Tech talk: The formation of coal and its forms
Posted by Heading Out on April 18, 2010 - 10:59am
The tragedy in West Virginia this week, with the loss of 29 miners, emphasized the dangers that can arise in the production of fossil fuels. While the United States mines are, in general, much safer than those of many countries around the world, any death is a loss, and so I thought I would write a short series of posts on coal mining, from its origins to the methods of mining that are used today. Hopefully, as the series progresses, you might get a better understanding of the world in which the modern miner works. I will throw in a little history, since it helps to explain the evolution of some techniques. I intend to cover some of the other uses of coal, beyond power plants. The coal at the Upper Big Branch Mine, for example, is a metallurgical grade coal, used in steel manufacture. The loss of production from the mine is already having an impact in that market.
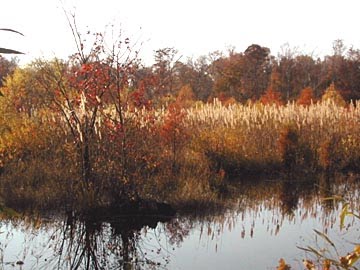
So, to begin, I am going to chat a bit about the origins of coal, where it came from, and some of the limits on estimating how much there is, and how much we are going to get out of the ground.
On the occasional morning each week I exercise and watch a video to help pass the time. About a year ago I watched the Cosmos series with Carl Sagan. If you get to about 9 minutes into the 10th episode he talks about how the Sun and planets rotate about the galactic center (the middle of the Milky Way) at somewhere around 220 km/sec. (The actual number has recently been increased about 15% to around 568,000 mph). Bear in mind, however, that speed is generally considered relative to something else and so different numbers can be found. This means that it takes about 250 million years (according to Sagan) for the Sun to circle the center, and as it passes around the center it will move through the four major arms of the galactic structure. And as it moves through these different zones, so there are different conditions that the Earth and the Solar System encounter and these changes reflect on the conditions that helped form the rocks that are now beneath our feet.
Geologists have been able to divide the history of the Earth into different epochs, with changing conditions leading to different types of rock being deposited, depending on what the surface conditions were at the time. In those different epochs temperatures varied, with the following plot coming from the Paleomap project.
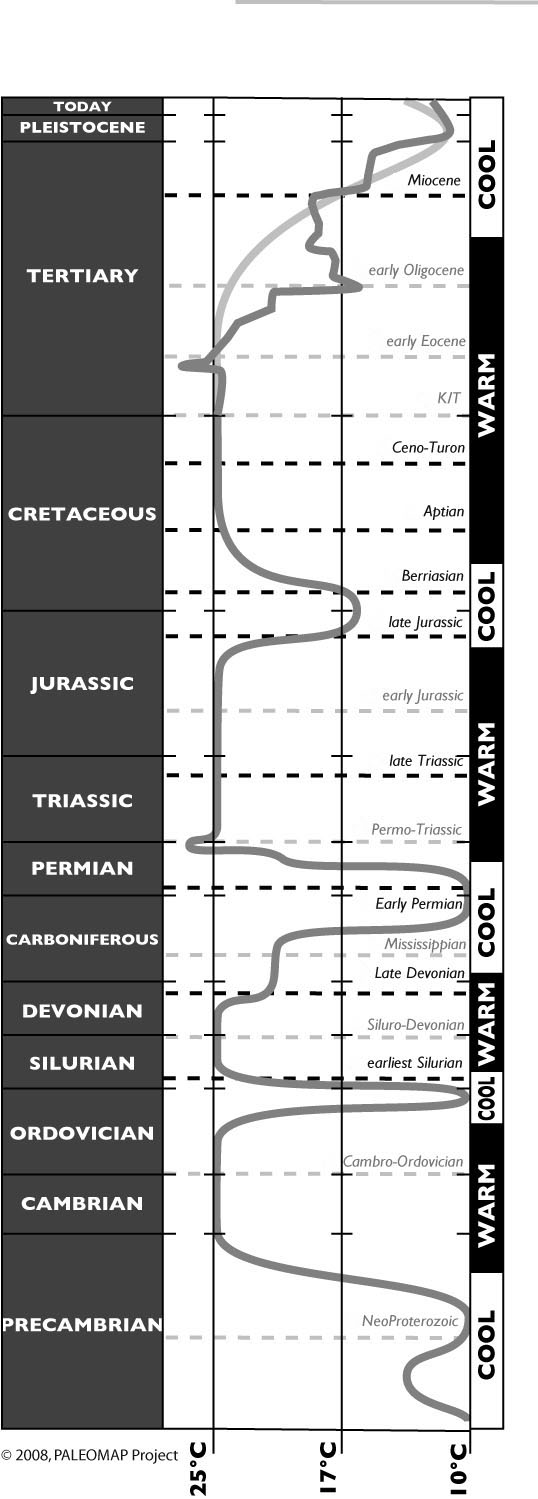
The period that I want to talk about today is known as the Carboniferous. It was some 300 million years ago, and so a bit more than one revolution of the Sun around the Galaxy. Back in those days, about 30 million years before the time of the dinosaurs (which appeared about that 250 million year ago time when the Sun and us were in about the same relative place as we are now) the landmass of the planet was much different than it is now, and Continental Drift had not yet got the continents to their current position.
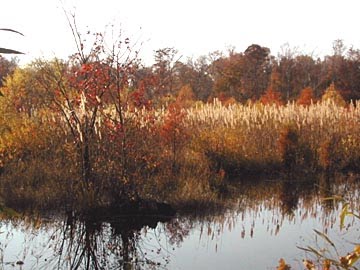
The late Bob Stefanko has written that the Great Dismal Swamp is probably the best current place that represents the type of conditions under which, back in Carboniferous times (about 355 to 290 million years ago) the various vegetative fragments fall into the water, and are slowly compressing to form a layer of peat. The peat layer in the swamp is about 7 ft thick, which may ultimately compress and ultimately convert to about 20 inches of coal. The swamp is slowly sinking, allowing the vegetative mat to continue to deepen and slowly built to a greater thickness. The trees, however, back then did not look quite the same as today.
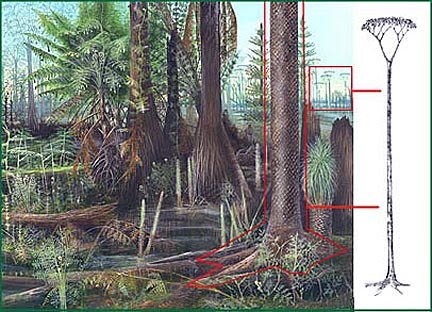
Back in the Carboniferous Bob Stephanko noted that the speed at which the layers formed was likely about twice that at which the current Swamp is growing, and that, due to the different levels of pressure required to form them, it would have taken about 160 years to lay down what is now a 1 ft thick layer of lignite; 260 years for a foot of bituminous, and about 490 years for a foot of anthracite. Since it is more worthwhile to mine thicker coal, and eight-feet is a nice working height, this would have required about 2,100 years of steady growth to lay down the layer of vegetation that formed the Pittsburgh seam in Pennsylvania. The original areas over which these forests and swamps grew were vast, and the cycles of deposition grew as the land distorted, with multiple seams being deposited in some cases, and a single thick seam in others. But how has it survived? How much is really there, and how much can we actually produce?
I thought I would split those questions into several bits, and in this one, I’d try and explain some of the reasons why, while oil companies often get more oil out of the ground than they anticipate, it is often the other way out when you are mining for coal. So, consider, if you will, the map from the site above, that shows back in Serpukhovian times that the planet looked a lot different than it does now.
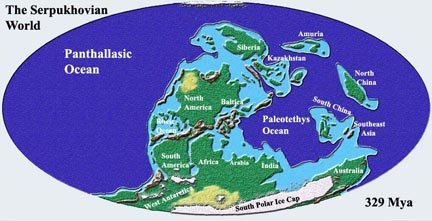
With all the continents together, as they were, they have had to travel a considerable distance over the past 300-odd million years to get to where they are today. Along the way they have bumped into each other, and in the process caused mountain ranges to rise, and fall, and there has been quite considerable erosion of the surface between times. And when the land fell then the seas would deposit more layers of rock, so that the coal as it was formed by the pressure of the rocks that overlay it, and the increase in temperatures that the coal would encounter as it moved deeper into the earth. These would change the original peat into the different types of coal that we know today. These start with a brown coal, or lignite. This is mined today in Texas, among many other places, and the transformation is not yet totally complete.
The color of lignite is usually a very dark brown . . . .It contains more or less clearly separable pieces of plant material identifiable as lignitized roots leaves, twigs, and tree trunks; but besides this material there is a considerable amount of earthy to dense, more or less friable material that cannot be identified as a botanical entitiy without the aid of a microscope.”
With increasing pressure, heat and time, the brown coal (which often has a very high moisture content) is transformed into a sub-bituminous coal. This may have between 42 and 52% carbon and 19 to 26 magajoules of energy per pound. That in turn, if the conditions exist, will transform into a denser, harder coal that is the most commonly found around the world, bituminous coal. And if the pressure continues, then the highest quality coal, anthracite – at 86-97% carbon may be formed. This latter is relatively rare in coal fields, being less than 0.5% of the coal in the United States, for example.
Even though the original coal material was laid down horizontally, as the continents moved these rocks became folded and tilted. Thus, for example, in Washington State beds, and in Colorado coal seams may end up lying at very steep angles. The geological way of expressing this is to talk about the dip of the seam, that being the maximum angle to the horizontal, while the strike of the seam is the line along the horizontal perpendicular to the dip. Thus beds in Washington State may dip at between 20 and 60 degrees.
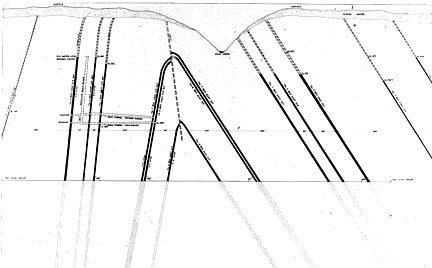
There are considerable problems when the seam gets to dip more than a few degrees. Bear in mind that machines don’t easily move around slopes that are more than fifteen degrees, and you might understand why, for example, it has been very difficult to mechanize the mines of what once were the most productive in the Soviet Union, but now belong to Ukraine. These are the mines of the Donetsk Coal Basin, where
During the communist era, this region produced most of the Soviet Union's coal, and reaped the high wages and prestige that came with it. Statues of miners were erected in squares; villages were named after famous engineers.
The tide began to turn in the last 20 years of Soviet rule, when the flow of funds from Moscow dried up. After communism's fall, the mines were exposed as the money-losers they are, at the same time that economic pressures devastated their customers. In a decade, the demand for Ukrainian coal dropped 45 percent.
Now Donbass is clinging to the two-thirds of its industry that remains. Viktor Yanukovich, the regional governor, said in an interview last month that another 30 of the region's 89 mines would eventually have to close. But he said he needed at least five years to accomplish that and needed an average of $18 million per mine to provide the miners benefits, retrain them and safely close the pits. "I am not against closing down the mines," he said. "But not as it was done before."
Talk of closure is anathema to Anatoli Goncharov, the director of Gaegova mine, which is located in a bleak village outside Donetsk. His mine produces 360,000 tons of coal per year, less than half what it did in the mid-1980s. Because of the steep angle of the seam, half of coal must be extracted by hand, with hammers.
It costs the mine nearly $30 to produce a ton of coal -- $11.50 more than the coal is selling for. Even with $2.7 million in state subsidies last year, the mine failed to break even. The Ministry of Fuel and Energy has put it on a list of pits that cannot be saved, the mine director acknowledged.
(Note that the story is from 2002). At the time the region was being considered as a future source for coal bed methane. There is, for example, a prospectus as part of the methane to markets program.
Coal seams can be mined as deep as 4,500 ft, but the methane and other problems (including keeping the roof from falling in) have made these difficult conditions to work in, so that while there is still coal in the ground, it is becoming increasingly uneconomic, under present conditions, to extract it.
So one of the first problems that the mining engineer has in determining whether to count coal as a reserve is whether or not it is either practical or economic to mine it. This means that, in general, it must be machine mineable, since the productivity of a human miner is no longer sufficient, in many countries, to cover the costs of labor and support.
The second problem is to ensure that there is enough of it. And so before deciding to mine the company will drill cores down to the coal seam over the planned area that will be mined. These don’t, at first sight, need to be that close, since the initial intent is just to see what is there and that it goes out far enough to make it worth while to sink the mining shaft (which costs several million dollars).
However, even the best attempts at accuracy with this planning do not necessarily work out. Consider for example, the case of the mine at Glenrothes in Scotland.
The primary reason for the designation of Glenrothes was to house miners who were to work at a new super coal mine. This was to be the most modern of the day and was built west of Thornton, an established village south of Glenrothes. The Super Pit was named the Rothes Colliery and it was officially opened by the Queen in 1957. Iconic photographs of the young Queen going down the Rothes Colliery in an all-white boiler suit and helmet are still regularly shown in programmes about the royal family. About 5,000 miners were to be required to produce 5,000 tonnes of coal per day, and huge railway yards were established. The pit was to have a working life of 100 years. The planned long-term benefits were to be huge, and the driver for economic regeneration for central Fife. In 1961, 4 years after opening, the huge investment was written off and the mine run down because of un-stemmable flooding.
There were actually a couple of problems at the mine that could not easily have been foreseen by the drilling pattern. If you look back up at the picture of the Washington coal seams you will see the almost vertical line in the middle of the picture, with the black lines representing the coal seams vertically offset one from the other on either side of it. This is what is called a “fault”, and over the course of time the rock was moved to the point that it broke here and created this fracture in the ground. The rock then moved on one side relative to the other. (Like, on a very small scale, the movement that occurs across a major Earth fault when there is an earthquake).
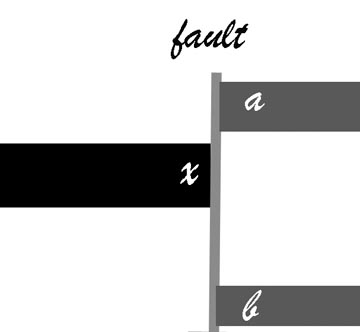
With the movement the rock that lies along the fault gets crushed, which can make it easier for water to flow through. So that if some of the rock, on either side of the coal seam, contained water, then when the mining operation reached to fault, then water could flow through the fault into the mine. There is also another problem. Say you are in charge of the driving of the tunnel that reaches the fault. Suddenly, in front of you, the coal turns into rock. You realize that you have hit a fault, but which way did the coal go – did the ground hade (move) down, or up. The rational way to find out is to drill up or down until you find where it went. Well that takes time, and money, but the worst isn’t over yet. Now you have to move the mining operation that say 20 ft that the coal has moved up. This means driving tunnels upwards, then moving the equipment up, and then recreating the mining pattern, and then moving on. All this development has been in rock, so you haven’t been earning any money from the area while this was going on. And if the difference in height is too great, then the area is abandoned for the time being.
What happened at Glenrothes was that the ground had a lot of these very small faults. They were not apparent from the initial cores, which were quite widely spaced, but over a period of four years they were found sufficiently often, and with a lot of water in them, that it became uneconomic to continue to operate the mine. The mine was estimated to have reserves of 183 million tons of coal, to be able to produce a million tons a year, and to have an operational life of 100 years. In the five years of its life it produced around 700,000 tons in total. (The full story of the mine – which had many problems that I have glossed over – can be found in The Disappearing Scottish Colliery by Robert Halliday.)
There are a couple of other problems that happened at Genrothes that are also fairly common, but that can make life miserable for the miner. Let us go back to when the coal was first still vegetation in the swamp. Swamps are not great flat growing areas that are all even. Streams work their way through, cutting channels through the peat and filling them, often with sand, or other material washed down from local hills. There are dirt hillocks and, after storms the land might be flooded with layers of silt, that can be feet thick.
Now move forward some 300 million years. The sand and silt have turned into sandstone and shale, but they did not compress as much as the vegetation. So now instead of there being a nice 8-ft thick seam which the miner has a machine to extract, the coal has a layer of rock in the middle (the silt layer) that is about a foot thick, but that wanders up and down in the seam, and then gets thicker, as it approaches an old river channel so that the two sections of the seam are now about four feet apart. The mining machine grinds off the coal with picks but when these start to run into rock they wear away very quickly, so that mining slows down and becomes more expensive. And then the machine reaches the sandstone filled river channel and things get just a little more difficult.
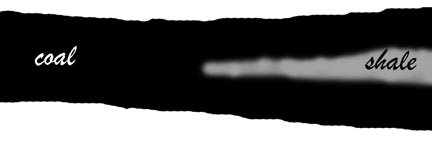
Now the coal is still there, there are a lot of tools to help get it mined that were not around at the time of Glenrothes, and it is not really necessary in all cases that miners be physically present at the machine when it is mining. But some of the time we still haven’t got the answers that would bring some of this coal back to the point where it is considered a reserve. And there is some doubt, therefore, as to what we call a reserve and what not. But we’ll leave that discussion to another day.
Thank you, Headingout. It's nice to see a little geology here at the Oil Drum.
~Roger
I'm heading out-side soon, so this ruthless copypaste may help:
Lifted from here, for educational purposes only, http://www.appaltree.net/aba/coaltypes.htm
Is this true? Are the galactic "arms" not part of the rotational structure as well? How would "passing through" a galactic "arm" affect biomass and accumulation of organic material terrestrially? Just curious, cosmology/cosmogeology/astrogeology wasn't my forte.....
We pass through the spiral arms (and they're still working out how many major arms there really are):
http://en.wikipedia.org/wiki/Milky_Way#Spiral_arms
Elsewhere in that wikipedia article is
No, and the OP is wrong on this too. Our solar system, like the vast majority of material in the galaxy, is a part of the spiral arm structures. The spiral arm structures are quite permanent, on any earthly time scale. They can be described as "compression waves". but only as their elements - stars and other matter - make them so. That is, there is not a field of variously moving star systems travelling through a different field causing the formation of the spiral arms; there are dynamic (though locally relatively static and consistent) large-scale structures in the galaxy which we call "spiral arms" and can describe as "compression waves", which are simply one field consisting of the arrangement of matter within the galaxy, including our solar system. Our solar system doesn't traverse through different arms over time, it is an element of the same arm it was "born" in.
(on edit - though I certainly defer to the writer's greater knowledge in everything else here!)
I have given you several of the sources that I got the information from, but they are not exhaustive since I was aware of this before watching the Sagan series. There is also some correlation of the changes in geology with the relative location of the solar system relative to the various arms of the galaxy that it would have passed through, which is supporting evidence for the statements.
"Galactic Habitable Zones"
The solar system is in the smaller Orion-Cygnus arm. The idea of the "Galactic Habitable Zone" is part of the Rare Earth hypothesis, and like many of its aspects has been challenged. Ostensibly since the SS avoids the major galactic arms we have also avoided being near supernova bursts that would adversely impact life.
What connection is there between deep time geology and extrasolar context? I was under the assumption that once the gas giants had sucked up most of the large impactors the primary factors in geology were terrestrial.
It appears computer modeling is used to explain the arms which are actually transient themselves as they usually form and disappear and reform but galaxies at almost any time have similar looking arm structures, if I understandthis article by Ray Carlberg correctly. If I understand you correctly, early on as the earth passed through these arms while it was still forming it accreted recognizably different materials. The latest take seems to be that the Milky Way is a two armed rather than a four armed galaxy however.
I'm looking forward to this coal series. Thanks for putting it up.
If we're going back through deep time why not start with the big bang? Then discus novae and super novae, the abundance of the elements up to Fe and the formation of those "higher" on the chart, presence of H, C, O, N, etc. I'm still unsure how transits though galactic spiral arms affect coal formation and types on earth. Relating galactic transit time to deep time passage on earth is poignant though.
what is the co2 product of burning the various grades of coal ? say tons of co2 per ton of coal or better yet maybe tons of co2 per quadrillion btu or some such units, anyone know or have a source ?
The ratio of CO2 mass to some mass value of coal depends upon the coal itself. However, the mass of CO2 formed per unit mass of carbon is always the same (assuming carbon-12 and oxygen 16): 3.6667
Take the ton of coal, multiply by it's as-fired fractional carbon content (e.g., 80% = 0.80) and then multiply by 3.6667 to get the mass of CO2 (in this case, tons) per ton of the particular coal fired.
This assumes complete burnout (no carbon loss) and little carbon monoxide formation during the combustion process.
I will add a bit of biology to this. Interestingly the formation of coal beds suddenly stopped right around the time that termites evolved. After termites evolved the only coal beds that form are in areas where termites would not have survived. Its circumstantial, but I think its a fair guess that major new coal beds will never form again.
That is really interesting. Can you provide a link to this? I ask because it seems to me that the termites wouldn't really be dispersing the carbon that much. Don't they live and die in roughly the same place?
What kind of animal has the highest worldwide output of flatulence?
http://www.fart-sounds.net/facts_on_farts.htm
http://www.uspest.com/termites-are-cause-alarming-rate-methane-emissions
There go your hydrocarbons! Some soldier termites literally hold it in, turning themselves into little bombs.
Perhaps a better way to convert biomass!
http://en.wikipedia.org/wiki/Termite#Soldiers
This is one article I found that seems to be related:
There you have it. Increasing greenhouse gases and deforestation...... by roaches and termites.
And we thought we were special in so many ways.
Does that mean we will be around as long as termites and roaches ?- )
This is an amazing find from the period (300 Mya):
Giant Fossil Rain Forest Discovered in Illinois
http://news.nationalgeographic.com/news/2007/04/070424-forest-fossils.html
Is your sketch of a WA state mine of any mine in particular? I found this on the Centralia Coal Mine:
Experimental Open-Pit Mine Slope Stability Study (1969). The Centralia is WA state's largest mine; btw it's purely a coincidence that the town of Centralia shares its name with the infamous methane leak ghost town in PA.
Found this, too: HistoryLink.org- the Free Online Encyclopedia of Washington State History
It is from a paper in the 1970's so I will have to get the information from my office tomorrow - sorry for the delay.
The slide came from Don Hume of Carbon River Coal back in 1975. It is the area around Roslyn, which became more famous for the filming of Northern Exposure that took place there. It was also one of the sites for the early trials of hydraulic coal mining in the country.
It is not only the solar system that rotates around the galactic center. Our neighbors, Centaurus, Sirius, etc. rotate with us and so does our arm of the galaxy, with small relative differences in speed. The other arms also rotate. The whole galaxy rotates and that is why there are spirals. Thus, the solar system will not pass through the other arms of the galaxy.
As I understand the theory of the effect of the rotation of the solar system about the galactic center, it is not the actual rotation that is the problem, but the "nutation" or "nodding" of the position of the solar system as it rotates. I.e. during some of the time during the rotation we are in the plane of the galactic arms and at other times, we are not. I believe that this is hypothesized to change the amount of galactic cosmic rays (GCR) that impact on the earth. Cosmic rays are very high energy particles (mostly hydrogen atoms - protons) accelerated to ridiculous energies by interstellar "accelerators" (magnetic fields in deep space) and these impact on the upper atmosphere causing a cascade of secondary particles that reach the surface (and certainly reach the cloud forming regions of the upper troposphere). Clouds at these altitudes (as opposed to statispheric clouds) are known to reduce temperatures on the surface of the earth (shade). If it is true (not certain) that GCR changes causes changes in the amount of low-level cloud formation (Svensmark theory) GCR changes might be partially responsible for long-term changes in global climate (100s of millions of year).
However, there are other "shorter" term effects on global climate. The most obvious of these is continental drift. The position of Antarctica makes a huge difference in global climate. Access to the South pole for ocean currents would GREATLY increase the temperature at that pole. (e.g. the Arctic is much warmer than the Antarctic because of ocean currents accessing the North Pole, under the ice).
The presence of Antarctica at the South Pole may be the principle reason for that the Pleistocene epoch is an Ice Age (we are due for another one "soon"). Another possibility is the gradual cutting off of the Bering Straight to deep ocean currents from the Pacific Ocean. There is plenty of heat at the Equator to keep the Arctic Ocean ice free for the whole year, BUT it has to get there!! The position of the continents makes that difficult.
All of these effects are very interesting but also highly uncertain. The global climate is not easily modeled !
Love this stuff. Keep up the good work.
IWylie
With all due respect, any potential effects of continental drift or changes in GCR due to galactic rotation are absolutely negligible on any time-scale that is relevant for our present civilisation (or even the succeeding 2 or 3, or 4...). If there are still humans around in 10 million years they may have a slightly different continental configuration...
The lobbyists who want to kill carbon mitigation schemes seem to think coal mining is a natural part of human destiny. Presumably they think coal mining is a major activity on other planets. However the existence of major coal deposits requires the right geological history and atmospheric composition. Thus if major coal seams start from the Carboniferous and coal is half depleted we have
- 340 million years
time to convert primordial CO2 to coal
- 200 years
time to convert 50% of coal back to CO2.
So the soil to atmosphere rate of carbon transfer has drastically shifted. Human industrialisation can be likened to fleas discovering a healthy dog and making it sick by demanding too much from their host.
I think evidence is mounting that supposedly abundant coal is getting harder to extract. Mine deaths in the US and China, mountaintop removal in the US, prime farmland despoiled in Australia, coal ships running aground on protected coral reefs. Time to move on from coal.
The actual health of an industry cannot really be gleaned just from transient headlines. The coal mining industry continues to grow at a relatively steady rate, and may surpass oil as the world's predominant fuel within the next five years. Its "death" has been foretold many times in the past.
OTOH peak oil supply could cause an early peak in coal demand. If 'stuff' can't be delivered to market then there will be less need for electricity and cement that use coal directly. In particular there is little point getting 'stuff' made in China with cheap labour and un-carbon-taxed coal if the shipping cost is too great. Natural gas straddles both the stationary and transport sectors but I don't see how it can be used for both for more than a decade or two.
Peak Oil isn't no oil. Half the oil we produce today could keep ocean shipping, heavy construction and remote equipment running quite a while, probably keep the plastics industry supplied too. If we shift to back heavily to coal there will be enough petrol to get it out, and there could well be enough electrified transport to move all of our goods around and keep coal demand growing. I'd really rather we got most of our electrical power some other way though and use this little breather natural gas is going to give us to make that happen. Coal will be usefull in industrial applications for a long, long time and we pretty much have as much as we are going to get in the ground right now.
Thank you for this article, very helpful in explaining why huge reserves may not necessarily be capable of translation into production.
Which countries still have the potential for a major increase in coal output? Seaborne trade in coal has grown phenomenally over the last 30 years. In Asia, coal accounted for 69% of the growth in primary energy consumption in the six years 2002-08, but in country after country the production is failing to keep up with domestic consumption, and the region seems increasingly dependent on coal exports from Australia - even with a massive nuclear rollout. On the other hand there may be new sources - Mongolia and the Russian Far East are both said to have large reserves.
I have explored some of the country trends at
www.apolloinvestment.com/asianenergy.htm
- as noted on Drumbeat at the time of writing, but with some comments on coal added subsequently.
OTOH, that growth may bring a much earlier end to coal production (since it also has an EROEI to contend with) and this is a point that Dr. Bartlett has made about exponential growth and the exhaustion of a finite resource.
We already have seen the decline in coal grade (and heat content). A unit train of coal, nominally 100 cars of coal each with a net weight of 100 tons per car, just does not go as far as it used to and it takes more cars (trains) to keep the heat input rate up, assuming that the puverizers can actually handle the increased mass. (Note: one of the reasons some, but not all coal-fired electric generating units can switch from bituminous to subbituminous without severe derating is that the coal does not need to be ground as finely. Most bituminous coals are agglomerating, meaning that when exposed to the furnace heat/burner heat, the coal particles tend to stick to one another reducing the effective surface area available for combustion. Subbituminous tends to be non-agglomerating AND, owing to a fairly high inherent moisture content, tend to explode like popcorn when exposed to heat, increasing the surface area for combustion. Where a bitminous coal must typical be ground to a minimum of 80% passing through a 200 mesh screen, subbituminous coals can get away with 65-70%. Other things like ash fusion temperature can dramatically affect combustion sharacterisitics and limit load, but that is another of many factors that can limit or enhance performance.
If we start using coal for CTL (which gives you a yield of anywhere from 1.95-3.2 BBL/ton, depending upon coal grade, with a nominal value of just under 2.5 BBL/ton), the growth and the depletion will be much faster. As Dr. Al Barlett has pointed out, it takes a simple bit of calculus to come up with the exhaustion equation for growth of consumption of a ficed resource.
Thanks for an interesting article.
For some reason I find discussion of the geologic history of the planet quite interesting. Both in terms of the conditions under which things like coal and oil were formed, but the configuration of the continents, the climate, and the types of living things that were around at the time..
Careful ericy....thats what sucked me into being a geologist. Growing up in New Orleans even sand was a novelty let alone rocks. Took freshman geology in college and saw a world I never knew existed.
Thanks, Heading out for a wonderful, interesting and simply written post covering geology.
First, let me say that I thought this was an interesting article to read. I do have one question though that I would like to ask the experts here. My basic understanding of where oil comes from sounds very similar to how you describe the formation of the coal deposits. What determines whether a deposit of dead organic material becomes coal or oil? Thanks.
They largely come from different sources. Oil comes from algae that existed in the water and wetlands, while coal comes from the trees and woody material.
Thanks for the reply and I have one more question. What causes the algae to form oil and the trees to form coal? They both contain organic materials that are subjected to heat and pressure and I'm trying to figure out what on the path to oil/coal leads one to form oil and the other coal. Thanks again.
One of the reasons that algae are such an area of interest today as a sustainable source of fuel is that certain of the species can have oil contents (well actually the lipid) which can get to 50% of the mass. It helps them to float, among other things. Thus from that point of view the pressure and heat merely change the hydrocarbon form, and if heated too much it transforms to natural gas.
There is little natural oil in the trees and woody material, and so it gets compressed and heated more of the non-carbon material changes to a form that can leave, and the carbon (coal) is what is left.
Thanks for explaining this to me. That makes a lot of sense.
If you have a few minutes, there are some excellent videos on the subject of oil formation here:
http://www.abc.net.au/science/crude/
One of the concepts that I found really interesting is that oil collected at the bottom of pink toxic seas beneath a runaway greenhouse atmosphere... !
Those look interesting. I'm at work now, but will definitely take a look at them when I get home tonight. Thanks for sharing.
Thanks for the questions and the answers.
First, thanks to Heading Out for the addition to the excellent series.
I'm trying to decide what the relevance in future may be of in-situ energy / fuel production from deposits presently known to exist but too difficult to mine? It seems to me that in many locations, it will in future make sense to convert coal to coal-gas right in the coal bed, then extract the gas and use it at surface or ship it by pipeline. Of course it will be limited to linking coalbeds to markets which can be joined by pipelines only, eg. will never likely send Australian coal to China. What is your "feel" for the state of this tech? Feasible but not (yet) economical? Not feasible on any scale? Only serious test I know of was done in Australia several years ago. The sponsor claimed it was successfull and economical at that time. It seems to me that if the kinks are worked out of the technique then the whole question of coal reserve sizes goes through the roof, eg. the deep beds which underlie pretty much the entire province of Alberta, Canada. Nearly incalculable resources in place too deep for surface mining and too low quality for underground minimg.
Lengould,
As I understand the current glut of natural gas in North America it is partly because of the application of "fracing" and horizontal drilling on the shale and coal beds (as you imply above). Natural gas is a much better fuel than coal and conversion of ground transport to natural gas is quite possible. It is not ideal for large ships and trucks but cars can quite easily be converted to natural gas.
I suspect that you are quite right that the potential reserves are indeed huge (but difficult to quantify). The depth that coal mining can be economically performed is much less than the depth of horizontal drilling. The problems with mining of coal (thin seams, shale inclusions, faults, "coal gas" - methane), are substantially reduced or eliminated by the use of the techniques pioneered for the oil industry.
Of course, this still means that the ultimate natural gas reserves are large indeed. The only problem may be that the CO2 concentration will continue to climb. Let us hope that crop growth increases off-set any negative effects from the presence of increased CO2 in the atmosphere!
IWylie
Actually, in-situ coal gasification and coalbed methane are completely unrelated. Coalbed methane is simply a means of extracting a very small fraction of the energy in a coal seam by removing the natural methane locked up in it. In-situ gasification is a method of drilling (one or more) injection wells and (at least one) extraction well into a deep underground coal seam, fracturing it, starting it burning, then injecting controlled amounts of oxygen and water to initiate the water-shift process as using in the gassifier of an IGCC generating station, converting the coal + water + oxygen into CO / CO2 and hydrogen, which are then brought to the surface for use as fuel. Theoretically can extract all of the carbon from deep uneconomical coal seams.
March 17, 2009 - Alberta continues to explore cleaner energy from coal - Clean gas produced more than 1000 metres below earth's surface
A fairly scary proposition if, as me, one believes the smartest way to sequester carbon is to leave the coal in the ground in the first place.
Scarier than a massive nuclear expansion?
41% of all the world's electricity comes from coal and that will increase as the prices for oil and natural gas increase. Hydro, 16% can't increase much as most of the existing sites are built and nukes, 15% costs too much and aren't likely to increase quickly.
Electricity is pretty much a human right.
Electricity will always be produced with the lowest cost fuel available,which is coal.
What is the likelihood that people will agree to losing 50% of their electricity?
Zero.
Better to remove the CO2 and bury it.
Then there will be the energy to build new bridge technology without blacking out
civilization.
What is it that you think might be scary about a massive nuclear expansion?
It looks to me MORE rational to propose that coal-generated electricity with carbon sequestration is FAR closer to a zero EROEI than oil from oil sands.
A lot of interesting material in HeadingOut's previous Underground Gasification article:
http://www.theoildrum.com/node/6164
I asked earlier which countries may still have potential for a major increase in coal output - eg Australia is forecast to increase production 30% over the next few years, but I think that demand may rise many-fold. Does anyone have any views on this?
You are really talking about is ability to export. The world export coal industry is 1 billion tons versus 1.2 billion tons of USA production and 2.8 billion tons of Chinese production. The world production can be divided into USA 16%, China 39%, ROW domestic 31%, world exports 14%. There seems to be unlikely that world exports can be increased to offset demand growth or depletion barring some new technology.
Over 80% of proven coal reserves are located in 6 countries; Australia (#1 export), South Africa(#4 exporter), China, India, Russia (#3 exporter), and the USA.
Russia has the largest ability to increase coal production, IMO.
Other coal rich export countries include Ukraine, Kazakhstan, Canada, Serbia, Poland, Venezuela, and Columbia. Indonesia(#2 exporter) and Vietnam are major exporters with limited reserves.
http://tonto.eia.doe.gov/cfapps/ipdbproject/IEDIndex3.cfm?tid=1&pid=7&aid=6
Thanks. China, India, Indonesia and Vietnam are unable to keep up with the growth in domestic production - Coal Exportland.
The Uppsala gang (Höök, Aleklett et al) have had a good look; check out "A resource-driven forecast for the future global coal production" PREPRINT VERSION (PDF!)
The condensed version of their findings is this graph:
Interesting report. Alaska coal is always of interest to me and drop in recoverable reserves from 65,042 million short ton in 1970 to 2,832 short ton in 2005 is an eye catcher. Big change in reporting criteria. The hypothetical resource of about 3,500,000 million metric tonnes located north of the Brooks Range is of course totally ignored right now.
This article addresses the Alaska reserve issue nicely. On the arctic coal it states:
Though at the moment North Slope coal is fairly inaccessible, as ice recedes and rail is built that will change. Arctic coal could well be joining the reserve base in a big way within a century.