclear (a large fraction of world electric consumption and held back largely by politics), wind (falling in price and rising 40% or so each year) and solar. These 3 resources are effectively unlimited on the time scale of nations.
This is true. However, the energy inputs for cultivation and even chemical applications are relatively small; the crop residues from maize are more than sufficient to supply all necessary tractive power and nitrogen with the potential for a great deal left over (more recent analysis). There are efforts under way to slash the required inputs with perennial grains and nitrogen-fixing maize and wheat; perennials will also slash the loss of nutrients in runoff. This situation is bound to get better with time, ceteris paribus.
But the big issues are hidden using big concepts described in big numbers.
The error (deceit?) is implied by the comparison between total US NPP and total US energy consumption: using biomass implies meeting all needs using it and nothing else, and no improvements over the status quo. This is a straw man. No advocates of RE (or RE+nuclear, which is my position) take anything close to this position. Some of us have looked at the edges of feasibility using assumptions we know to be pessimistic in some ways and found that impressive things are at least theoretically possible. BAU changes every generation or so (there was no vehicle-class called SUV in 1980), so there is no reason to project the future to be exactly like the present and forecast collapse if it cannot be.
A serious attempt to look at the problems would start with a reasonable estimate of available biomass, such as The Billion-Ton Vision (perhaps 1 billion dry tons available without undue difficulty including solid waste). At 15.8 million BTU per ton, that's about 16 quads of raw energy. Depending on how and where it's processed, it could yield as little as 8 quads (charcoal, processing heat discarded) to almost the full 16 quads (fast pyrolysis, 11 quads storable oil + 5 quads heat byproduct). The winner for storable fraction is torrefaction, with roughly 90% of input energy (14 quads) in the net product.
Proposing to run a 100-quad energy economy on even 14 quads of fuel is a non-starter, but that proposal is a straw man. As Nick noted ages ago, the proposal is to use flows when we can, and use long-term storables to backfill the difference. In the USA the flows are quite high; the potential windpower in Texas, Kansas, Nebraska, S. Dakota and Montana is calculated to be over 20 million GWH per year (adding ND, IA, WY, OK and MN increases this to nearly 31 petawatt-hours per year). 20 million GWH is 68 quadrillion BTU of electricity, roughly 200 quads of fossil-fuel equivalent.
68 quads plus 14 quads isn't even 94 quads, you say. True. However, some things must be kept in mind:
If you take the 100 quads of raw fuel and knock out 20 quads of losses in coal plants and 12 quads of inefficiencies in light-duty ICEVs, the total required is about 68 quads. 68 quads of requirements is less than the 82 quads of energy available, and we haven't even looked at efficiencies in freight (electric rail replacing diesel trucks), space and water heating (heat pumps replacing combustion furnaces), gains from better insulation, solar DHW heating, and a long list of other things. And notice that I didn't mention nuclear yet?
Yes, BAU is in danger; BAU is always under assault by the future. It will die, because change is the only constant. But industrial society and even most of the recent American lifestyle has plenty of energy to feed on.
Returning to the post (unlike some people, I have a day job):
Table 3 is another graphic which raises more questions than it answers:

The penalty number appears to be (1 - (biomass/fossil)) * 100%. If I have this wrong, please feel free to correct me.
The derivation of the efficiencies for biomass is crucial. BTL is probably going to be closer to 45% than 40%, but it's the others which raise my eyebrows. Biomass to nitrate, just 25%? Pyrolysis oil ought to be a pretty easy substitution for natural gas, yielding just a 30% penalty. Torrefied biomass (90% efficiency from biomass) should be an easy substitute for coal in a gasifier, with less sulfur. The 55% number just does not seem realistic.
I'm even more skeptical about the biomass-to-electricity figure. Pyrolysis oil has been burned successfully in gas turbines. If used in lieu of natural gas in a CCGT, it should yield roughly the same 60% output efficiency (plus low-grade heat). Added to the 30% loss in fast pyrolysis, the field-to-wire efficiency should be about 42%—a -5% penalty over coal. Using torrefied biomass as a direct substitute for coal should yield about 36%, a 10% penalty. This simply does not add up.
I can't touch the combined-power figure without some idea of the assumptions. Process heat can be anything from medium-pressure steam to warm air for drying. Call this another very big question mark, calling for publication of the model details.
Last, the serious proposals for RE call for combinations of resources, where appropriate. Take backup electric power when the wind goes down. Direct generation from pyrolysis oil would yield about 42%, but using that same oil to re-heat air in a CAES system could produce 70% oil-production * 80% oil-to-electric = 56% (plus the electric input to store the compressed air). Storage of compression heat could boost that figure considerably, perhaps well above 100%. Assuming 70% recovery with regeneration but no reheat (per General Compression) and 85% turbomachinery efficiency, increasing electric output to 133% of input would require fuel equal to roughly 74% of the electric input for a fuel-to-electric "efficiency" of 179% (overall 76%, probably optimistic but not by too much).
The questionable assumptions in Table 3 are fed into Table 4:
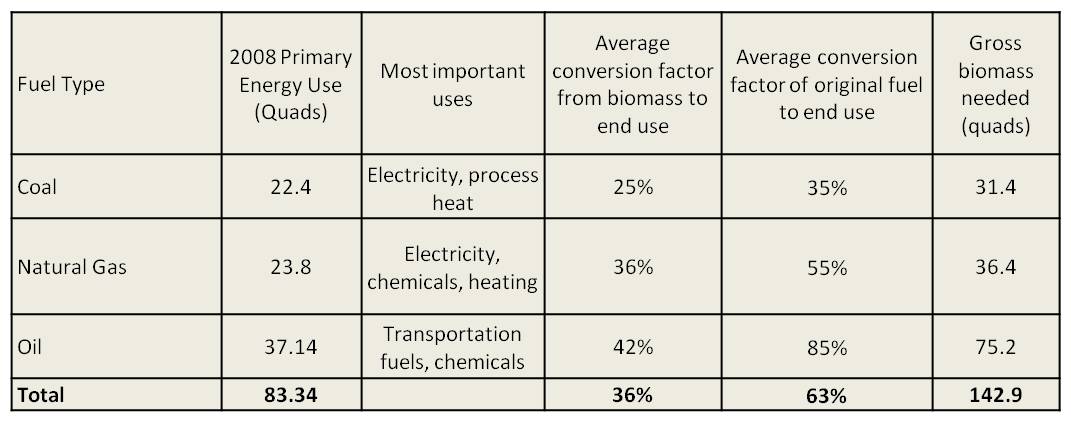
Take electricity as one example. The USA currently consumes about 4 PWH of electricity per year (13.7 quads). Adding an additional 40% to accomodate electrification of all ground-vehicle transport increases this to 5.6 PWH (19.1 quads). If half of this comes directly from wind and the other half comes from CAES with some regeneration and reheat at 150% fuel-to-electric efficiency, the raw fuel input is 6.4 quads. This could be produced using 9.1 quads of biomass converted to pyrolysis oil, or 7.1 quads of biomass converted to torrefied form and gasified on demand. This is considerably less than the ~20 quads of byproduct biomass projected by the Billion-Ton Vision study, and still well within the scope of my much more conservative assumptions above. This figure takes care of all fuel (coal, gas) burned to make electricity plus all petroleum used for motor fuel; displacing fuel for DHW and space heat would add to this, but remember heat pumps.
More troubling are claims everyone should recognize as questionable:
No, we do not. Both Otto and Diesel engines can run mostly or wholly on producer gas, made aboard the vehicle from e.g. wood chips.
Rentech would dispute that (they are starting from coal to make ammonia) and the Stranded Wind Project is attempting to make ammonia electrolytically. Even methane has to be reformed to produce ammonia, and the reformers can start with other things than natural gas.
Table 5 is more questionable still:

Kunz & Co. start with the 3.9:1 EROI without examining it. The fuel required to make a pass over a field with a tractor is roughly 1 gallon per acre; call this 140,000 BTU/ac. 5 passes per year (pre-seeding, planting, 2 fertilizer/spray passes and harvest) requires 0.7 million BTU per acre per year. The yield of corn stover from maize is roughly 2 tons per acre per year; at 15.8 million BTU per ton, the excess non-food biomass is more than 20 times the necessary fuel. The energy required to fix 150 pounds of nitrogen, assuming 1.5 moles H2 input per mole NH3 and 50% efficiency of production, is about 3.4 GJ or 3.3 million BTU. This is a small fraction of the energy in the byproduct stover. Crops like wheat and soya are going to be closer to the margins and may require some cross-subsidy (maize is somewhat of a star performer), but the claim that net productivity must increase by 50% is clearly not supported by the evidence; calculations suggest that no increase is needed at all.
I didn't find anything worth writing up in the next several paragraphs and I'm out of time for tonight, so tomorrow I hope to resume at "What about waste?".
When it comes to survival, i'm ok with the idea of limited use for biomass--if all it does is sustain agriculture, that is plenty. We are not going to maintain the current energy use for much longer unless there is some unlikely breakthrough or the laws of physics change. Even the optimists think we have 40-50 years of fossil fuels to play around with. They think that is not a problem because we'll come up with something else to substitute for oil (such as biofuel). Many on the Oil Drum believe we will have serious problems within 10-20 years as inputs from oil decline. Because of the twin problems of resource depletion and climate change (depletion of oil forcing increased coal use), we are caught in a vise grip. We will use less energy, some people will have a shorter life span.
Searching for "char" I see no mention of biochar in article or comments. Charring and burying biomass has many advantages: it removes CO2 from the air, offsetting coal use elsewhere. We have a lot of coal which can serve to bridge BAU to a future where non-fossil energy is available as a result of technology improvements in solar, nuclear (e.g. closed cycle complete burnup of fuel), etc.
Buried biochar (which is activated charcoal if produced at 600C rather than 400C) adsorbs many of the minerals which otherwise leach out of the soil.
Buried biochar permanently sequesters the carbon.
I think there are other advantages I can't recall just now.
Eventually, all of our energy inputs will be sunlight energy or sunlight derrived fuels. Nuclear and hydrocarbons are finite. Hydro is just sun evaporated water condensing and falling into a reservoir. Wind is sun powered atmospheric circulation. Tides and geothermal will likely be miniscule. Looks like in the long term the only practical solution is a massive die-back in human population.
Solar provides about 100,000x as much energy as humans need. We have plenty.
Closer to 10,000 by my calculations, but what's an order of magnitude when we're this far away?
Ah, yes - I was thinking of the 100,000TW the sun provides. Our consumption is on the order of 5TW (varying depending on how one counts), so you're right - about 20,000:1.
Hi Folks,
An interesting example of a low entropy self sustaining farm is give here, in the example of an project called 'Dream Farm 2'. It is based on George Chan’s Integrated Food and Waste Management System
The main concept is built around re-designing our energy and food systems so that the waste produced by one part of the system becomes the feedstock for another part, as happens naturally in any complex ecosystem. One of the main problems with most of our current systems is that they are linear, as espoused in the Story of Stuff.
As regards the biomass question, this then becomes part of a cycle, just one of many in the larger system, with energy drawn off during another cycle in the system, say in the composting process that might produce methane which could provide a small amount of power for either a tractor, or to run some other process on the farm, such as pasteurising milk, or heating a fish tank. The focus is on the circular energy flows and the minimisation of entropy, as opposed to our hugely wasteful linear systems including energy, which are mostly one shot processes - raw material in - product and waste out.
L,
Sid.
You can do considerably better than you think. If you can either torrefy or pyrolize some biomass to a non-hygroscopic, less biodegradable form, you have a compact storable fuel which can be stockpiled and fed to a gasogene later. Small amounts of biogas (not needing large, heavy tanks) should be sufficient to start tractor engines and provide pilot ignition for gasogenes. If the gasogene is 2/3 efficient (roughly the efficiency of converting carbon to carbon monoxide), it would take about 15 pounds of charcoal to replace 1 gallon of diesel fuel (1.8 kilos to replace 1 liter).
And we can do still better, by not assuming that the farm needs to be an independent system.
Far better to power extended-range electric tractors using wind power, and use the charcoal for the minority of the time when recharging/battery swapping is inconvenient.
Algae: The Green Crude
Algae is renewable, does not affect the food channel and consumes CO2. Algae can be harvested 2-3 times every 24 hours. It's the fastest growing plant in the universe. Algae farms are scaling-up today throughout the US. All Big Oil has invested in algae! Exxon and Bill Gates recent $700 million investments in algae were interesting investments. To learn more about the algae production industry you may want to check out a trade association called the National Algae Association.