How Long Before Uranium Shortages?
Posted by Gail the Actuary on February 19, 2009 - 10:16am
There is a great deal of controversy about how much uranium will be available for future use. I decided to check to see for myself, and came to the conclusion that we are likely headed for problems within the next ten years. Below the fold are a few things I discovered, in looking through reports available on the Internet.
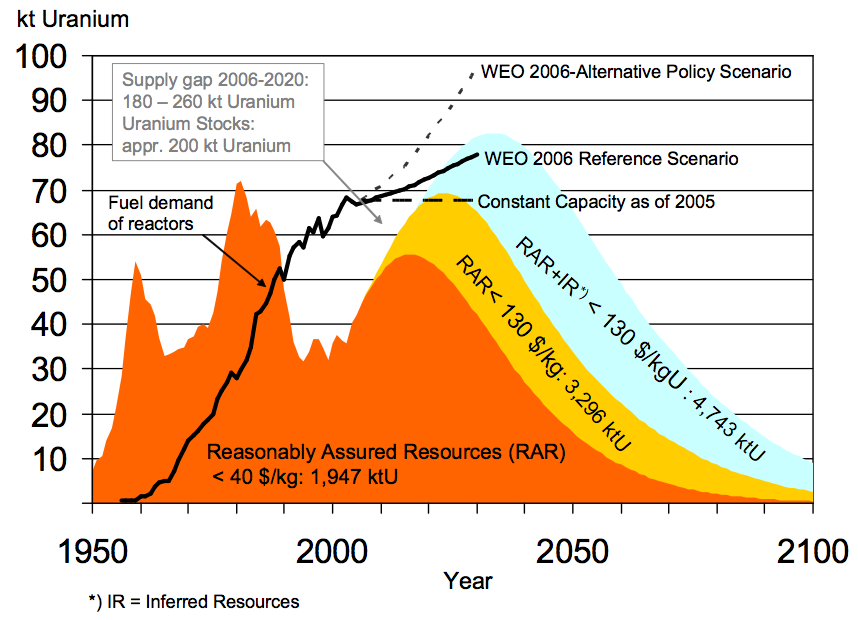
Supply Disagreement
How much uranium is "out there"? One gets a very different answer if one looks at (1) known concentrations that are suitable for ore, versus (2) the amount that is theoretically available, if one considers all of the dilute amounts available in rocks almost everywhere, and in seawater.
Suppose we start from the point of known amounts hopefully suitable for ore. In Figure 1 above, the Energy Watch Group (see this 2006 report) takes three levels of reserves and spreads them out in likely production patterns. The dark orange section represents "reasonably assured resources" that can be economically mined for $40 per kilogram or less. The lighter orange section represents "reasonably assured resources" for $130 per kilogram or less. Current spot prices are about $50 pound, or $110 kilogram, so current prices are now somewhere between these two levels.
The top section in light blue adds "inferred resources". These aren't resources that anyone actually has found and examined. These are resources that one would hope would be somewhere that we haven't looked yet.
Note that in the chart above, there is a likely shortfall even with the light blue "inferred resources" included. The United States has been purchasing recycled Russian bomb material since 1994, and our contract to purchase it continues until 2013. We also have plans to continue buying recycled Russian bomb material after 2013, and to recycle American bombs. These are the kinds of programs which are contemplated in the Energy Watch Group analysis. Even with these in place, their analysis indicates a possible shortfall.
The Energy Watch Group is not the only one who has looked at the question of the adequacy of uranium resources. The International Atomic Energy Agency issued a study in 2001 called Analysis of Uranium Supply to 2050. Its analysis also shows a peak and decline of uranium supply, with a peak occurring in 2024, assuming all resources, including the highest cost resources, can be extracted. If only lower cost resources can be extracted, the peak will be sooner.
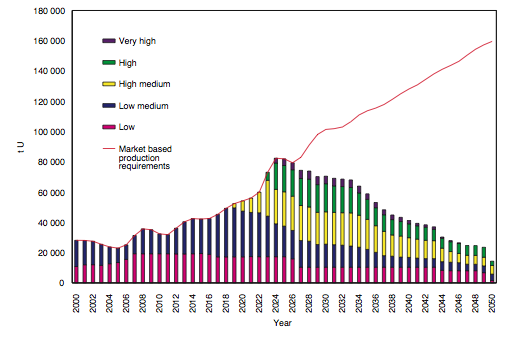
What do other agencies have to say about these projections? The World Nuclear Association issued a report called Supply of Uranium in June 2008 in which it explains how there will be plenty of supply. These are excerpts from the report:
From time to time concerns are raised that the known resources might be insufficient when judged as a multiple of present rate of use. But this is the Limits to Growth fallacy, a major intellectual blunder recycled from the 1970s, which takes no account of the very limited nature of the knowledge we have at any time of what is actually in the Earth's crust. Our knowledge of geology is such that we can be confident that identified resources of metal minerals are a small fraction of what is there.
Uranium supply news is usually framed within a short-term perspective. It concerns who is producing with what resources, who might produce or sell, and how does this balance with demand? However, long-term supply analysis enters the realm of resource economics. This discipline has as a central concern the understanding of not just supply/demand/price dynamics for known resources, but also the mechanisms for replacing resources with new ones presently unknown. Such a focus on sustainability of supply is unique to the long view. Normally-functioning metals markets and technology change provide the drivers to ensure that supply at costs affordable to consumers is continuously replenished, both through the discovery of new resources and the re-definition (in economic terms) of known ones.
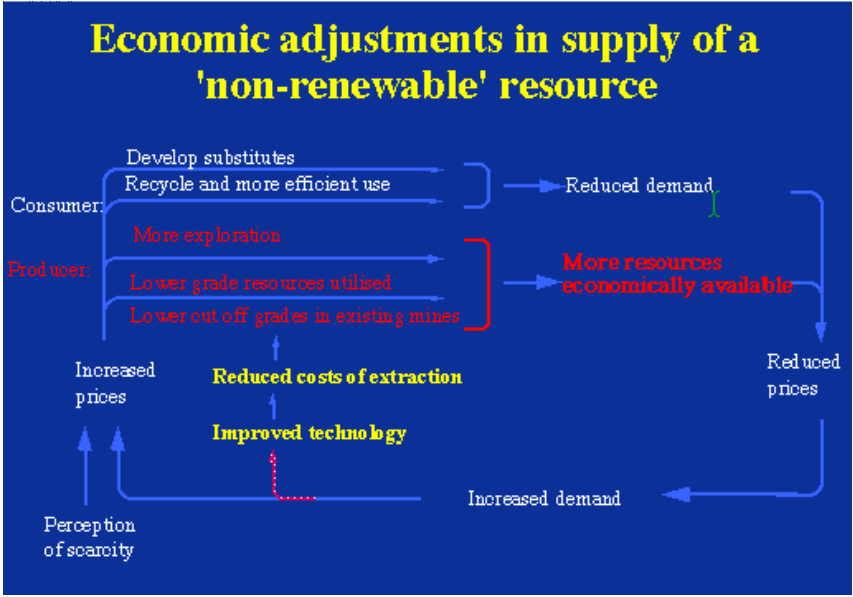
The World Nuclear Association (with the above faith-based statements) also published a report for £375.00 called The global nuclear fuel market: supply and demand 2007-2030. I do not have a copy of this report, but according to the EIA Energy Outlook 2008, WNA is forecasting that world uranium production will peak in 2015, before slowly declining to 90% of its peak level in 2030. It is strange that the report I quoted above, available on their website, fails to mention this.
In this section, I have not talked about the accuracy of the reserve amounts. In several countries, production has stopped or nearly stopped after available resources have been exhausted. If one compares the reserve figures prior to exhaustion to the amount actually produced, one finds that the stated uranium reserves were significantly higher than the production actually achieved. Below is a chart by Energy Watch Group comparing France's reserves with its actual uranium production. This kind of pattern does not give one much confidence that mining companies will actually be able to convert the reserves (as published by nuclear organizations, which are higher than individual company estimates) into production.
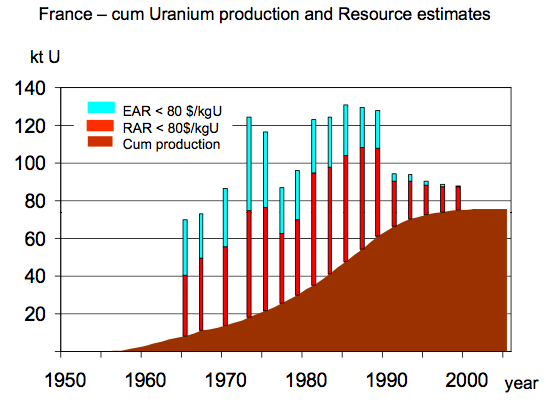
Where We Are Now
Energy Watch Group shows this graph of actual production versus demand through 2005:
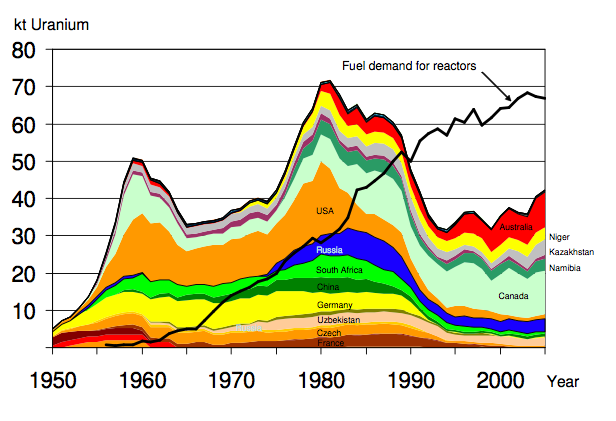
More recent data from the WNA indicates that world production since 2005 has been flat:
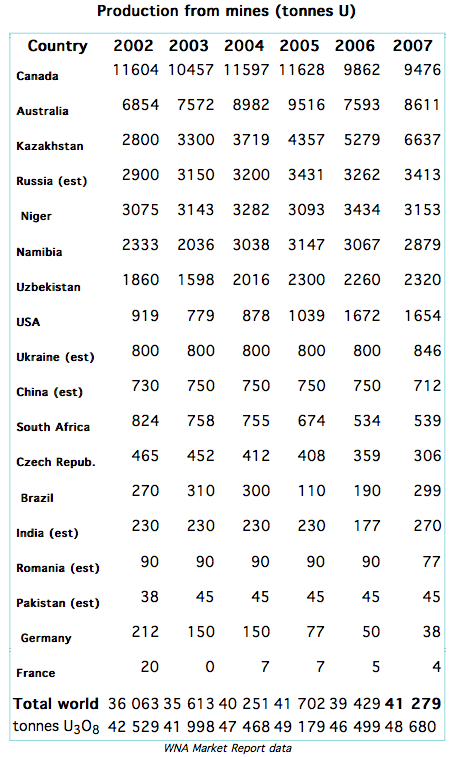
Uranium production for the United States continues at a very low level, compared to the amount we use as fuel in nuclear reactors. Recent EIA data shows that 2008 production has declined from the level produced in 2006 and 2007, despite rising numbers of people working in mines and greater expenditure (see this report and this one):
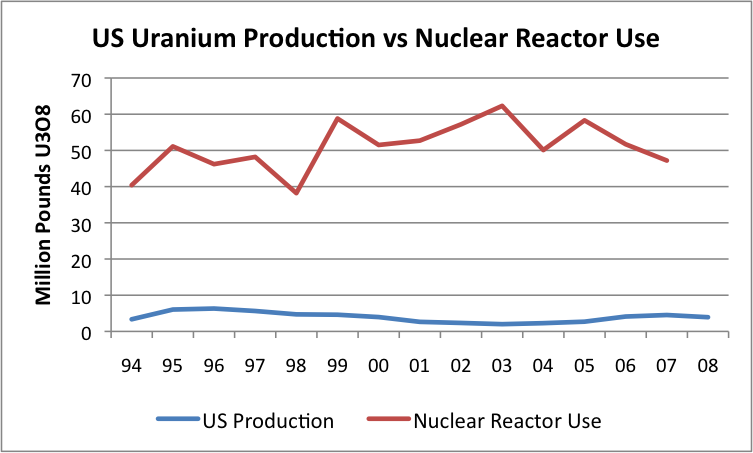
The EIA in International Energy Outlook 2008 says there is no problem: there are lots of reserves (the same ones as in Figure 1, including the light blue). It also says:
The relatively high price of uranium already is leading to increased output. New mines in Australia, Canada, Kazakhstan, Brazil, and India are expected to add 30,000 metric tons of production capacity by 2010.
Adding new mines takes a long time--one often sees 8 to 10 years quoted as a reasonable time frame. Production in 2007 was only 41,000 metric tons, so increasing production by 30,000 metric tons would represent a 73% increase. This doesn't seem to be happening. If we look at news reports, we find that mining companies are struggling financially, because of high debt loads and low prices available for their products. Production plans are being cut back or delayed.
In Canada, the big new project is Cigar Lake, under development by Cameco. I haven't seen production estimates for the mine, but would guess that they are of the order magnitude of 10,000 metric tons of production per year. Cameco began work on the mine in 2001, but the mine has repeatedly flooded. The mine has very high grade ore (20%), but is located below the water table. The plan is to try to freeze the ground to stabilize the area for mining. The most recent flood was in August 2008. Prior to the latest flood, the planned production date was 2011. No new date has been set. Cameco has not given up yet, but one wonders whether the problems with this mine will ever be worked out.
The other very large project which many had great hopes for was the BHP Billiton's Olympic Dam expansion in Australia. The project would have cost about $15 billion, and increased world uranium production by something like 8 million metric tons a year. The expansion is now being scaled back and delayed, because of the current financial situation.
If we read Cameco's latest report about its mine in Kazakhstan, its report is less than glowing. In describing its new Inkai mine, it reports sulphuric acid shortages, the Kazakh government reneging on the agreed upon tax rate, and an after-the-fact change in the subsoil law.
What does seem to be happening is a grab for available mines by countries like China and Japan. These countries will be needing fuel for nuclear reactors and cannot see good long-term sources of supply. For example, Ranger is the second largest mine in the world, representing 11% of 2007 world production. The Japanaese are trying to get a controlling interest in Ranger and in other mines, according to Japan: Securing Uranium Supply.
China has also been trying to invest in mines. It has been having discussions with BHP Billiton, operator of Olympic Dam in Australia.
The United States believes that the free market will provide and is not trying to buy up assets. Instead, we are trying to ramp up US production, but with little success so far. The US is very dependent on foreign imports, as shown in Figure 7, especially recycled Russian bomb material, which currently makes up 50% of nuclear fuel. Since about 20% of US electricity is from uranium, this means that 10% of our electricity supply is obtained from recycled Russian bombs. Most of the remainder of our nuclear electric supply is from other overseas sources. With current world financial problems, one wonders how secure these sources are. Also, we will need to find additional sources when the Russian bomb contract runs out it 2013.
How Do We Mine the Uranium?
The amount of uranium available depends upon what mining techniques are available and how pollution is handled.
When countries first started producing fuel in the 1950 -1980 period, there was little concern about pollution. Open pit and underground mines were used to produce huge volumes of uranium. Without adequate pollution control, there were often serious pollution problems. Eventually, in the United States, Superfund was called upon to try to clean up many of these sites, and people became disenchanted with this approach to mining. The cost of the Superfund clean-up was never charged back to the uranium industry, so analysts looking at statistics such as EROEI got a more favorable view of the industry than would otherwise be the case.
A USGS Report Issued in 2002 indicates that because of dissatisfaction with the pollution problems of open pit and underground mines, these mines are difficult to get permitted. Instead, most mines now being built are in situ leaching (ISL) mines.
ISL mines can't be built everywhere--only where conditions are right for them. The need to use ISL mines has cut back significantly on the sites available for building mines, but reserve statistics (at least in the United States) don't reflect this. The 2002 USGS report says:
Future uranium mining in the U.S.A. will be mainly by ISL mining of large reserves in Tertiary sandstone formations in Wyoming, Nebraska, and Texas. Mining of equally large reserves of uranium in Mesozoic sandstone formations in the Colorado Plateau region is less amenable to ISL.
It also says:
The national uranium resource assessment completed by the DOE in 1980 is significantly out of date. A new Federal assessment, using recent developments in uranium assessment methodology and applying new geologic concepts, would greatly aid future planning for the uranium industry and contribute to the formulation of a national energy policy.
It is likely that reserve estimates for the United States are still using the 1980 DOE assessment, even though it is significantly out of date, and not up to date with mining techniques currently in use.
Clearly, if we were to develop better mining techniques that have acceptable pollution levels and can be used in a wider range of sites, then our ability to extract uranium resources would be improved. As far as I can see, development of additional techniques has not yet happened. Recycled bomb material has been flooding the market for almost twenty years now, keeping uranium prices low. This has deterred investment in better techniques for extracting uranium, both by companies and governments.
The shortfall in supply that seems to be headed our way will be coming very soon--as soon as we become unable to find sufficient recycled bomb material to fill the gap between nuclear reactor needs and current year production, which could be as soon as 2013 (or sooner, if world financial difficulties interfere with imported Russian bomb material before then).
If we need new mines, we should have started years ago, since there is a lag of up to 10 years before a new mine begins operation. If we need new mining techniques, research on these should have started even longer ago. While there may be a whole lot of low level uranium resources "out there", if we don't have techniques to economically extract them and also keep pollution in bounds, the resources are not very useful to us. We may someday develop new techniques, but in the meantime, we are likely to have a large supply gap.
Yawn. What's with Gail becoming TOD's top scare monger as of late?
How long can Uranium last for nuclear power ? 5 billion years at double current world electricity usage.
http://nextbigfuture.com/2008/08/how-long-can-uranium-last-for-nuclear.html
We don't have a way of getting uranium out of the ocean. Ugo did a post earlier, explaining why this is not likely. It certainly is not likely within the next ten years.
I didn't say thorium. Thorium is a separate subject. We had one post on thorium earlier, and perhaps sometime will have another one.
If you read the comments following Ugo's post on the supposed infeasibility of seawater uranium extraction, you'll see that one of the posters checked Ugo's sources, and found that he had misquoted the yield of uranium from polymer adsorption by a factor of 10. This effectively destroys his entire argument.
Nearly eleven assuming 6g-U/kg-absorbent is used. ;) Course, it's something of a non issue since the aim was to minimize cost (at around $150/lb), and if we're looking to minimize the volume needed we can probably construct something with greater absorption at greater cost per lb of Uranium.
My persistently unanswered question.
'Gail the Actuary' clearly shares Ugo Bardi's optimism that uranium's apparently extreme abundance is not real.
By pretending that this is pessimism, I suppose they both justify a lack of conservatism in their research. So there is no reference in the above article to the secular variation of the IAEA's "Red Book" reports. Two recent ones that came out two years apart showed a rate of increase of uranium reserves in that time that worked out to the thermal equivalent, if burned unenriched in CANDU reactors, of 100 million barrels of oil per day.
Also, haven't the problems in the Kazakh U mining industry worked out to 8651 tonnes U in 2008, as opposed to almost 2000 tonnes less in 2007? No, it was only 8521.
(How fire can be domesticated)
There is a reference to it, but I called it by its real name, not the Red Book. It is the he global nuclear fuel market: supply and demand 2007-2030. It is the report that supposedly has peak production in 2015. If someone will buy me a copy, I will read it myself.
My response to a very similar comment below from Advancednano was:
Kazakhstan is one area that is actually growing, but as the link you cite notes:
It is not clear to me how much of its production will filter back to the West.
The parts of my posting I have emphasized show that 'Gail the Actuary' is refuting a remark that is not exactly the one I made.
Whenever the Red Book comes out I don't buy it, but someone does, and reports the upshot: the estimated reserves. Looking only at these numbers' change over time is what enables us to compute that 100 MBOE/d of uranium reserve growth has been occurring.
(How fire can be domesticated)
Hello Gail, This is a comment similar to the one posted on Ugo’s report. He made no response.
http://www.theoildrum.com/node/4558#comment-413193
At what price does uranium become expensive?
If all our electricity was made with coal, a years supply of coal (14,200 lb) cost $218 in 2005 and is much higher now and climbing. A year’s supply of natural gas (115,000 cubic feet) cost $850 in 2005.
To make all U.S. electricity with current reactor designs, we only need 0.72 pounds / year / person.
For uranium to match the price of coal or natural gas, using current reactor technology, the uranium price would be $303 or $1,180 dollars per pound respectively.
Using breeder reactors we need 0.35 pounds / 80 year lifetime.
For uranium to match the price of coal or natural gas using breeder reactors, the uranium price would be $51,500 or $194,000 dollars per pound respectively.
The average American paid $1,100 for electricity in 2005. Uranium cost is a small fraction of what we pay for nuclear electricity, about 0.2 cents per kWh. Uranium price spikes have little effect on our bill.
These numbers come from this paper
http://coal2nuclear.com/energy_facts.htm
Reports in the 1970’s estimated the cost of extracting uranium from sea water at $1,500 to $2,000 per pound. R&D has reduced that to less than $150 per pound, of uranium.
http://www.taka.jaea.go.jp/eimr_div/j637/theme3%20sea_e.html
http://jolisfukyu.tokai-sc.jaea.go.jp/fukyu/mirai-en/2006/4_5.html
The oceans contain 4.6 billion tons of uranium, half of which is sufficient to support 10 billion people at the U.S. level for 400 years using first generation reactors and over 30,000 years with breeders. In reality the oceans are continuously supplied with uranium by the erosion of land, so the uranium supply is effectively unlimited.
We do not need breeders for a long time but we should move forward with breeder R&D to reduce mining and waste volumes.
Why are there no sea water uranium extraction plants?
Historically price has been under $60 / pound with a few big spikes.
http://www.uxc.com/review/uxc_g_hist-price.html
http://www.uxc.com/review/uxc_g_2yr-price.html
U3O8 is 85% uranium by weight.
Would you bet your life savings on uranium staying above $150 / lb? I don’t think so, and neither do professional investors, however if sea water technology keeps improving the cost may drop enough to make it happen sooner than most people think.
Sea water uranium is very important because it puts a cap of $150/pound on the maximum sustainable cost of uranium for thousands of years.
Sea water uranium does not have to supply all of our uranium in order to cap the uranium price at $150/pound. It only has to replace the percentage of land based uranium sources that cost more than $150/pound, and that percentage is zero for the foreseeable future.
The uranium supply is effectively unlimited.
For a linkable version;
http://www.theoildrum.com/node/4558#comment-413193
"Hello Gail, This is a comment similar to the one posted on Ugo’s report. He made no response.
http://www.theoildrum.com/node/4558#comment-413193
At what price does uranium become expensive?"
In short, uranium extraction cost considered (less than 130 $/kg) is equivalent to one dollar per oil barrel, with current nuclear technology (no fast breeders or thorium breeders)
Personally I'm a huge supporter of thorium breeders based on liquid fluoride reactors technology, but we have plenty of time to develop them
It's hard to compare them. If our vehicles were all electric with batteries charged by generators run by oil, coal, gas, uranium, etc, then we could compare. We can't make bags or fertiliser out of uranium, either.
Oil (coal, gas) is used for things you can't use uranium for, and vice versa. A simple comparison of energy content isn't always helpful. If all that mattered was total energy in the source, the world would be covered with solar panels and a global electricity grid already. Other things matter.
1) You don't need oil, natural gas, coal or any other fossil fuel to make nitrogen fertilizer.
2) All you need to make nitrogen fertilizer is air, water and energy
http://peakoildebunked.blogspot.com/2007/11/314-peak-oil-and-fertilizer-...
Ammonia production based on the Casale process started at Nera Montoro in 1922/23 with a capacity of 14 tonnes per day. Synthesis gas for the ammonia converter was based on hydrogen from water electrolysis and nitrogen from the air.
http://depletedcranium.com/?p=1520
Corn based plastic used at Walmart
http://www.treehugger.com/files/2005/10/walmart_to_use.php
Again, there's a difference between what's technically possible and what's likely to happen, or accomplished most easily - or else we'd have that global supergrid of solar panels with everything electrified already.
In the particular case of the Casale process, you can just look at how all the endothermic and exothermic reactions add up to see why we don't often make synthetic gas from electrolysing water and combining with atmospheric nitrogen.
Corn-based plastic isn't exactly widespread, either.
There's a difference between what's technically possible, or possible in theory on paper, and how things turn out in practice. Which is why we can't compare uranium with fossil fuels and renewables on a simple energy-out basis. There's a lot more to it all.
Sure, whats likely to happen is we'll burn some tens of trillions of tonnes of coal as synfuels.
Whats not going to happen is the entire energy industry collapsing faster than it can restructure because all the fossil fuels disapear overnight.
"It's hard to compare them."
It' s quite simply, instead. You have to compare the thermal energy value for a kg of natural uranium and a barrel of oil, no matter which uses you do of them. Indeed, 130 $ per kg of natural uranium grossly corresponds to one $ per oil barrel. Ergo, worldwide uranium resources can't stop at a cost of less than 130 $/kg and we have almost infinite uranium reserves at a cost of x10 or x20 that cost (perfectly acceptable in terms of energy and economics), even without breedres or thorium reactors.
Of course, there is a price market point which thorium breeders (maybe still today) or fast breeders (far less likely) become more economic than once-through, low eniriched uranium, LWR technology cycle
Comparing them by energy content is as meaningful as comparing them by weight or spectral signature.
I can't chuck a chunk of uranium in a car. I can't put oil in a little radiative reactor and send it to Jupiter to power a space probe.
They're different things. Just as our vast numbers of cars couldn't run without all the millions of kilometres of roads we've put in - all that oil would be useless for transport - so too uranium is useless without reactors, converting everything to electricity, and so on.
The change is non-trivial, which is what makes a simple comparison of energy content meaningless.
The energy content per dollar's worth is meaningful in understanding governments' attitudes. I seem to recall you saying something about governmental desire for gambling revenue. Oil and natgas revenue are similarly pleasant, and governments seem to dislike seeing five dollars' worth of uranium cancelling tens of natgas royalty or tax dollars.
It would be very hard to use a TWh of heat from burning oil to make even a watt-hour's worth of uranium, starting with, say, stable zirconium and barium. But a thermal TWh worth of uranium could readily drive chemical synthesis steps that would convert water and CO2 into a fifth of a thermal TWh worth of (non-fossil oil plus oxygen), or more.
(How fire can be domesticated)
Absolutely. But energy/$ varies a lot year to year, and lots of other things are important, too.
For example, when the US was more or less self-sufficient in oil, they didn't talk much about using less; now that they supply only 1/3, they're more keen on it. They're still not doing anything, but at least they're talking about it.
It depends on the country. Here Down Under we export all of those, basically we're Asia's quarry. We don't view it as either/or, we know we can sell all that stuff.
Given a more-or-less free market, uranium doesn't compete with fossil fuels, people happily consume both. The country with the biggest nuclear generation is the US, they're also the biggest oil consumer. China has heaps of coal-fired stations, but quite a lot of nuclear, and are keen to build more of both.
"They're still not doing anything"
That's not quite fair. The US has raised the automotive fuel efficiency standard (CAFE) quite a bit, and has given it's states the right to go even further.
This is highly likely to produce a very good start on large volumes of PHEV's like the Chevy Volt in the next two years.
Sorry, you're missing the point again.
My comparison is clearly between fuels for electricity production only, that means coal, natural gas and,yes, even oil (today, 20 of 80/90 bpd are consumed worldwide for electricity production, mostly in developing countries). Last summer when oil peaked at about 150 $ per barrel, uranium price was at about 2 $ per oil barrel equivalent when gas and coal, respectively, IIRC about 100 $ and 40-50 $ per oil barrel equivalent. That clearly means it' s totally meaningless to stop exploitable uranium resources (both for energy and economics reasons) at less than 130 $/kg, that means less than one $ per oil barrel equivalent (coal, gas and even oil have or had had market prices tens times higher,at least)
For transportation and heating there are surely other solutions where nuclear can help only indirectly, for example for collective transportation electrified railways (both for goods and people) or for private transportation electric and plugin hybrids; for heating needs, moreover, high efficiency electric heat pumps or cogeneration eventually from nuclear plants themself or renewable energy sources
If the proposed method really works, someone needs to show that it can be done at scale at reasonable cost, and that it can be maintained (not wear out immediately). There are a lot of what look like good ideas, that don't work well in practice.
The oceans contain 4.6 billion tons of uranium
The problem is collecting it.
http://jolisfukyu.tokai-sc.jaea.go.jp/fukyu/mirai-en/2006/4_5.html
What collection scale are you asserting will achieve the necessary amount of uranium to sustain the world's reactors?
Sustainable Energy Without the Hot Air has a pleasantly undeceiving treatment of this in which McKay figures the mentioned scale on a per-person basis.
(How fire can be domesticated)
A per-person basis is deceiving; I want to know how many miles of collector need to be suspended in the currents of the world, how they will be suspended, how much energy it takes to make them, how much energy is required to deploy/harvest/redeploy them, and how the logistics for harvesting and redeploying them will be accomplished.
If one solar cell is 20% efficient nobody questions that a million identical solar cells will be 20% efficient. Why would it wear out immediately at large scale? If specific engineering problems show up they will be resolved as they were with early steam engines, aircraft, cars, windmills etc.
I support a demonstration facility, as we are doing with demonstration solar plants that are not economically justified, but there is no evidence that it is not feasible.
Perhaps you missed this part.
Sea water uranium does not have to supply all of our uranium in order to cap the uranium price at $150/pound. It only has to replace the percentage of land based uranium sources that cost more than $150/pound, and that percentage is zero for the foreseeable future.
Why?
Personally my eyes tend to glaze over from all the big numbers thrown around. One or two zeros more or less doesn’t seem to make much of an impression, but I understand this.
If all our electricity came from coal we would burn 1.14 million pounds of coal per 80 year lifetime (13,400 lb/yr/person) and release 2.4 million pounds of CO2 plus 200,000 of pounds of toxic solid waste.
If all our electricity came from fission using today’s technology we would need 58 pounds of uranium per lifetime, of which only about 6 pounds would actually get into a reactor, resulting in about 10 pounds of spent fuel containing 5.4 ounces of fission products.
Consider this quote;
This works out to six 60 day cycles per year. At 4g per cycle that is 24g / kg adsorbent / year. At this rate we only need 41kg of adsorbent to produce 1kg of uranium per year.
25,000 yen/kg equals $238/kg, equals $108/pound.
To make all U.S. electricity with our primitive steroidal submarine reactors, we need 0.72 pounds (330gm) of uranium / year / person.
We will need only 13.6 kg (30 pounds) of membrane per person using today’s primitive reactors.
Can you think of any way that hanging 30 pounds of adsorbent in sea water could cost more than digging up 13,400 pounds of coal each year and shipping it half way across the country?
Even if these cost estimates are off by a factor of 5 or 10 it is still cheaper than natural gas.
Using breeder reactors we need 0.35 pounds (159 gm) of uranium / 80 year lifetime. To produce all our electricity from fission at the U.S. rate using seawater uranium in breeder reactors our 0.35 pounds per lifetime will cost $37.80 / lifetime, 47 cents per year. We will need only 83g (0.18 pounds) of membrane per person
In fact the technology is so good that Leeuwen did not complete his cost estimate of sea water uranium. Consider his analysis on extracting uranium from sea water, page 57 of this October 2007 report.
http://www.stormsmith.nl/report20071013/partD.pdf
In this analysis the most recent work considered was published in 2001 by Sugo on polymer adsorption of uranium from seawater.
http://npc.sarov.ru/english/digest/132004/appendix8.html
A… He ignores the more recent 2006 report, [Confirming Cost Estimations of Uranium Collection from Seawater- Assessing High Function Metal Collectors for Seawater Uranium ]
http://jolisfukyu.tokai-sc.jaea.go.jp/fukyu/mirai-en/2006/pdf/4-5.pdf
It describes the experimental results from testing improved technology. It concludes;
“The lowest cost attainable now is 25,000 yen ($280/kg) with 4g-U/kg-adsorbent used in the sea area of Okinawa, with 18 repetition uses.”
B… The latest technology uses braided rope like adsorbents that can be deployed in single strands or long loops for continuous processing. Leeuwen prefers to analyze the older technology of the 2001 study that packs the adsorbent in metal cages that are responsible for a large fraction of the cost and weight. The study describes three techniques to deploy the cages, one is much cheaper than the other two. Leeuwen discusses the most expensive option.
He assumes that the cages will be shipped to a distant reprocessing plant, running up the transportation cost and emissions. In reality the cages would be reloaded at sea, as in the crab industry.
C… By eliminating the cages and moving the extraction process onboard the adsorbent deployment ship, the transportable product mass is reduced by a factor of a few thousand making those transportation emissions negligible.
D… Leeuwen likes big numbers. He describes a single plant that would generate 1/7 of the world’s uranium consumption.
E… After beginning his analysis of Sugo 2001 he introduces a great deal of superfluous information from old reports evaluating a different technology, for example.
“Estimates of the cost of deriving uranium from seawater range between approximately $1000 and $25000 per kg uranium.”
F…He does not complete his cost estimate of the Sugo technology, but includes the authors estimate of $280 / kg in a table with the older/higher estimates saying, “The cost estimates by Sugo et al. may be low by a factor of at least 10.”
He ignores statements by the author indicating the potential for improvement, for example.
“The calculated stoichiometric chemical uranium adsorption capacity of this adsorbent is 500 g per kg adsorbent based upon the concentration of amidoxime groups”
“It is clear from experiments that metal adsorption rate increases roughly 3-fold above 10°C.”
Most new technologies improve with time and his own table, D29, shows that the estimated cost of sea water uranium has dropped an order of magnitude in 30 years.
G… He ignores the other valuable products that could be extracted along with uranium, offsetting a portion of the cost.
H… The adsorbent concentrates the uranium from 3.3 parts per billion in seawater to 4 parts per thousand in the adsorbent, a concentration factor of 1.2 million.
On page 55 Leeuwen has a flow diagram showing 5 waste streams. He writes;
“A five-stage process with an assumed yield of 80% of each stage, would have an overall yield of 33%. If each stage has a individual yield of 70%, the overall yield would be 17%. A rough estimate of the overall yield of a five-stage extraction process, excluding the first stage (adsorption from seawater), may be in the range of 20-30%.”
Any chemical process engineer who recommends a process that throws away 70%-80% of the product AFTER concentrating it by a factor of 1.2 million would be laughed out of the building, pink slip in hand. The so called waste streams would be recycled.
I… Most importantly he does not explain that at a few hundred dollars / kg the uranium cost per kWh is lower than our cheapest fossil fuel, coal. Even at 10 times Sugo’s estimate, the cost of uranium per kWh would be about the same as natural gas, but the cost trend for natural gas is going up while that for sea water uranium is going down.
J… Gen 4 reactors will reduce uranium requirements / kWh by a factor of 60-100. Gen 4 plants using sea water cooling could extract all their fuel directly from the condenser cooling water.
Leeuwen’s strategy is to create a straw man based on irrational assumptions that will result in the desired analytical conclusion, and then applying that conclusion to all possible designs, declaring them all impractical.
"30 pounds of membrane per person"
Ok, that's about 900,000,000 pounds of membrane for the US alone, not counting new reactors;
- Where would this membrane go specifically?
- How would the infrastructure for it be built?
- What is the EROEI for the method you refer to?
- What nuclear energy company is going to do this any time soon?
Money quote:
For existing capacity, maybe. New capacity is what really matters, and this depends on what current reactor technology costs. In the US, new nuclear costs of 4-8 dollars per Watt is not competitive even at zero uranium price, compared to current coal and natural gas prices, and new coal and natural gas plant costs.
Hi Cyril,
Is this taken from a presentation? Do you have a link back to the source?
The growth in cost is amazing. We are expecting such an event as we switch to lower EROEI power sources and the energy sector grows to consume more of the economy. But it could also be rampant monetary inflation. Still, you can see EROEI at work. Low EROEI nuke had the largest jump in cost, while higher EROEI sources jumped less, as expected.
Breeder Reactors have been "just around the corner" since the 1950s. I'm still waiting.
Thorium? While more realistic than the past, still no scaleable solutions.
It is often good to have things in hand before counting on them.
Fast-reactor technology is well known and proven to work. It is not a pie-in-the-sky fantasy. The Russians have been running the BN-600 continuously since the 80s. The IFR design was successfully tested and verified to be intrinsically safe in the early 90s, after which team Clinton promptly cancelled the project for reasons that had nothing to do with the science or engineering. I think it is a tad misleading to suggest this technology is not real, not ready or not possible. My understanding is that it is a case of simple economics: fast reactors are more expensive. Since fuel cost is a small fraction of the cost of operating a reactor, it just hasn't made sense financially to consider a commercial-scale reactor when U is cheap and abundant. If the technology is mandated over rad-waste concerns, or if carbon is taxed making the fossil competition way more expensive than nuclear, or if we really run into U supply problems, then I'm sure they'll start getting built.
Even if we start building, there are a huge number of reactors already in operation, needing fuel.
Since fast reactor technology is relatively untested in real-life situations, I would expect that phase in would be pretty slow--at best two or three fast-reactors in place in 10 years. It would be difficult to get initial approval. Until two or three were up and running trouble free for several years, I expect it would be difficult for regulators/local citizens/stockholders to believe that this was the appropriate step to take, especially if they are significantly more expensive.
Jeff Vail ran a post talking about the time lag between price spices in oil, and the adjustments necessary to change supply. The situation is even worse with uranium, in my view.
Regulators and public opinion are the biggest factors affecting the speed of nuclear innovation and deployment, IMHO. When we have a genuine crisis, however, the role they will play in throttling the rate of implementation of any new developments could change dramatically.
As Yogi Berra once said, "Its difficult to make predictions, especially about the future."
And the future of fast reactors falls into that same category. How many NRC-approved designs are there? How long before any are even submitted to the NRC, after the lengthy pilot design/build/operate phase? How long then before any are approved, given the poor track record of the nuclear industry in responding to design shortcomings this century. How long then before factories that could build the components are up and running? And then how long before the first such reactor could be fully commissioned? I'd be very surprised if a commercial fast reactor could be up and running before 2025 in even the most optimistic scenario.
Could they be a part of the overall solution mix? Perhaps but as Yogi Berra once said, "Its difficult to make predictions, especially about the future."
Good article, Gail; it takes courage to speak plainly about this subject. I don't doubt that there can be innovations, though most of what I hear is rumour or advocacy positions.
I found myself looking through a huge stack of material to put together this post. I probably had enough information to put together 10 posts. The next trick was to try to pull out some important pieces, and try to put them into an article. If there is a place where all of these things are digested and put together, I didn't find it. If you look at the links, most are to original reports or to financial statements of companies in the nuclear industry.
I think the targeted time frame from GenIV reactor roll-out is 2025-2030, which is probably based on BAU assumptions on how things get done. Rather than shrug our shoulders and say "that's how it is," I think we need aggressive action on the regulatory side to permit much more innovation and entrepreneurialism in the nuclear space. For example, I think opening up the door to factory-built modular micro-reactors (size < 100MWt) suitable for electricity, industrial process heat, home district heating, propulsion, etc. could be a game changer in terms of innovation and speed of roll-out. I'm thinking, for example, the PBMR, molten salt reactors (Thorium or otherwise), the Toshiba 4s, Hyperion Uranium-Hydride "nuclear batteries", etc. The regulatory landscape is currently hostile to such innovation and that needs to change!
All dependent too on financial conditions - which are unlikely to "correct" for a long time. There won't be any money to invest in Gen IV, fast breeder role out, fusion or anything else. The human race will need to move into its new no-growth reality long before these nuclear issues are resolved.
The other non-fuel limiting resource is the human capital that needs to be developed before a major expansion of the nuclear power industry is possible. Assuming that fast breeder reactors and nuclear batteries are theoretically possible, it will take an army of nuclear physicists, engineers, techncicians, operators,supervisors,government regulators, managers, investment bankers and educators all working towards the same goal to create a viable commercial industry.
That will take political will firstly to overcome the very real misgivings that most people have about nuclear power and its potentially deadly offshoot into weaponry, and secondly to actually invest in teh educationa and training of the necessary numbers of people required.
My guess is that we are at least 10 years away from even getting to the point where you reach critical mass with the politics of the new and improved "safe" nuclear reactors. Add another 10-15 to develop the critical mass of knowledge and enough pilot and demonstration plants to prove things up and another 10-20 to roll out the large scale commercial power plants (distributed or not) before you see any real return on the investment.
This is a 50 year plan at least, that few individuals or governments have much experiencce with embarking upon, given that the benefits will only be realised by a future and as yet unborn generation, long after the current generation of rulers is dead.
I know that there was an article in Drumbeat recently about the lack of trained nuclear engineers, if we are to build new power plants. Most of the folks who worked on the first round are retired now.
Given the treatment of waste and the general safety record of the first round, their retirement isn't necessarily a bad thing. A second generation might be a bit more careful.
I don't think this will be as big of a problem as you do.
If we had the Manhattan project we should have, we could build prototypes of an LFTR and IFR within 4 years.
Exactly. Do you see much talk by TPTB about another Manhattan Project? Obamas has just barely gotten teh energy debate started, let alone developed enough momentum in his argument to get another trillion or so for a new large scale nuclear development project. It may take the best part of his first term to articulate the argument, then he will have to win the election. Maybe in his second term he might be able to get some funding from congress to get a management committee together.
As for prototyping in 4 years, it amy be possible, but that is a long way from creating a commercially viable industry based on this new technology, given the constrainsts of human and finacial capital required.
I am not arguing against exploring new nuclear technologies, just injecting some of the realities that would apply to any new energy industry based on yet to be proven technology.
It would be considerably smaller than the GM/Chrysler bailout, and have much more impact. Given the amount of information that's already in hand about alloys, practices, etc. I doubt that the money would be a big problem; what we need right now is a sense of urgency, to skip all the roadblocks thrown up by the opponents and get some proof-of-concept into operation.
It will be interesting to see what Chu and Obama do with energy and peak oil. I am sure the administration is aware of the problem, but the political calculus has to be "what do we do, and what can we get through congress." Imagine you're the president, and you are standing in a press conference and announce the problems associated with peak oil. The press then all jumps on you with questions of how are you going to afford your solution, how can it be done in time, and what will you do about wall street, because as you speak, the stock market will crash. I am not sure what their decision will be, but its a little like juggling chain saws with cobras tied to their handles.
I believe quite the opposite. While people can stop a large fraction of their driving literally overnight and create huge variations in the profitability of oil, the construction of a nuclear reactor implies a steady demand for fuel for as much as 60 years; this certainty of demand makes the suppliers very secure. If you don't think that contracts haven't been let and project schedules worked out to determine when uranium has to be delivered for fabrication, back to enrichment, back to yellowcake, I wonder how you think major building efforts get done.
As I said, I'm still waiting------
http://nextbigfuture.com/2008/09/breeder-reactors-uranium-from-phosphate...
Japan's monju 300MW breeder is close to re-opening
http://www.physorg.com/news151221075.html
The BN-800 fast neutron reactor being built by OKBM at Beloyarsk is designed to supersede the BN-600 unit there and utilise MOX fuel with both reactor-grade and weapons plutonium. Further BN-800 units are planned and a BN-1800 is being designed for operation from 2020. This represents a technological advantage for Russia and has significant export or collaborative potential with Japan.
The BREST lead-cooled fast reactor (Bystry Reaktor so Svintsovym Teplonositelem) is another innovation, from NIKIET, with the first unit being proposed for Beloyarsk-5
In 2008 Rosatom and Russian Machines Co put together a joint venture to build a prototype 100 MWe SVBR reactor. SVBR is modular lead-bismuth cooled fast neutron reactor from OKB Gidropress. If built in clusters of 10 to 16 units it is claimed to be competitive with VVER types.
Rosatom's long-term strategy up to 2050 involves moving to inherently safe nuclear plants using fast reactors with a closed fuel cycle and MOX fuel. Fossil fuels for power generation to be largely phased out. Starting 2020-25 it is envisaged that fast neutron reactors will play an increasing role in Russia, and an optimistic scenario has expansion to 90 GWe nuclear capacity by 2050.
Russia's uranium production plans
http://en.wikipedia.org/wiki/Fast_neutron_reactor
Currently operating
Phénix, 1973, France, 233 MWe, restarted 2003 for experiments on transmutation of nuclear waste, scheduled end of life 2014
Jōyō (常陽?), 1977-1997, 2003-, Japan
BN-600, 1981, Russia, 600 MWe, scheduled end of life 2010
FBTR, 1985, India, 10.5 MWt
Under construction
Monju reactor, 300MWe, in Japan. was closed in 1995 following a serious sodium leak and fire. It is expected to reopen in 2009
PFBR, Kalpakkam, India, 500 MWe. Planned to open 2010
China Experimental Fast Reactor, 65 MWt, planned 2009
BN-800, Russia, planned operation in 2012
In design phase
BN-1800, Russia, build starting in 2012, operation in 2018-2020[5]
Toshiba 4S being developed in Japan and is planned to be shipped to Galena, Alaska (USA) in 2012 (see Galena Nuclear Power Plant)
KALIMER, 600 MWe, South Korea, projected 2030[6]
Generation IV reactor US-proposed international effort, after 2030
Gas-cooled fast reactor
Sodium-cooled fast reactor
Lead-cooled fast reactor
JSFR, Japan, project for a 1500 MWe reactor begin in 1998->2010
India is kicking their breeder program into higher gear. Starting two new breeders and have plans for 6 by 2020.
http://nextbigfuture.com/2009/02/india-major-fast-breeder-program.html
India first larger 500MW breeder will be done 2010.
http://timesofindia.indiatimes.com/India/Indias_first_fast_breeder_react...
Russia continues to finish the 800MW breeder for 2012. China is ordering one of Russia's 800MW breeders.
Uranium from Phosphate
Old and new uranium from phosphate mines are starting up or being restarted. Uranium from phosphate is not included in the current uranium reserve estimate of 5.5 million tons at $80/kg or less and could add another 22 million tons of Uranium. Reserves estimates are still increasing and exploration spending is less than one billion dollars per year.
http://uk.reuters.com/article/rbssIndustryMaterialsUtilitiesNews/idUKN19...
Kazakhstan has 15% of the world's uranium resources and an expanding mining sector, aiming for 15,000 tU annual production by 2010 and 30,000 tonnes by 2018.
http://www.world-nuclear.org/info/inf89.html
KazAtomProm announced that Kazakhstan's uranium production increased 28% in 2008 to 8521 tonnes. Plans call for uranium production to reach 11,900 tonnes in 2009.
New 1.2 million tonne per year Canadian-built sulfuric acid plant feeding from the Kazakhmys copper smelter in Balkhash started production at the end of June 2008, financed by a European Bank for Reconstruction and Development (EBRD) loan to abate sulfur dioxide emissions from copper smelting. The start-up of the sulfuric acid plant helped reduce a national shortage of the acid, which is used in large quantities at Kazakhstan's in-situ leach (ISL) uranium mines due to relatively high levels of carbonate in the orebodies. Additional acid plants are planned in the country, which will help meet future demand.
The company announced in 2008 that it aims to supply 30% of the world uranium by 2015.
http://www.world-nuclear-news.org/newsarticle.aspx?id=24343
Uranium mining forecast to 2020
http://nextbigfuture.com/2008/09/uranium-mining-forecast-to-2020.html
Anticipated New Mines
2007
China Qinlong 100tU/year
Kazakhstan Appack LLP-West Mynkuduk 1,000 tU/year in 2010
Kazakhstan Karatau LLP-Budennovskoye 1,000 tU/year in 2009
South Africa Uranium One - Dominium & Rietkuil, 1,460 tU/year in 2010
2008
Australia Honeymoon 340 tU/year
Kazakhstan Semizbai 500 tU/year
Kazakhstan Kharasan-1 3,000 tU/year in 2010
Kazakhstan Southern Inkai, 1,000 tU/year
Kazakhstan Irkol 750 tU/year
Kazakhstan Kharasan, 2,000 tU/year in 2014
Kazakhstan Akbastau LLP-Budennovskoye 3,000 tU/year
Namibia Trekkopje 1,600 tU/year
Russia Khiagde 1,000 tU/year, 2000 in 2015
2009
Iran Saghand 50 tU/year
Malawi Kayelekera 1,270 tU/year
Namibia Valencia 1,000 tU/year
2010
Canada Midwest, Sask 2,300 tU/year
India Tummalapalle 220 tU/year
Russia Gornoe 600 tU/year
2011
Brazil Itataia 600 tU/year
Canada Cigar Lake 6,900 tU/year
India Mohuldih 30 tU/year
Niger Imouren 5,000 tU/year
Niger Azelik 700 tU/year
Russia Olov 600 tU/year
2012
India Lambapur 130 tU/year
India Killeng 340 tU/year
Russia Elkon 5,000 tU/year
I haven't gone through your list, but the Canada Cigar Lake 6,900 tU/Year in 2011 is clearly wrong, as I said in my post. The company has withdrawn the 2011 date, and not issued a new later date.
Kazakhstan is one area that is actually growing, but as the link you cite notes:
It is not clear to me how much of its production will filter back to the West.
Yes, a delay in one of the mines. Just as there are occasionally delays with oil mines etc... Big projects. Crap and delays happen.
But the resource is still there (150,000 tons) and they will get it.
It is not the only project out there.
Bunch of other mines in Africa too.
the money discussions on extending the HEU downblend program
http://belfercenter.ksg.harvard.edu/files/inmm-expanded-blend-down-incen...
http://www.world-nuclear.org/info/inf13.html
Highly-enriched uranium in US and Russian weapons and other military stockpiles amounts to about 2000 tonnes, equivalent to about twelve times annual world mine production. World stockpiles of weapons-grade plutonium are reported to be some 260 tonnes, which if used in mixed oxide fuel in conventional reactors would be equivalent to a little over one year's world uranium production. Military plutonium can blended with uranium oxide to form mixed oxide (MOX) fuel.
If there was a supply gap crisis, supply can be obtained it is a matter of price.
Thanks! I have discovered that there is a huge amount of "stuff" out there to read.
If the market can continue to buy recycled bomb material in adequate quantity, that will avert (or put off) a crisis. The catch is the need to keep uranium prices high enough, and incentives for research high enough, that new mines will be developed and better methods will be discovered, before we absolutely require them, so that there is not a "step function" when we have to suddenly switch from a subsidized method to an unsubsidized method.
I wonder what the impact will be of all of the financial disruption. I see this as a big "known unknown".
One way to slowly ramp up the uranium commodity market would be to throttle back weapons-fuel conversion, which has discouraged prospecting/mining for some time now.
But the price of nuclear electricity is relatively uncoupled from the cost of uranium ore; I believe the entire fuel cycle is around 10% of costs. I'm sure you're aware of this, and assume everyone knows this, which is why it wasn't mentioned in the article, but it dampens any alarmism as there's alot of room for the price to go up/new prospecting before it affects the economics of a reactor much.
It's not like natural gas, which here in TX is producing most of the electricity I'm using to type this. Perhaps the deregulated market wasn't a good idea. It's become very expensive to run my A/C in the summer. :)
I knew the uranium cost was small relative to the reactor cost.
It is not clear to me how many companies will actually be successful in building new nuclear reactors now. I know a lot of companies have applied, but I wonder whether very many will make them past the financing hurdle. As I understand it, the 2005 energy bill only helps the first few who complete the application process and are accepted. Even at that, I believe that the utilities have to arrange financing for the nuclear power plants--not so easy, when revenues are down, and it isn't easy to get loans (sell bonds).
heavy carbon pricing and lack of suitable renewable storage will probably be enough to arrange the financing of new reactors
We don't need renewable storage per say, just an integrated "smart" grid with an appropriate renewable/dispatchable mix. Course, with heavy carbon pricing we would probably end up with some nuke baseload and the rest renewables because of costs.
hightrekker wrote:
Well the ideas of factory produced small modular LFTRs has received a lot of attention on Energy from Thorium during the last year. Clusters of small, low cost, mass produced, and quickly built reactors can compete with much larger, expensive to build large reactors. Small modular reactors can be clustered to produce impressive power amounts. Do we have a viable route to scaleability? Absolutely! The paradigm includes innovative approaches to reactor siting, including recycling old power plant sites, under ground siting, and under water siting. Can such ideas contribute to scaleability? Sure they can. Has anyone yet to raise a plausible objection to these ideas? Nope. People would rather stand around and complain that there are no scaleability solutions, rather than acknowledge the scaleability solutions already in hand.
The BBC did an interesting documentary on fusion, yes I do know its different from fission the link's here. Neither fusion or fission appear to have any time horizon that appears useful. Maybe fission can give humanity more time, but we always seem to be leaving future generations something to clean up.
Gail's posts are all about analysing the Cornucopian paradigm so many have. For me, I really glad she's doing that.
Humankind cannot bear very much reality. ~T.S. Eliot
There are also some interesting reports out about coal recently, saying that there is likely a lot less than what we have been told. Rembrandt is working on a post about these, I believe. For those interested in some links, here are a few:
http://pubs.usgs.gov/of/2008/1202/pdf/ofr2008-1202.pdf
http://www.cleanenergyaction.org/documents/press/our%20news/coal_supply_...
http://www.cleanenergyaction.org/documents/coal_supply_constraints/Coal%...
http://www.altenergystocks.com/archives/2009/02/life_after_coal_its_soon...
Even more reason to focus on demand side / efficiency measures, its much cheaper to add 'negawatts' and there is no supply line or fuel costs to worry about.
negawatts is nice for reducing co2 in the comfortable first world, but does little to provide energy to bring the other half of the world out of poverty.
Sure it can. Solar cookers, led lighting, and so on, are incredibly cheap for the developed world to churn out and can cut energy consumption, pollution, and so on dramatically in many places.
That doesn't really meet the definition of "negawatt". While the replacement of LPG with solar/biogas is desirable, it often gives people more energy than they had before (albeit from different sources).
Doh! I suppose the appropriate term would be negajoule. I don't think utilizing more energy in general disqualifies something from being a negajoule, as long as it results in a drop in conventional energy consumption.
Gail, why did you stop at $130/kg? Whatever price per kg you consider a reasonable limit on economical recovery, presumably it is based on the fraction that the fuel cost is of the cost of a kWh? Deffeyes [Beyond Oil] says that uranium is distributed in a fairly regular pyramid, with each lower step in concentration being present in vastly greater amounts. Perhaps uranium will be economical and have a decent eroei at $1300/kg?
These aren't my numbers, they are the numbers in the particular paper I quoted. They don't go above $130/kg.
Manfred Lenzen wrote a paper called "Life Cycle Analysis and Green House Gas Emissions of Nuclear Energy: A Review". This is the paper Oil Drum staff are referring to when they talk about an EROEI of 6 for nuclear energy. It is my understanding that this is computed by dividing the expected life of the reactor by the payback period (Table 18, last column). The EROEI is very sensitive to the quality of the ore. At an ore quality of .01%, Table 18 indicates the payback period would be a 14.1 years. With this payback period, we would be talking about an EROEI of 2 to 3, depending on whether the life expectancy of the reactor is 28 years or 42 years. I would expect that uranium that costs $1300/kg would be equivalent to this ore quality, or worse, so its EROEI would be 3 or less. The model does not seem to consider pollution costs.
They're lying, most likely by using Storm Van Leeuwen's model.
See the energy consumption and uranium output of the Rössing mine. At 300 ppm ore-grade it has an EROI of ~100(no 'credits' for co-mining or other BS). Enrichment, disposal and plant construction will drag total EROI down below 100, but once you've got pure yellowcake the ore-grade it came from is no longer relevant to the energy consumption of the rest of the process.
Storm Van Douchebag's model predicts that the Rössing mine will consume more energy than the entire country of Namibia. He's also fond of considering only gaseous diffusion enrichment plants running on the coal heavy US grid; rather than the order of magnitude more efficient gaseous centrifuges that represent a clear majority of the worlds enrichment capabillity. Even more efficient MLIS and AVLIS systems are being developed for enrichment. He also won't consider Insitu Leach Mining.
You may also want to look at the EPD for Forsmark and Ringhals.
http://www.environdec.com/reg/021/dokument/EPDforsmark2007.pdf
http://www.environdec.com/reg/026/dokument/EPD-ringhals2007.pdf
They use uranium from Rössing and some mine in Australia with similarly low ore grade(Olympic dam?). The full LCA for everything is included; feel free to crunch the numbers and see what the EROI is(hint, energy payback time is measured in months).
It always helps to actually read the paper before accusing anyone of lying, or assuming they're using someone else's data. Lenzen specifically rejects most of the paper you refer to.
Read articles before dismissing them. Or you could just go around writing "you poopyhead, me smart" which is after all what your post boils down to.
The one where they actually measure the energy cost of the mine, indeed the entire country of Namibia?
You guys are nuts.
Very interesting paper Gail. It is a publication of the results of a study commissioned by the last Australian Prime Minister from the University of Sydney. It has data for the Rossing mine in Nambia. Here is the graph from the paper.
There are some outliers. What is interesting is that in the insitu leaching is not as much lower on energy use as one would expect, which is disappointing. And that the Australian mines fall pretty much on the line. Rossing in not that much lower than the line. The paper notes that the SvL results were a summery of 39 other studies of uranium mining. So it is not surprising that a few more studies fell mostly into line.
I find this paper very good. It does a nice job of comparing many studies. It uses SvL results when they match up with other studies (mining and milling) and rejects them when they don't (reactor construction and decommissioning energy costs). It has an excellent sensitivity analysis section where they pose many variables such as operating up time, and types of enrichment, ore yield, etc. The formula are given to calculate the energy return using any set of conditions you would like to assume. This should be very helpful for modelers who would like to see what happens as say: centrifuge enrichment is put into place to balance a drop in ore grades.
The conclusion is important. A 6 to 1 EROI will support a negligable growth rate. Nothing even close to the rate needed to replace fossil fuels. Lovelock is totally wrong in backing this technology. It will never scale to solve our problems fast enough.
http://me.queensu.ca/people/pearce/publications/documents/as15.pdf
This strongly supports Alan's long time claim that we should rush wind development and let nuke construction move slowly. If another reactor type should be invented with a much lower EROI then it may be possible. But while we wait on that development, the push to an electrical economy should be done via wind and hydro.
Regarding the relative energy use of ISL, it seems to me that the ISL mines are in general smaller. Tis might affect efficiency. They also need quite a bit of water. I believe that this can be piped in from a distance, or obtained through desalination. I would expect that either could be expensive/energy intensive.
I am guessing that their operating life is shorter, but I don't have any data on this. If it is, the shorter start-up costs are amortized over a shorter life-span.
The issue with the in-situ leaching is mainly the chemicals involved. They use vast amounts to bring the uranium out of the rock, and more vast amounts to make the uranium precipitate out, then yet more vast amounts to take the tailings from the process and turn from extraordinarily toxic to merely toxic. And all those chemicals take energy and resources to make...
Also the tailings ponds have to be maintained, which involves a lot of work by construction vehicles and the like. For example, Australia's Olmpic Dam had its tailings pond leak up to 5 million m3 of uranium and copper and chemicals into the aquifer from 1992-94.
Jon,
Using the default values, it appears that 203 tonnes of natural Uranium produce 8600 GWh of electricity, 43GWh/tonne.
From the graph of mining energy 3,000Gj/tonne is a ball park for low grade ore, so 1GW energy to produce 1tonne uranium(3.6 Million j=1Kwh,3.6Gj=1GWh).
So as far as ore mining goes, ERoEI is >50:1
Using the Swedish study referred below by Soylent, it has lifecycle of 4g CO2 per kWh, based on coal generation. Since 1kWh generates 1Kg CO2 in a coal fired power plant, that gives a EROEI of 250:1. Even allowing for a much lower ore grade(3,000Gj) where most energy use is from extracting uranium from the ore, we still have a lifecycle energy use of >40:1.
Not sure where you get 6:1? higher inputs from non-mining?
Most likely from assuming the use of cold-war era gaseous diffusion plants for enrichment. They consume some ~50 times more energy per SWU.
No new enrichment plants of the gaseous diffusion type will be built. The majority of enrichment plants are already of the gaseous centrifuge type.
AVLIS or MLIS can potentially reduce the energy consumption and cost of enrichment further as well as making enrichment practical for other causes(e.g. isotopically pure silicon, if silicon is still relevant for semiconductor technology in the future).
I recommend reading the paper, then you don't have to guess. They assume mostly centrifuge enrichment. Data is provided on laser based enrichment. The paper has a substantial number of references.
Hi Neil,
Yes, it is other non-mining inputs. Like reactor construction etc. I do recommend reading the whole paper, it is quite interesting. Here is the link again (Gail has it up top).
There are a number of interesting ideas. On the mining side, the Olympic Dam mine cuts energy costs by also mining copper. Those kinds of efforts could slow the rise in energy use as ore quality declines. The Rossing mine has a much lower energy use for its claimed ore grade. It would be worth a more in depth study to find out why it is doing much better than other state of the art Australian facilities.
I will have to look at Soylent's link. CO2 per kWh tends to vary quite a bit based on grid input assumptions etc. In depth studies of coal plants put the EROEI in the 10:1 area with coal itself in the 80:1. (See Dr. Hall's EROEI studies posted by Nate Hagens).
Jon,
Thanks, the link you gave was for the Pearce paper.
Also more detailed report by Lenzen ;
http://www.isa.org.usyd.edu.au/publications/documents/ISA_Nuclear_Report...
The Olympic dam data point is for copper 1.6% not the uranium. Olympic dam is fairly deep( <300) meters and underground so energy costs are higher. Also note that acid leach is considerably lower in energy costs. This is true for acid leach of other ores such as copper where 0.3% copper ores are extracted(if close to surface).
The Lenzen report summaries other studies and derives a 6 year payback from 0.18kWh energy equivalent/kWh generated based on a 35 year life of a nuclear plant. Since about half the energy is reactor construction and decommissioning, would expect a higher EROEI for a 60year reactor life.
Probably more important is the 60g CO2/kWh(lifecycle)of nuclear power, compared with coal fired electricity of 800-1100g/kWh that nuclear would replace, ie a 95% reduction in CO2 emissions.
I've got that document open on my machine but haven't had time to take it apart yet (working on another piece ATM). However, I can tell you right away that it doesn't add up. A nuclear plant requires less concrete and steel per average kW than a wind farm. As we've seen elsewhere in this comment thread, the EROI of the fuel cycle is on the order of 50 even for LWRs. Given this, how can nuclear have a lower total EROI than wind? You don't even have to put in the energy for more than 1 fuel load before it begins operation and the rest is spread over as much as 60 years, while the wind farm is 100% up front.
Something is very fishy about these claims, and sooner or later the news will come out.
People tend to be very surprised when they discover this; wind and nuclear use about the same amount of steel and concrete per MWe when you take into account average capacity factors. (large, e.g. 3MW wind turbines, and LWRs). They imagine turbines as dandelions blowing casually in the wind, without thinking about how much foundation is necessary to anchor a 100m tall structure.
I don't have a bill of materials for a nuclear plant to compare with a BOM for a wind farm (if you do, please send them over!). But I have looked over the work Dr. Hall did on a coal plant and so I can make some guesses.
First, the complexity of the work for a nuke plant is much higher than a wind turbine. It is one thing to put down a square concrete pad dug into the ground (even if it is large). It is another to form a containment dome that will survive an aircraft impact or a burning reactor core. I would also guess the quality of steel is lower in a tower than in the complex piping that carries the coolant and steam. There is also the complexity of internal electronics and sensors. Steam turbines have a much higher energy use per ton than plain steel, and I would guess that steam turbines have a much higher energy use than the relatively simple gearboxes and generators in a wind turbine.
Again, these are guesses. Without actually having two studies which list every major component side by side then you can't really know. In these days of electronic publishing, I find it frustrating that so many papers are so short. You almost always get a summery, and not the line items.
http://www.windpower.org/en/tour/wtrb/comp/index.htm
Wind turbine supply chain.
8,000: the number of components in a modern wind turbine, ranging from steel towers and high-tech composites for blades, to gearboxes, bearings, electrical wiring, power electronics, and more;
http://www.industryweek.com/articles/wind_turbine_supply_chain_spinning_...
Floating offshore wind structures
http://renewableenergyactions.blogspot.com/2008/08/overview-of-floating-...
Offshore wind turbine modelling
http://www.marineitech.com/downloads/OMAE2006-92029.pdf
Life cycle assessment of floating offshore wind turbines
http://www.sciencedirect.com/science?_ob=ArticleURL&_udi=B6V4S-4ST45R5-1...
Steel and concrete per MWe
http://nextbigfuture.com/2008/07/per-peterson-information-on-steel-and.html
Nuclear reactor components
http://en.wikipedia.org/wiki/Category:Nuclear_power_plant_components
Many different reactors
http://www.nrc.gov/reactors/new-reactors/design-cert/ap1000.html
China's high temperature reactor
http://nextbigfuture.com/2009/01/chinese-researcher-2007-presentation-on...
The lifetimes of coal, gas and wind are too low - most thus far built have lasted longer - and that for nuclear unprovably high (there are no 60 year old reactors still in operation, though some built in the 1970s may still be around in the 2030s).
As others have said, though, the real issue is design complexity. Semi-literate African peasant boys have built their own wind turbines, but even modern Britain needs French help to build nuclear reactors.
Advanced,
The offshore wind modeling gives 100Tonnes/MW for the two examples.
Larger on land turbines (5MW) have a weight of 450 tonnes and similar if steel tower, so 200Tonnes steel/MW capacity. Less steel if concrete towers, 100-200 Tonnes reinforcing for a 5MWc.
Perhaps this is talking about Tonnes/MWa output, ie correcting for capacity factor?
Thanks Nano!
Here is another paper by Lenzen that looks at wind. It examines quite a few turbines. There is quite a bit of variation due to assumptions about recycled steel, capacity factor, size of turbine etc. He comes out with an average of 16:1 EROEI.
Energy and CO2 Analysis of Wind Turbines
He also makes some comments at the end about how annoying it is that other studies don't use common boundaries etc when doing analysis (it sounds like the TOD forum a bit. Lol). (If you don't want to buy the article I recommend visiting your local university library where they will often let you down load them for free).
If he's referenceing stormsmith at all, he's either an idiot or has an axe to grind. When Rossing has an energy return on mining alone of 500 on a low ore grade hard rock mine, the question of ore grades is just meaningless.
Jon,
This graph you have posted seems to have some very big errors. Olympic dam uranium ore grades mined are 0.06%, not 2% shown on graph. They produce 160,000 tonnes of copper, and 4,000 t of uranium so if uranium was 2% in ore would be mining 80%copper!! actually grade is 1.5%copper
http://www.mining-technology.com/projects/olympic/
I think they are underestimating the energy used to mine the uranium because most of value is for copper and gold.
Do you have the reference for the Uni of Sydney study?
Yes, I do
I believe they use the copper ore grade and then factor in the added uranium extraction cost. There are extensive notes. It looks like they use BHP Billiton's calculation. If you dig into it further and learn anything, please send me an email as I would like to hear about it. Similarly if you learn anything about Rossing. Thanks!
Jon,
Thanks, I didn't see this before I replied further up thread.
The Olympic dam situation is important because it contains 400,000 tonnes of proven reserves. As I said earlier to Gail, none of these reserves would have been proven if it wasn't for the copper, because the deposit is deep. Even if they had started mining the uranium they would never have drilled out such a large resource based on today's uranium demand. If future prices rise enough, lots more Olympic dam size deposits will be drilled out and proven and inferred reserves will increase
The Beverly mine is new and only for uranium so energy costs are probably more realistic ( and low) for new mines where in-situ acid leach can be used.
The computer guru John McCarthy parlayed the Bernard Cohen article into a wild claim regarding the sustainibility of nuclear
power.
http://www-formal.stanford.edu/jmc/frames.html
Bernard Cohen has also researched risk, radon and radiation hormesis. A few years back I visited him at his emeritus Pittsburgh office and talked him into writing an article for the American J. of Roentgenology, one of the two major radiology journals.
http://www.phyast.pitt.edu/%7Eblc/Cancer_risk.pdf
And when the fission industry feels they are safe enough to not need the blanket protection of Price-Anderson, then let them build their reactors a-plenty.
When the fission industry can operate without fines from the feds over safety violations, let that model of correctness be then taught to the rest of industry.
(Go ahead - produce a copy of the testimony of fission industry reps asking for Price-Anderson to not be renewed as they no longer need it because the industry is just too gosh darn safe.)
what is the death rate per TWy from LWRs? what is the death rate per TWy from wind or hydro?
Here are the deaths per TWh stats. Multiply by 8760 to get deaths per TW year.
However, note that solar only has about 15 TW hours. Wind is at 200 TW hours. Nuclear worldwide is at 2600 TW hours. Only coal gets up to about one TW year.
Deaths per TWH from european stats, exTernE (swiss) study for Europe
http://nextbigfuture.com/2008/03/deaths-per-twh-for-all-energy-sources.html
wind power death rate
http://www.wind-works.org/articles/BreathLife.html
Wind death rates in the UK. Mostly falls from the 30-60 story tall turbines.
http://www.thenewamerican.com/tech-mainmenu-30/energy/788
Refers to the Caithness wind farm stats
http://www.caithnesswindfarms.co.uk/accidents.pdf
the question is designed to elicit exactly that externe reference; people refuse to believe that nuclear fatalities per TWh are just about the lowest of any source. How could it be the case, when nuclear is the dangerous monster waiting to take your children away?
What people believe is important. I happen to believe in democracy. If people don't want nuclear, or coal, or wind, or whatever, they shouldn't have it. On the other hand, they have to have something in their backyards, or else accept that they'll have no electricity. That's why I put the poll on my blog.
So far coal, oil and natural gas are the least popular, 11 of 147 have voted "None" and 13 of 147 for nuclear. So it seems that some people would rather have no electricity at all, than electricity from nuclear or fossil fuels. Given that they're voting online using electrical equipment, it's an open question whether they'd still have that opinion after a week or so without electricity, but...
The well-known renewables are the clear winners. Of course, probably lots of people just don't know about tidal, for example - but that's why I gave a year for the voting and let people change their votes until then.
If people don't want nuclear, or wind, or fossil fuels, we shouldn't have them. That's why I'm always puzzled when pro-nukers say, "only politics have stopped a wide expansion." That's like saying "only politics have stopped communists taking power in the USA." It's true, and it simultaneously states and misses the point - if people don't want it, they shouldn't have it. That's democracy.
You should add magic pixie dust to you're little poll too.
I'm basing my poll on reality, not on the latest story about how technology X will allow us to keep on truckin' and scoffing down burgers forever and ever, amen, well when we get it to work 30 years from now, anyway.
I offer only commercially-proven options; whether they are good or bad in non-commercial ways is something the people ought to be able to decide.
I don't believe that using 'peaks' to illustrate fuel depletion
is helpful.
Logically if you are past peak you know that it makes no sense to build more refineries, power plants, etc. because the resource is decreasing.
For example, if your IEA uranium graph is correct normal supplies of uranium will decline in 17 years if demand grows as expected, that's not a lot of time. As an alternative we could look at our present rate of consumption and cap it--no more nuke plants and instead we have power for the next 80 years or so years; 1941000/~25000(once Cold War stocks are all gone) instead of 50 or so.
As far as thorium goes, it is bred in reactors which are stoked with plutonium or natural U-235. U-233 breeds slowly and burns fast so there is a natural bottleneck. This is why they need FAST breeder reactors(inherently dangerous). When you breed fuel, you must reprocess the fuel batch to extract maybe 1% of 'new fuel' while 99% of the batch is removed so the process is problematic.
I doubt that current breeding technology will produce adequate quantities of plutonium and U-233 to feed the current reactor fleet.
Theoretically something like Carlo Rubbia's energy amplifier would be much better but it would require gigantic amounts of electricity. Why no one is looking at this is surprising to me.
http://en.wikipedia.org/wiki/Energy_amplifier
http://canteach.candu.org/library/20041209.pdf
It's the same thing with oil. Oil production has peaked, the amount of conventional oil cannot grow and experience has shown that unconventional oil grows very slowly.
China has shown that coal can grow very fast but there is only enough proven coal for a couple more centuries at current rates of production and that is the very end of our high energy future.
We need to forget about all the peaks and cap the consumption of energy and reduce demand with energy taxes.
Regardless of the how sensible their action is, China, Japan and India are all building nuclear reactors. If they built nuclear fuel recycling facilities at the same time, the situation might not be too bad (but I don't understand what inputs are required for recycling facilities). If they are not aware of the problem, they will not plan ahead.
From the making-stuff-up department:
The final run of the Shippingport reactor was 5 years with a thorium-uranium core. The reactor bred slightly more fuel than it burned. This disproof of your BS is more than 30 years old now.
Fast-breeder studies are looking at burnup of upwards of 300,000 megawatt-days per ton. That's well over 20%.
Clean up your act, or your blatant disregard for truth and facts will get you banned sooner or later (probably sooner).
I don't see banning as a possibility. We all learn from each other.
Thank you, Gail.
And Engineer-Poet ought to be glad that bannings aren't more frequent.
Show me where I just Make S**t Up and don't respond to correction.
"The foolish reject what they see, not what they think; the wise reject what they think, not what they see."
-- Huang-Po
(never thought I'd be quoting that, but I am willing to admit when I'm wrong. ;-)
Thorium breed/converts best in slow neutron thermal reactors as does plutonium in LWRs where you get about 1% plutonium out with a breed ratio of .3: 3.5% x .3= 1.05%
The conversion/breed ratio for U233 is .8 so you your breeding would slow down the the need for additional fuel but wouldn't actually breed fuel for other plants.
See Table 6.1 in below.
http://tiny.cc/sl6Eb
The burn up rate for thorium reactors has historically been lower than FNR or even LWRs at 15-20000 Gwd/MTU(40000 in newer studies).
Given its limited reserves of natural uranium and its abundant supply of thorium, India has chalked out a unique three-stage nuclear program. In the first stage, pressurized heavy water reactors (PHWRs)—similar to those used in advanced industrial countries—burn natural uranium. In the second stage, fast-breeder reactors, which other countries have tried to commercialize without success, will burn plutonium derived from standard power reactors to stretch fuel efficiency. In the key third stage, on which India's long-term nuclear energy supply depends, power reactors will run on thorium and uranium-233
http://www.spectrum.ieee.org/print/6590
Here's an older study indicating that U-233 breeds at a rate of .3 grams in a PWR with 19.5%(!!) U-235 in it while plutonium breeds at .4 in an ordinary PWR with slightly enriched 4.5% U-235 in it.
As I said thorium is harder to breed even in an highly enriched environment.
http://www.princeton.edu/~globsec/publications/pdf/9_1kang.pdf
Obviously there are physical reasons why nobody is breeding a lot of U-233(see above). It makes no sense to ignore them.
Breeding thorium once U-235 is exhausted isn't possible. Thorium can gain a few years more if it is to be enriched inside reactors unless new technology such as fusion or the Rubbia Energy Amplifier comes along we can expect fission(derived from U-235) to keep us powered for more than a century IMO.
If the proponents (like EP) of breeding in fission reactors have different research they should post them.
Er, MSBR maybe? or Shippingport?
Rubbia's energy amplifier is a crock. Its essentially a rube goldberg machine to get around NRC regulations for reactors by having your reactor not be critical. It'll never ever be built because the extra cost of the accelerator will dominate. It'll never be financed because its simply a dumb design.
The reported result for Shippingport's final run is a breeding ratio of 1.01.
You seem to have a fixation on breeding systems designed and operated to make weapons-grade plutonium (short cycles, frequent reprocessing) and generalize this to breeders designed and operated to maximize energy return and minimize waste production (long cycles, high burnup). This is grossly incorrect, and if you're doing it deliberately it is just as grossly dishonest. Now that you've been informed, you can no longer claim that you are merely misinformed; it's time to clean up your act.
I posted a source to back up .8.
See Table 6.1 in below.
http://tiny.cc/sl6Eb
The 60MW Shippingport EXPERIMENT used a core of about 20% U-235 highly enriched uranium( almost weaponizable) and thorium and bred at 1.0. That's enough U-235 for 5 normal
1 GWe power plants. The EXPERIMENT ended so they could 'look at the fuel', which means the fuel needed reprocessing to remove U-232 so this doesn't amount to a true thorium cycle operation as EP claims.
Plutonium is frankly a better fuel than thorium and is used in the few MOX nuke plants plants and sunmarines,etc. Unfortunately it also produces lots of actinides.
I will be first to applaud whoever develops a practical thorium cycle powerplant as I will continue to question those who claim such a system exists when it exists nowhere on this planet.
Here's a recent (negative)assessment of thorium cycle from Finland's atom scientists. A
thorium breeder cycle is futuristic, coming online in 2050!,
but perhaps thorium can be used in some heavy water reactors.
http://www.ats-fns.fi/archive/esitys_wallenius.pdf
There are only 29 heavy water reactors in the world(17 in Canada) with 13 more coming(out of a total 439 nukes with 119 shutdown and 17 being decommissioned).
Heavy water reactors produce significant amounts of radioactive tritium.
http://www.ccnr.org/tritium_1.html
OTH, EP is a nuke cornucopian who feels threatened by people who argue fairly against his precious pipedream (that
fission power is practically infinite).
There are well known reason why there are no thorium reactors and there are no fast breeders and why people don't reprocess spent fuel, etc--and I've provided those 'inconvenient truths'.
EP has now reveal himself to be a troll(how ugly).
That also makes him a problem for TOD, which should not encourage flaming trolls.
The only thorium-based cycle in that table postulates an HTGR loaded with thorium, not one designed to have e > 1. Both molten-salt and light-water thorium cycles are conspicuously absent.
Had you checked deeper into your reference, you might have found this:
See also the sentence about the rest of the plant being essentially unchanged from the standard LWR configuration. Retrofitting existing LWRs to thorium breeders appears to be a distinct possibility, although the higher power density may cause problem with neutron capture by Pa-233.
Yet you have never been able to refute the ~1 GW(e)-year/ton energy available from fissiles. You never will, because it's a fact of physics and not subject to your political views.
I also argue that solar and wind are practically infinite. They're just starting from a much smaller base than nuclear and will take longer to ramp up.
You're practicing "tu quoque" without a license, I see.
Since you are the one quote-mining books and not citing the sections which actually deal with your claims (perhaps not even reading them, given your search terms), on top of repeating claims which are blatantly false, I think anyone with a brain can figure out who the troll is.
You mean like how uranium is so cheap that any such breeder cycles have to have obvious advantages beyond mere fuel efficiency?
If you want to argue that breeders at any premium at all are uncompetitive, I won't argue that. It's because uranium is and allways will be too cheap for breeders fuel advantage to be economic.
Don't forget the other end of the fuel cycle; breeders have a cost advantage if waste disposal is expensive, because the volume is only a few percent of what an LWR produces.
Iridium is one of the products of the decay of radwaste, so waste may actually be worth keeping around just to mine it for valuables. At upwards of $350 per troy ounce, iridium might be quite profitable.
No reprocessing is cheaper than dry cask storage.
I'm being pedantic here, but iridium is not in the spent fuel matrix. Of the platinium group metals its rhodium, ruthenium, and to a lesser degree paladium. But currently its still more expensive to get it out of LWR spent fuel than out of mines.
I have hopes for LFTR fuel cycles changing the economics of reprocessing, but I dont think that it has any future for LWR fuel unless its by political fiat as it is in France.
Dry-cask storage is only for spent LWR fuel.
I'm not expecting recovery from oxide fuel (unless it's reprocessed to feed a fast-breeder). Things like precious metals will be much easier to recover from the salts produced by pyroprocessing (IFR) or salt distillation (LFTR). You can just let the salts sit and maybe warm them up occasionally, and precious metals will fractionate by themselves; I've found items about this actually being a problem in the fuel systems of molten-salt reactors. Some time ago I read about some recovery of precious metals from Purex waste tanks going on at Hanford, though I can't find it any more (it's buried under too many items with the same keywords); I did find this news item about fractionation of the liquid wastes.
We all have limited time to spend. Do you have a recommended list of readings? It would be great if TOD had some kind of reading list by subject in priority order. That would make coming up to speed much faster and hopefully reduce the amount of disagreement. We could update it as new studies become available.
Me too.
People usually just post and respond often without sources
or reasoning.
I think you may need a new kind of comment format, maybe maybe a kind of examining committee or referee with specific questions and answers coming from an objective point of view.
What do we know? What don't we know? What questions should we be asking? It's a little bit more formal and less friendly but
maybe more productive.
Gail's article has been an excellent starting point because it simply asked 'Is Uranium peaking?', which can be debated.
Maybe you want to simply list the link references under each posters name for future reference.
If people who consider themselves knowledgeable on this subject want to list references they think others should read, that might be helpful. Also, thanks to Advancednano and others who have already posted links.
On some of these issues, I doubt that there is really consensus. Also, new things keep coming out that modify the things we thought we understood before. So it is difficult to come up with a list of recommended references that stays current for very long.
Nuclear's particularly difficult, because with the Cold War and all that "Atoms for Peace" stuff, we got oodles of research into the strangest and most obscure areas. For example, we've even had a U-233 bomb tested by the Americans (it didn't work very well).
Someone can always pull up some obscure type of reactor which was built once and run for three months before being shut down, or studied in a paper somewhere. There have even been EROEI studies on fusion designs - not very useful, given no working ones have ever been built, but there you go.
Really it's most useful to look at widespread practice. Saying, "oh but what if we all used this brilliant new super-safe reactor design" is about as useful as saying, "what if we all rode bicycles?" If we did, it'd be great - but it's not going to happen. We have to assume that we'll continue to be pretty mediocre.
Not crappy, not brilliant, just mediocre, stumbling along.
(duplicate removed)
What about something that was run for 5 years, e.g. the Shippingport thorium test? (I'm beginning to feel like a heckler at a Ford lecture, shouting "What about Nixon?" Even knowing about this brands me as an old fart.)
If Shippingport could be run on thorium, most any LWR could be run on thorium. Pulling the uranium out of spent fuel isn't particularly difficult, and who (besides the DoD) cares what makes good bombs so long as it makes power? I'd have to look into the details to be sure, but if we could use extra enrichment plus thorium to make 60-month cores instead of 24-month cores, wouldn't that change your appraisal?
(If new information wouldn't change your appaisal, it means you're an ideologue instead of rational.)
Engineer-poet,
Also studies with CANDU reactors running on thorium
http://www.iaea.org/inisnkm/nkm/aws/fnss/abstracts/abst_te_1319_4.html
Interesting that you mention CANDU, because the U-235 content of spent LWR fuel (~1.0%) is greater than that of the natural uranium used for CANDU (0.71%). This means that the entire uranium supply used for CANDU can be replaced with reclaimed uranium from LWR fuel (no enrichment required, just separate and convert to oxide). This allows the current CANDU fuel stream to be used for LWRs. It may not be much, but it's one more possible stopgap measure.
Contaminants. Actinides, etc. Spent LWR fuel is not a trivial thing to clean up for reuse.
Who cares about actinides? The CANDU would have created a bunch of them anyway. The only thing that matters is that the LWR fuel is perfectly suited to run a CANDU; indeed, given the higher U-235 content it should be able to increase burnup by about 60%.
Separation of uranium isn't all that difficult; fluorination converts it to UF6, leaving the fission products and actinides behind. This would also achieve a radical reduction in the bulk of spent nuclear fuel, which is an asset for environmental isolation and disposal.
translated:
There is no free lunch.
At least not anymore.
woolie, you are so right. "Energy" taxes using AGW or other ecological impact as the justification are grossly wrong if applied to nuclear, wind, tidal, etc. We should tax pollution and let people do as they will otherwise.
What form of energy will be used to extract and process the uranium?
IF it is oil, then linkage may produce shortages sooner.
That is a good question.
There is a lot of big movable equipment used in mines in Canada (for example, Cigar Lake) and Australia (for example, Olympic Dam), so these would seem to use a lot of oil.
I am not sure how the ISL mines work. Little dirt is moved, but they use a whole lot of water, which has to be pumped from somewhere, or desalinated. They also use sulfuric acid, which apparently has been in short supply recently, at least in Kazakhstan. I believe oxygen is also used in the process.
Pumps, crushers, conveyors, massive draglines; it's almost all are either electric now or easily electrifiable. The only parts I'm aware of that aren't easily electrifiable are giant ore trucks.
Since they don't need to go very far it ought to be possible(but non-trivial) to have a set of massive flywheels that can be recharged very quickly every few loads(it would still need a back-up engine for when people screw up or for moving from one mine to another, very slowly).
Is there a practical "all electric" process for manufacturing steel for reactor vessels (and trucks, etc) in large quantities?
if we aren't able to produce enough infrastructure to mine ore and cast steel, our society is well beyond the threshold of totally and completely fucked
Sure. You've got to presume that coal has gone well over 10 times its current price and then you use hydrogen reforming and induction furnaces, so some ten decades from now maybe.
It's not about the price of coal in dollars, it's about the environmental costs of coal.
Read the IPCC reports, read Hansen.
electricity is the highest quality energy, it can substitute for nearly anything you'd like to burn (burning? how primitive!). For transportation, even absent biofuels and their attendant issues, there are processes to capture atmospheric CO2 into liquid fuels, conveniently economic with a heat source like a high temperature reactor.
While I generally agree, its not the case in steel production. There you need to deoxidize the iron ore chemically, so you provide carbon from coal as the feedstock for deoxidation. You can also use hydrogen provided you have some additional steps to clean the hydrogen out of the final product (hydrogen embrittlement of steel sucks)
If we have no carbon feedstock, then you can use hydrogen reforming or reforming of the CO2 into CO or C for steel production at a cost. But this is at least a century into the future, as I imagine even carbon sequestration (pick your type, even mineral carbonization) is less expensive than closing the entire cycle with electricity from whatever the source.
An interesting exercise however.
A lot of the future steel production will be from re-cycled steel, old oil tankers,( actually happening today) old SUV's( US's richest mineral stockpile).
The last 10% of coal production will be able to keep new steel production going for a very long time, especially if the coal is only used to regenerate CO for reduction, not to heat.
True to a point, but irrelevant. Future steel production (not recycling) should be done with biochar and carbon-captured for atmospheric remediation. Electric arc furnaces are already the state of the art for recycling, and need little/no new carbon.
I don't see why we wouldn't just use coal. Biochar (as well as CO2 recycling to CO) is an interesting thing to contemplate when coal actually gets too expensive to use for steel production. Sometime just after never.
as is usual, I learn something new from your comments. thanks. :)
Sure, but not even wrong.
Some copper mines in Africa have electric ore trucks. (A Canadian mining company).
Many large ore mining trucks are diesel electric, and all underground ore trucks are electric, they could also operate above ground.
What about the ~30 years worth of spent fuel that hasn't been recycled in the US? That could be a short term resource while: a) new mines are brought up to speed; and, b) GevIV reactors are brought online. It should be faster to bring recycling plants online rather than to build a new generation of reactors or develop new mines. Once GenIV fast reactors and/or thorium fuel cycles are widely deployed, then we'll have a supply of fuel consisting of LWR spent fuel rods, depleted-U stocks, fresh U and Thorium (which is a much more plentiful element) that will be, for all practical purposes, limitless. I think with a complete burn (fast reactor or Thorium), you are looking at roughly 1 ton per GW-year! Compare that to the millions of tons involved for a GW-year of coal-produced power or the 100tons (IIRC) for LWR once-through fuel cycle.
It seems to me that reprocessing plants would need to be built, in order to reprocess what is now deemed to be waste. For a long time, there was a law against this in the US. I am fairly sure that I heard this was changed in the last year or so, but I don't have a reference on hand.
Knowing how things work, I would expect it would take well over five years to get reprocessing plants built. First there would need to be some kind of plan, then a site would be needed. Next, the plan would need to be modified to fit the site. A contractor would need to be found to build the site, and actually do the building. There probably would need to be some testing before the plant can actually be put to work. Along the way, someone would need to pay for all of this. Given the inability of companies to borrow money, and the debt load of the government, it is difficult to see where the funds will come from.
Gail,
Reprocessing is a scam, breeders are a sham.
Breeders cannot produce much more fuel than they consume therefore they cannot save the 400 Gwe existing nuclear power industry, so your peaking chart for natural uranium is still accurate.
A complete system of fast breeders(full actinide recycle in 2050) would still require about 60% of our current intake of natural uranium, so that would still not save us from your peaking situation.
Reprocessing would save at best 10%(in 2050) of uranium input.
http://web.mit.edu/nuclearpower/
Please read Chapter 4 to convince yourself(Fig. 4.1,4.2,4.3). This is from MIT. They aren't idiots.
Funny you should say that, cause it seems to me when MIT announces a new energy tech that could have very positive impacts, the first thing doomers tend to do is try to portray them as idiots.
Being from MIT, I just want to throw out there that just because it's from MIT doesn't mean they're telling the whole story. They do state at the beginning that their principle reason for not recommending fast reactors is political (non-proliferation), not technical.
Further, they are looking at using only uranium as an input, and are ignoring what they do in places like India, where they use the excess neutrons from the reactor to convert thorium into fissile material. This is more like the technology we would be talking about when we discuss long-term solutions because thorium is very abundant.
There are a variety of reactor designs which depart substantially from the PWR designs analysed in the MIT report you quote. Everything I have seen (although I'm not a nuclear engineer) suggests that these designs are technically feasible and will allow for long term nuclear energy.
So, while certainly the MIT folks who wrote up this study are clearly not trying to make up data, they're not being very optimistic about what types of reactors we will be using in the future, nor are they considering alternative fuels to uranium. And I suspect that is because they're trying to tell a story... a story that recommends that the correct political solution is also the correct technical solution for the near to mid-future.
Perhaps what Gail's article does, more than any specific technical point, is provide a call-to-action for nuclear adherents to plan out a future for existing and new plants.
I see on DB that Oklahoma is among other states considering a new nuke reactor. I'd rather support a new supposedly-safe thorium cycle reactor that would enable waste reprocessing from other sites (waste is probably worth more to get rid of than any fuel would be to buy!) and provide a technology step-up as well, than build another 'proven' but demonstrably obsolete design.
Of course negawatts and wind would make good sense for OK as well, but I have zero issues with pushing for multiple technologies as best we can, and spending dollars on long-term needs rather than short-term "shovel ready" projects.
Okay, you proved me wrong.
There must be idiots at MIT.
If you would bother read you would understand that LWR provide fuel for breeder plants(either plutonium or thorium) therefore if no LWR there will be no breeders.
India is doing nothing with thorium even though they would dearly like to. Instead they are buying big LWRs from Russia and leaving their small CANDUs which could conceivably use thorium as fuel, as demonstation plants.
The IEA chart for the peaking of natural uranium is probablr accurate and a crash program in nukes will only eat up natural uranium faster.
Kid, I have no problem with being wrong, and being wrong doesn't make you an idiot. That's part of being a scientist. However, what you said doesn't contradict what I posted at all. Thorium may well work, and obviously it has a decent theoretical net energy release in the transmutation cycle. I wasn't making an argument that I've analysed all the new options, and in fact explicitly stated that I'm not a nuclear engineer, to make it clear that what I'm saying shouldn't be taken as truth. Being a domineering loudmouth doesn't lend itself to productive discussions, and I know my goal from reading here is to learn about these issues.
That said, what I have seen in numerous presentations on fission reactor design is that currently we enrich uranium to ~5%, and then when it's depleted, the remainder is scrapped. However, by changing the way the fuel and moderator are designed, we can convert the U238 or thorium to plutonium or U233 and burn it much more completely, and in fact produce more fissile free energy equivalent of these fuels than the free energy equivalent of U235 we started with. Perhaps you're a nuclear engineer. Perhaps you're a particle physicist. But nothing you've said makes me give *you* any more credibility than the physicists and nuclear engineers I've had tell me these things. Nor does the paper you linked to, just read the author list and you'll understand why. I've been told by many credible sources that with breeder reactors fuel will no longer be an issue. You, a single non-credible source, are telling me that they're all wrong and directing me to a paper with what I consider to be an incomplete analysis as proof.
But at the end, there is simply truth. The paper you linked to clearly stated in the abstract that proliferation is the primary reason for their recommendations. The lead author is a political scientist. That should send up warning flags to anyone reading it that the examples *perhaps* are not being made by people who want the result of their analysis to be in favor of breeder reactors. Bias is everywhere, and it has to be kept in mind at all times when you're reading.
Personally, I think that when particle physicists tell me about the physics of energy conversion in breeder reactors, they're about as unbiased as it gets. So, I will continue to trust that source over the analysis of a group led by a political scientist.
The thing is that political and diplomatic considerations really do matter. Obviously we shouldn't let that colour our scientific assessments of things, but we do have to consider these issues.
A waste recycling and/or breeder programme for nuclear power means the possibility of nuclear weapons. This causes political and diplomatic concern, not only in our own countries with the NIMBY effect, but when we start wanting to extend the programme to other countries. After all, we can't solve the problems of peak fossil fuels and rising emissions just by going entirely nuclear in our country, can we? Global problems require global solutions. But we mightn't be keen on nuclear as a solution for all countries.
For example, Iran claims that their nuclear programmes are in part designed to help them be less reliant on a declining resource and greenhouse-gas emitting energy. We don't seem to be that keen on them doing it.
Are we in the West likely to be as happy about (say) Libya, Indonesia, Algeria, Honduras, Morocco, Sudan, Nigeria, PNG, and so on going largely nuclear with their energy? Do we really want Sierra Leone having a fast breeder reactor?
Or are we going to tell them, "you just have little reactors, we'll supply and recycle all the fuel"? Then they become very dependent on us. As former colonial possessions of the West, they're unlikely to be keen on becoming dependent on the West for their day-to-day energy supplies.
Or will we tell them, "well, we can be trusted with nuclear, but you can't be. We say it's impossible to get by with just wind turbines and solar panels, but you'll just have to try to manage it, because we're not going to let you keep burning stuff, and we won't let you go nuclear"? Our solutions don't look so global now, do they?
So these political and diplomatic issues are very important if you want to propose nuclear energy as a global solution to peak fossil fuels and climate change.
Hi Kiashu, for this comment. I agree completely, the political concerns are the primary roadblocks. I really don't mean to sound like I am a nuclear advocate, I'm extremely agnostic when it comes to such things and try my best to remove the bias from all the things I read. My posts were meant only to point out that you can't take everything you read at face value, because everything has bias, or even just mistakes, and it's important to never fully trust any source, ever.
A nuclear engineering professor once told me, "With nuclear power, you have to learn to not be too logical." It turns out this is also true with wind power (cape wind), and probably any other technology you could think of. If we lived in a magical world where it had never occurred to anyone to make fissile material into a bomb, we would probably have some level of functional breeder reactor technology now, right? I mean, the programs in the 80's weren't canceled (at least not directly) due to technical failure... we'll never know how those would have turned out, what we would have learned, and how the reactor designs would have evolved from there.
But we probably will have to learn eventually... Promethius and all... once we need power and fuel badly enough, concerns about proliferation will vanish in comparison to concerns about unstable governments with existing nuclear weapons (i.e. Pakistan).
Well, what I think most likely is that we in the West will build quite a bit of nuclear stuff, but we'll use force to present anyone with dark skin doing so. Most likely we'll have Euros, Japanese, East Asians and (maybe) Americans living in ecotopian cities surrounded by slums of impoverished Africans and Asians and South Americans who toil in the fields making biofuels.
And rising sea levels, too.
Believe what you like!
This is what nuclear cornucopians always do.
Or it warms the cockles of your heart to conclude that the study authors are a bunch of namby pambly politicians then at least post some science shows exactly how these breeders run on nothing but U-238 or thorium.
Bottom line, the only place we get nuclear fuel from is U-235
which is due to be exhausted in about 60 years at the projected rates of the IAEA.
I've looked in a lot of places and I can tell you that lots of different stuff has been tried most of it in the 1960s.
It is insane to believe that the French and Japanese have closed fast breeders that cost billions because the price of uranium was too low.
Reprocessing in France has been an 'economic' failure and breeder reactors which require nuclear waste(plutonium) for fuel are all closed except that state of the art Russian BN-600(1980) chugging away in the Ural mountains.
http://en.wikipedia.org/wiki/BN-600_reactor
I am not a nuclear advocate. I think it's probably a good solution, but I have no stake in nuclear beating out any of the other alternative to fossil fuels. I research advanced biofuels, and while I'm well aware of the limitations of biofuels, I still think it is worth trying to smooth the long decline in any way that is sustainable (where sustainable is defined as a 200+ year solution, trying to plan further than that is, I feel, rather presumptuous considering how much the world will have changed by then).
Obviously they don't run on nothing but U238 or thorium. However, they can run on U233 or P239, both of which are made from thorium and U238 respectively. The entire point of a breeder reactor is that you start with a stock supply of U235 (or better, U233/P239 so that you don't need separate reactors) and use it to transmute the otherwise non-fissile U238 and thorium into useful fuel. It wouldn't be a breeder reactor if it didn't produce more fissile material (in energy now extractable) than went into it. They are able to breed as much as 60% more fuel than went into the reactor.
Now, I'm no nuclear engineer, but this seems like pretty straightforward math. If after you're finished using the U235/U233/P239 you have more U233/P239 leftover than when you started, the reactor will run effectively indefinitely... instead of being limited by U235 availibility, you're limited by U238 and thorium availability, both of which are ~2 orders of magnitude more plentiful than U235. This obviously says nothing about economics, but that's not really the point in my opinion, the more important issues being primarily EROEI and a politically safe solution.
The biggest obstacle to breeder reactor technology is that they produce plutonium in a uranium matrix, or uranium in a thorium matrix. In both cases, the fuel is now chemically separable instead of being only separable by ultra high speed centrifugation. This is a proliferation concern, and is, from everything I've ever heard on the subject, the biggest reason why we don't use breeder reactors and instead the US tries to bully everyone into using light water reactors exclusively.
I don't have a great deal of time to look up scientific papers at the moment, however, the following paper is one of many I found with abstracts suggesting they found breeder reactors to be feasible. Most of the others were in Japanese, but these are both English.
----------------
Partitioning and transmutation (P&T) 1995. A review of the current state of the art
Skaalberg, Mats; Landgren, Anders; Spjuth, Lena; Liljenzin, Jan-Olov; Gudowski, Waclaw
The recent development in the field of partitioning and transmutation (P&T) is reviewed and evaluated. Current national and international R&D efforts are summarized. It is concluded that P&T is scientifically feasible and the recent R&D in the field of P&T makes it more tech. mature than a few years ago. Nuclear transmutation with energy prodn. is feasible in nuclear reactors where fast and thermal breeder reactors are the most efficient for transmutation purposes. The operation of subcrit. nuclear reactors by high current proton accelerators that generate neutrons in a spallation target is also an interesting option for transmutation and energy prodn., that has to be more carefully evaluated. These accelerator-driven systems (ADS) are probably the only soln. for the transmutation of long-lived fission products with small neutron capture cross sections and actinide isotopes with rather small fission cross sections. The requirements on the sepn. chem. in the partitioning process depends on the transmutation strategy chosen. Recent developments in aq. based sepn. chem. opens some interesting possibilities to meet some of the requirements, such as sepn. of different actinides and some fission products and redn. of secondary waste streams. In the advanced accelerator-driven transmutation systems proposed, liq. fuels such as molten salt are considered. The partitioning processes that can be used for these types of fuels will, however, require a long term research program. The possibility to use centrifuge sepn. is an interesting partitioning option that recently has been proposed. P&T is a complex issue with regard to environmental, safety, technol., economical, political and public acceptance aspects. In some countries (Japan, France and the Russian Federation) it has, however, received political or institutional backing as a complementary future nuclear fuel strategy. At present there seems to be no economical gain and only an insignificant redn. in future radiation doses from P&T as compared to the ref. concept of the closed fuel cycle and direct disposal of spent nuclear fuel. However, some of these conclusions can be changed by future long-term research and/or by a change in the economical situation.
---------------
or this one which is more succint:
---------------
The science of fast reactors and why it has been studied
Vendryes, G.
The basic advantage of fast n reactors, is clear cut. This type of nuclear reactor is the only one which makes possible to use in principle the whole and in practice a large part of the fission energy of natural U. By this it is meant the energy which would be released if all nuclei present in natural U could be fissioned. Slow n reactors in current use today release only a small fraction of the total energy.
If you breed at 1.0, you produce the same amount of fuel out as you put in. Thorium reactors are about .8.
Only plutonium reactors get above 1 at 1-1.6 and the real world reactors like Superphenix couldn't do more than 1.2 and they require fuel from normal LWRs.
See table 6.1 below.
http://tiny.cc/D1z2i
How easily 'chemically' separated is Pu-239 from U-238? Reprocessing is simply not economical looking at France's low rate of reprocessing.
I believe this talk of breeding by reactor is totally hyped.
We either develop a new breeder technology like accelerators (ADS)or forget about the expansion of commercial nuclear power IMO.
Shippingport reached 1.01 even with neutron-absorbing light water as the moderator. CANDU could do better, and the projection for liquid-fluoride thorium reactors is as much as 1.09 (relying on removal of Pa-233 to avoid unwanted neutron absorption).
And what's wrong with that? Every 5 cycles you can get enough fuel to start another reactor.
Not required. They can use the spent fuel, but all they need is U-238 (which constitutes the bulk of spent LWR fuel).
From the remove-the-beam-from-your-own-eye department:
That's plain wrong. LWR fuel is not required for breeders, and LWRs do not produce thorium (which you would know if you bothered to read). Fast breeders can use the other 96% of spent LWR fuel, though. They can also use natural uranium and depleted uranium (byproduct of uranium enrichment).
The entire point of a breeder program is to produce fissile U-233 or Pu-239 from either Thorium Th-232 or Uranium U-238 once natural uranium U-235 is exhausted.
I know you(EP) don't care but for those who might happen to read this,
the argument is where additional fissile will come from fuel once natural uranium and U-235 is exhausted in about 60 years according to the IAEA report.
Good question.
MIT study compares the three routes; 1) once thru nuclear in LWRs.
2) reprocessing spent fuel from LWRs into enriched uranium MOX
3) fast breeder reactors using plutonium from LWRs.
Why LWRs? Because almost all commercial reactors on the Earth is LWRs.
The result is that once thru chews up 300000 tons of natural uranium,
MOX chews up about 240000 tons of natural uranium and fast breeder/LWR combo chews up 166000 tons of natural uranium.
Conclusion--they all chew up uranium at similar rates so the effect on the Uranium Peak is only to stretch it out for a few years before the whole system breaks down.
Maybe you can burn up U-235 in dangerous super-hot fast neutron reactors but why do it? Only because they could make plutonium that would enrich fuel rods for LWRs.
LWRs have a marvelous safety record why change?
More mines, lower grade ores and undiscovered resources, and only if we haven't moved to something less expensive than once through reactors that sends the price of uranium down even further.
In which case we are near peak uranium from a simple collapse of demand.
You keep cherry-picking pieces of studies that reach opposite conclusions from you. From the study summary, page 4 (page 14 of the PDF):
Note that this is with the once-through cycle recommended in the study. If uranium supplies are truly as scarce as the doomsayers say they are, the MIT cost-based recommendation against breeders in a closed fuel cycle no longer holds, and the fuel supply expands by about 2 orders of magnitude.
They leave vastly more waste than is necessary, and require large, heavy, expensive and safety-critical pressure containers. It also appears difficult to expand the LWR system as fast as necessary to replace coal and other sources of GHGs. The full study projects that the 1000 GWe program would only displace "15-25% of the anticipated growth in anthropogenic carbon emissions" (Chapter 3, page 26). Merely displacing growth is woefully insufficient.
Thanks, EP for making my point.
We have 400 reactors now and based on IAEA study quoted by Gail we will run out of uranium between 50 years and 100 years given the expected growth from 20kt to 80 kt. Not thousands of years! The MIT report figures are for 1500 Gwe in 2050, about 4 times the current 370 GWe in the world. The MIT report seems consistant with thr IAEA report.
Frankly the thought that the world would produce over 1800 Gwe(remember retirements) in 40 years only to see them finally run ou of fuel in 50 years seems crazy also.
Peak Uranium is a fact, the nuke renaissance is an illusion.
At $130/kg from a log-normal distributed fuel. LWR's can operate at over ten times that price.
All this doomsaying, from the guy who just a little upthread said "LWRs have a marvelous safety record why change?"
You've been told time after time that the MIT study you base your argument on assumes a once-through cycle, since uranium supplies "are sufficient". Your argument is that uranium supplies are not sufficient, therefore things fall down. Uh-uh; it just means that the reliance on a once-through cycle falls down, and the very study you're using for support fails to back you.
And I quote myself, from the reply you didn't bother to read any more than you actually read (rather than quote-mine) the books you cite:
Actually, that's more like 3 orders of magnitude after thorium is included, but who's counting?
Gail,
A fantastic post, with equally interesting discussion, but there are two lines of development that haven't yet been commented on. I reckon these will impact within the time period under consideration.
1. The probable impact of the Australian Silex technology (under licence to GE, Cameco et al) that is the first generation III enrichment process using laser excitement. (ASX:SLX)
2. The impact of thorium/uranium seed and blanket having been promoted by America's Thorium Power. (OTC:THPWR) The Indians have little uranium but plenty of thorium and are forging ahead with this. Spent fuel cannot be used in bombs.
But then again they could be fizzers?
Similarly the possible impact of the EEStor barium titanate ultracapacitor on post 2015 demand for oil. They call it an electrical energy storage unit or EESU) that is "said to be" being commercialised by end of 2009 could revolutionise vehicle propulsion and require more nuclear plants and a better grid :-)
We live in a world of possibilities. So we should not underestimate either the ingenuity of man or the stupidity of politicians.
EI,
Wikipedia lists 4 thorium cycle reactors, none of which now produces commercial power. There was also thorium work at Shippingport, PA and St. Vrain in Colorado.
http://en.wikipedia.org/wiki/Fort_St._Vrain_Generating_Station
http://www.nukeworker.com/nuke_facilities/North_America/usa/NRC_Faciliti...
http://en.wikipedia.org/wiki/Thorium_fuel_cycle
I do like the potential of thorium but lots of experience is against it.
As I mentioned, we probably need a totally different technology to breed nuclear fuel which is why I like the Energy Amplifier of Carlos Rubbia. There also was the idea of Hans Bethe to place a bed of thorium inside a fusion reactor.
Fusion(EFDA-JET) hasn't worked so far but it still has some potential(ITER).
http://www.jet.efda.org/pages/jet-iter.html
http://en.wikipedia.org/wiki/ITER
Both these technologies do not rely on reactor 'waste' for fuel but do use electricity. JET produces fusion energy equal to 70% of the energy input.
What "experience" would that be?
Looks to me like the experience is for it.
reprocessing in the US was only illegal via Presidential order from 1977-1981. it's possible we'll see some kind of domestic reprocessing facility as part of GNEP.
thorium MSR designs need a charge of fissile material (e.g. U233) and have breeding ratios on the order of ~1.10 which means it takes several years to regenerate enough material to start a new reactor, but there is probably enough existing stockpile for initial rollout of MSRs, or you could build a handful of fast breeders for this purpose, I also believe CANDUs would work.
Of course....Jimmy Carter.
IT'S THOSE DAMN GREENS--HOLDING UP NUKE POWER!
(Still, no Greens were in the USSR.)
http://en.wikipedia.org/wiki/Chernobyl_disaster
http://en.wikipedia.org/wiki/Mayak
There's no cornucopian like a nuclear cornucopian.
I didn't say anything about Carter; other than having a fondness for all Democratic presidents, my opinions on Carter and his actions are limited as I wasn't alive to provide any context. Regardless, the problems and available technology in the 1970s were quite different than today's. In 2009, whether the US reprocesses or not has no effect on world nuclear weapons proliferation. Instead we're struggling with AGW and peak resources.
btw, no sense in baiting me with horror stories of the Soviet weapons program. I want to turn the worlds weapons stockpile into electricity.
The real story is that opening new mines is cheaper than reprocessing by a factor of ten, and opening more enrichment facilities for reenriching tailings (of which we have 50 years worth of supply) is also cheaper than reprocessing by a factor of ten.
The only reason anyone does reprocessing at all is political.
One of the papers linked above said that even reprocessing Russian bomb materials is expensive.
This makes one wonder how secure our plan to continue buying LEU (even at higher prices) is from Russia. (The 500 metric tons is the amount agreed on in the contract ending 2013.)
If the tails assay of the DU stockpile is 0.2% U-235, and you re-process this to bring the tails stream down to 0.1%, you're only going to get about 20% more fuel out. That may buy you a few years, but only a few.
The total amount of fissionables in spent LWR fuel is greater than that in DU, and fast breeders can destroy them while also turning the U-238 into fuel. Claims are that the fission products are 85% gone after 10 years, so the waste stream is radically reduced. This appears to be the way to go.
Sure. At ten times the cost. There will never be a market driven reprocessing case unless the fundamental economics change, because opening a new mine or enrichment facility is far cheaper.
This doesn't necissarily rule out entirely different types of reprocessing, say LFTR online reprocessing of fluid fuel, but I'd bet against any aqueous reprocessing facility (or even the much hyped pyroprocessing from ANL) gaining ground.
External reprocessing of oxide fuels is costly, but electrolytic reprocessing of metal fuel for IFRs and salt distillation for MSRs appears to be quite cheap. The push for oxides is precisely that they are hard to reprocess, which is advanced as an anti-proliferation measure. The IFR and LFTR are also proliferation resistant, but through different mechanisms.
As of 2002, there was about 43,000 tons of it per the EIA.
What I've been able to find in the way of assays says that it's perhaps 1.0% U-235 (slightly enriched) and about 0.8% Pu isotopes. Unfortunately, recycling to MOX would take a big, expensive, messy plant with a long lead time (perhaps France would be willing to do it for us). This is the kind of thing that may be best dealt with by converting to fluoride salts and using the Pu as the initial fuel charge for liquid fluoride thorium breeders (but that's not going to happen in the next ten years either).
list of working, planned and prospective uranium mines at wikipedia
http://en.wikipedia.org/wiki/List_of_uranium_mines#Prospective_uranium_m...
Thanks!
It adds some to the knowledge base, but there are a huge number of blank spots. More missing numbers than filled in ones, it seems like. I guess owners don't like giving out this information (and keeping changing it).
we have to be careful in extrapollating data for uranium reserves, the mining history of uranium is very short, barely 30 years since every prospection has stopped after Chernobyl and the collapse of the soviet empire that led to the dismantlement of nuclear weapons. Energy Watch group is an ecologist group so they don't like nuclear energy at all, I think they did a good work on reviewing oil and coal reserves but I don't trust their work on Uranium reserves estimates, they are too biased against nuclear.
Being said I have no clue how much uranium is still to be mined and nobody knows since we simply don't have the data, as mentionned above new sources of uranium can be mined like coal ashes, rock phosphate, granitic rock.
The Rossing mine in Nambia mines Uranium at an Ore concentration of 300 ppm at an energy cost 500 times less than the energy it delivers with current thermal-spectrum reactors. If the energy cost increases in inverse proportion to the Ore concentration, shales and phosphates, with a Uranium abundance of 10 - 20 ppm, could be mined with an energy gain of 16 - 32.
http://nextbigfuture.com/2009/02/revisiting-duration-of-nuclear-power.html
Insitu leaching
http://en.wikipedia.org/wiki/In-situ_leaching
"If the energy cost increases in inverse proportion to the Ore concentration, shales and phosphates, with a Uranium abundance of 10 - 20 ppm, could be mined with an energy gain of 16 - 32."
Can you please please show me where you got or calculated this?
From the sentance just before the one you quoted:
"The Rossing mine in Nambia mines Uranium at an Ore concentration of 300 ppm at an energy cost 500 times less than the energy it delivers with current thermal-spectrum reactors. "
For the full accounting:
"The Rossing mine produced 3037 tonnes of Uranium in 2004, which is sufficient for 15 GigaWatt-years of electricity with current reactors. The energy used to mine and mill this Uranium was about 3% of a GigaWatt-year. Thus the energy produced is about 500 times more than the energy required to operate the mine. "
The article is wrong in stating that Olympic Dam is operated by Rio Tinto. The company is BHP Billiton. Rio is looking at offloading coal and aluminium subsidiaries to Chinese state owned companies. These deals are under Federal approval scrutiny at the moment. Some Rio shareholders have called for BHP to revive its earlier but now abandoned takeover offer rather than fall into Chinese hands.
Olympic Dam has the opposite problem to Cigar Lake in that it has water shortages. A coastal desal plant 300km away hasn't progressed. When that happens the mine will produce up to 15,000 tonnes a year of U308 along with copper and gold but they seem to be doing OK at current metal prices without the need to expand. The tailings from the plant also contain a lot of rare earths and thorium.
I'd guess continuing reliance on Gen III reactors is a bit like flying in plane with limited fuel tanks while knitting a parachute. With peak coal in 20 years and rapid climate change nothing I have seen or read convinces me there is any alternative.
Sorry about the Rio Tinto mistake. It must have been getting late. I got it right two paragraphs above.
According to infomine,
I think lack of water is a big issue with quite a few mines in Africa and Asia.
A problem with the desal plant is where to get the power since the nearby gas pipeline is running low and wind/solar are intermittent. Apparently the idea of combining seawater cooling of a nuclear power station with desal is politically unacceptable. Mining royalties OK, nuclear power bad. The desal could use a combination of vacuum flash and reverse osmosis with warmed water. Opponents say the warmer and saltier outlet water will stress the cuttlefish in the bay. Perhaps the cuttlefish could have their own aquarium since at full production Olympic Dam could support 60 one-gigawatt reactors for decades.
Boof,
Nothing wrong with intermittent solar or wind, as its easy to store a few days production, and Pt Augusta doesn't have many cloudy days.
Sydney is going to run a desal plant form wind power. Saline water could be piped to deeper parts of Spencer Gulf or mixed with much larger volumes of sea water.
AFAIK neither the Pt Augusta solar desal nor the Sydney wind powered desal have produced a drop so far. BHP's proposed Whyalla desal was to produce 120 ML/day (31 million US gallons for the non-metric) needing power 24/7.
Spencer's gulf (in which they shot the shark scene for Jaws) narrows near Whyalla so the Cooper Basin gas spur line takes an underwater shortcut. It has only got a few good years left. There are two 250 MW lignite stations at the top of the gulf near Pt A where the water is slow moving and hypersaline. Thus dirty coal power helps load the drums of yellowcake on to the Darwin railway and then on to enrichment in the northern hemisphere.
The Sydney plant is due to start operations summer 09/10, supplying 15% of Sydney's water use. Wind turbines to power this being constructed now. I doubt that reverse osmosis requires to have power 24/7, smaller plants on cruise ships operate intermittently. They have to stop occasionally to back flush the membranes.
To be fair, >20% of S Australia's electricity comes from wind power, so its dirty coal and clean wind power.
This graph http://onlineopinion.com.au/images/article-images/quirk-160209-1.gif suggests 20% is way too high for SA. Multiply nameplate energy types by capacity factors. Lots of wind turbines, shame they hardly turn in 45C heat when all the ACs are on.
I had the impression their was a lot of very windy weather in SE Australia 2 weeks ago, 46C and 100 km/h wind; that's what spread the bush fires.
You are a little behind times as far as wind power in SA, since Nov 2008, 760MW capacity, will be >860MW in 2009. In 2008, additional installed capacity was 193MW.
http://www.ministers.sa.gov.au/news.php?id=3881
That linked article came out two days ago and said SA wind nameplate was 307 MW with 33% CF. I have a friend's photo of a becalmed wind farm (Wattle Pt) during the 2008 heatwave. They still need something to run the aircons when Leigh Ck coal and Cooper Basin gas run out soon. Also to create water and jobs. My spies tell me that Premier Rann is a closet nuke supporter but is afraid to come out.
Boof,
Clearly SA name plate is greater than 307MW (not sure what source you are referring to?)
This site lists the 740 MW capacity locations and the two under construction http://www.climatechange.sa.gov.au/index.php?page=renewable-energy-in-sa
Saying wind power is not suitable because it was not available in a heat wave last year or 5 years ago, makes as much sense as saying NG power is not suitable because of an explosion in the pipeline as happen in Melbourne 7 years ago and last year in WA, or hydro is not suitable because the Bass Link failed in a heatwave a few weeks ago. All power sources have some degree of unreliability, either hours( transmission), days( wind) or months( gas explosions); nuclear can be years if a reactor malfunctions.
Every kWh generated by wind in Australia is one less Kg of black coal or 1.5Kg of brown coal that is burnt.
What you say is only true to the extent that the coal fired plants can be modulated up and down in response to the higher wind availability. Something has to vary response to wind availability--usually it is natural gas, in the US, or hydro. I have heard that some coal plants can be modulated to some extent, but I would be interested to see how much savings there really is from wind availability.
Gail,
Where their is a large peak reserve of NG or hydro, additions of wind capacity will reduce the coal base load. In SE Australia, in the middle of summer, during heat waves, their is not enough peak supply, so all during those periods all coal plants would be running and wind would displace NG during off-peak periods or be used for pumped storage hydro. In WA, wind would displace NG that is used for base-load.
Since the US also has nuclear base load with low operating costs, any surplus wind energy would firstly, short term, displace NG and then coal(when all NG is shut down) when prices are lower than the cost of coal. If nuclear and wind expands, coal will be squeezed long term from both ends, by power produced with very low operating costs. The price of uranium would probably have to be >$1000/Kg( perhaps>$2,000/Kg) before a nuclear plant would consider reducing power. Wind of course will keep selling electricity down to zero price, or the price of transmission. NG peaking will still be required but their capacity factor( 10%) would decline and peak prices would rise, and base-load prices decrease.
The coal industry could encourage the replacement of existing older low efficiency plants with diesel power using coal/water fuel mixture. This give efficiencies >50% and can rapidly respond(30secs) to changes in demand. This would displace expensive NG peak but be cheap enough to also generate at shoulder periods so the capacity factor could be higher than the 10% that gas peak operates. That would be a better outcome than massive investment in CCS, that will make coal fired plants more capital intensive than nuclear, and more expensive to operate than NG
A few days of storage is already non-trivial, but you're going to need far more than that if you're not content to live in the most hellish deserts on Earth.
If you live in a place like the US you need ~80 days worth of storage for a solar + wind only grid(magical continent-wide HVDC grid that can shuffle unlimited amounts of power from anywhere to anywhere included).(reference: http://www.iesvic.uvic.ca/publications/library/Dissertation-Love.pdf)
Using the cost of the Northfield project(~$3.7 billion inflation adjusted, 11 GWh pumped storage) and the wiki numbers for US grid power demand(3,816,000,000 MWh/year ~ 0.43 TW avg.). The cost of this storage would amount to (0.43*10^3*80*24) GWh/(11 GWh) * $3.7*10^9 ~ $280 trillion($1.2 quadrillion for the world).
The very cheapest claims I've seen for vanadium flow batteries is $150/kWh incremental cost at very large sizes, putting the tab at $120 trillion(530 trillion for the world).
Soylent,
I was referring to storing a few days production of a desalination plant ie water, which can be stored for months or years ( in dams). Pumped storage is generally only used for a few hours to top up peak demand and use off-peak to pumped water back.
The one potential except for N America are the Great Lakes that can store very considerable amounts of water( and energy) with only a 1 meter change in lake levels.
Presentation on uranium, has info on Insitu leaching and cigar lake
http://www.ats-fns.fi/archive/esitys_jander_mori.pdf
they also indicate that downblending highly enriched uranium would seem to be a significant source well out to 2025-2030.
http://209.85.173.132/search?q=cache:Gme_E7-mFJwJ:www.aph.gov.au/SENATE/...
In situ leach uranium production involves the continuous circulation of relatively large volumes (20-40 million litres per day) of leach solution containing a relatively low uranium content (20-200mg/litre or 0.002 percent to 0.02 percent). These characteristics were taken into account in the setting of Government reporting standards during the field leach trial process.
20 milligrams out of 1000 grams is 20 ppm.
Context re uranium in environment and assuming an average house block size of 1,000 sq m, (50 metres by 20 metres) the volume of soil in the top metre is 1,000 cubic meters, or about 1,700 tonnes. The average crustal abundance of uranium is usually quoted as 2-4 parts per million. For 2 ppm, the amount of uranium in an average house block is 3.4 kg. For 4 ppm it is 6.8 kg. Thus, an average Adelaide house block contains between 3 and 7 kg of uranium in the top metre.
discussion of uranium. Professors says we need more
http://www.miningweekly.com/article/more-uranium-will-be-needed-says-wit...
“In low-grade primary areas, it’s vital to understand the mineralogy,” says Kinnaird.
For instance, some hosts refuse to release uranium in acid and only do so in alkaline solutions.
When acid leaching is appropriate, sulphuric acid is generally the acid of choice because of its ready availability and low cost.
Either sodium carbonate or sodium bicarbonate, or, in the US, ammonium carbonate, is used for alkaline leaches, which are preferred when the carbonate content of the ore is more than 5%.
Extraction rates of 80% to 90% are typical.
Milling is avoided and more-economic heap leaching favoured when recovering uranium from low-grade ores.
Primary uranium deposits occur in granites, the dominant mineral being uraninite, which requires acid leaching as opposed to brannerite, betafite and davidite, which require alkaline processing.
Secondary sediment-hosted uranium deposits are more widespread and, where abundant calcite-dolomite is associated with carnotite and coffinite, the use of acid becomes too costly.
Many sandstone-hosted deposits are amenable to in situ leaching.
294 page manual on insitu leaching
http://www-pub.iaea.org/MTCD/publications/PDF/te_1239_prn.pdf
Insitu mining costs and uranium from phosphate
https://netfiles.uiuc.edu/mragheb/www/NPRE%20402%20ME%20405%20Nuclear%20...
for 1 million pound of uranium oxide/year
$8 million for 125 closely spaced injector wells, 100 producing wells and 60 monitoring sites
$10 million for the plant to process and dry the uranium
2.5 month payback time if uranuim oxide is $90/lb
395 tons of fuel reprocessed at Rokkasho in 2008
http://209.85.173.132/search?q=cache:UdaVSLNEGHIJ:www.jaif.or.jp/english...
China reprocessing:
Based on expected installed capacity of 20 GWe by 2010 and 40 GWe by 2020, the annual spent fuel arisings will amount to about 600 tonnes in 2010 and 1000 tonnes in 2020, the cumulative arisings increasing to about 3800 tonnes and 12 300 tonnes, respectively. The two CANDU units, with lower burn-up, will discharge 176 tonnes of spent fuel annually.
Construction of a centralised spent fuel storage facility at Lanzhou Nuclear Fuel Complex near Yumenzhen in NW Gansu province began in 1994. The initial stage of that project has a storage capacity of 550 tonnes and could be doubled.
A pilot (50 t/yr) reprocessing plant using the Purex process was opened in 2006 at Lanzhou or Jiayuguan. This is capable of expansion to 100 t/yr and will be fully operational in 2008. A large commercial reprocessing plant based on indigenous advanced technology is planned to follow and begin operation about 2020. This is likely to be under international safeguards and situated in far western China.
In November 2007 Areva and CNNC signed an agreement to assess the feasibility of setting up a reprocessing plant for used fuel and a mixed-oxide (MOX) fuel fabrication plant in China, representing an investment of EUR 15 billion. In mid 2008 CNNC said that the focus was on Gansu province for an 800 t/yr reprocessing plant operated by Areva from 2025.
So it is possible to do as detailed an analysis for uranium and nuclear power as it is for oil drilling.
One cannot talk about "peak uranium" when one does not investigate how insitu leach mining works.
Sticking pipes a few hundred meters into the ground and running acid or akalyne through it.
75% of the new nuclear reactors are not going into the US or western europe (not in the OECD at all).
So whether the US nuclear regulatory agency approves a reactor design is meaningless to Russia, China, India, Japan and South Korea where most of the reactors are going.
Those countries have top engineers and the supply of uranium and the ability of the reactors to deliver the energy is definitely handled and assured out for decades. Thus they have the major transition to breeders out starting in 2025 or so and in full gear in 2050. Now it is possible they could be less concerned about mine tailings or other issues but they have a handle on supplying their planned hundreds of reactors with fuel.
The bottom line is uranium is a log normal distributed mineral throughout the entire crust, and oil is not. They aren't isomorphic in the least.
The concept of peak uranium is about as useful as peak aluminium.
If we don't have the power for extraction and refinement, peak aluminum and peak uranium may come at the same time. Neither of them can operate in a closed loop with only themselves as power.
How you can use electricity from nuclear to produce fuel. Use the sulfur-iodine process and make diesel and methane.
The proposed factory mass production of liquid flouride nuclear plants
http://nextbigfuture.com/2009/02/aim-high-plan-for-factory-mass-produced...
Use high temperature reactors for industrial process heat.
Ta-da closed loop industry.
There are also electric heavy duty trucks
http://www.autobloggreen.com/2008/06/17/heavy-duty-really-heavy-duty-ele...
Electric drive mining trucks
http://jalopnik.com/5054135/caterpillar-793-worlds-coolest-dump-truck-no...
electric jack hammers
http://www.tool-sale.com/electric-jack-hammer-heavy-duty-chisels-p-4045....
In Applied Physics we all dream of the day the price of uranium goes up by an order of magnitude. This will be the incentive required for the existing elite nuclear industry in Canada to get serious about gen4 fasts, and also get some serious money into fusion research by Canada and the US. If only those cold war stocks would deplete sooner, we could've been there 10 years ago.
Now we know why Obama is visiting Canada today.
To get more uranium, since Canada is the number 1 supplier.
Gail,
not being an expert there is a couple of facts I don' t understand about this kind of argument on uranium resources/depletion. Why do you limit economically extractable/minable uranium resources at a cost of ONLY 130 $/kg? Even with uranium at a 10 or 20x cost of mining, nuclear cost doesn't change remarkable...and you know, there are enomous uranium resources at a cost equivalent of 1000 or 1500 $ per kg U (even without fast or thorium breeders, or seawater uranium): current nuclear reactors produce about 40 MWh per kg of natural uranium, with peaks of 55 MWh/kg for CANDU technology (pratically, 130 $/kg for uranium is equivalent to a cost of one dollar per oil barrel...)
And, moreover, with a large positive energy ratio in energy invested in mining (rossing mine with a mineral concentration of only 350 ppm is more than 500 times the electricity produced from the uranium mined)
These were not my choice of limits. I just used information from other reports. Uranium prices have been very low for many years, so these amounts probably looked high in comparison.
Gail, it looks like you're missing the key uranium study called the "Red Book". This study assesses the world's uranium resources every two years. Here's the key highlight from the latest report:
Based on static consumption rates, in which nukes make a negligible difference... Ramp up nuclear by a factor of ten and that's only 10 years' worth of Uranium.
Plot those nice exponential growth curves, measure the area underneath, and....
Smoke and mirrors, I'm afraid....
However, if we are looking at tripling that rate (add in that which is being built and advocated to be built in China, India, US, etc), what would the projected timeframe be then?
Three times negligible is still negligible, alas...
Nuclear's just another diversion from solving the real problems.
I was thinking the Red Book was the same as the WNA's The global nuclear fuel market: supply and demand 2007-2030, which is the one the EIA references. It is for sale for £375.00.
I see the report you link to is by the NEA and the IAEA. It is available for only $186.
I get frustrated with all of these expensive reports. I like to read (or look through) the entire report, to understand what arguments they are making. The EIA's reports are free. It is difficult to buy several expensive reports for a one-time analysis.
In some ways I equate the NEA to the IEA, since they are both OECD organizations. It was an eye-opener to me when I did the research for the post I did in November called Objectivity of the International Energy Agency, related to our analysis of the World Energy Outlook 2008. One starts to wonder whether their reports are slanted to make whatever point OECD wants to make. Perhaps I am cynical. I haven't research the NEA.
The IAEA 2001 report I quoted replaced a decade-old analysis that they had done previously, according to page 14 of this USGS report. I had assumed that they didn't do in-depth analyses very frequently. Does the IAEA have real staff? Who is actually doing the work on the Red Book? What agenda are they trying to advance? I guess if I worked on nuclear / uranium on a more regular basis, I would get a feel for these questions.
In a world in which systemic natural failures due to population pressures seem to indicate the complete unfeasibility of the techno-paradigm, the last thing we need is more dangerous technology designed to continue the paradigm.
More energy equals more population which equals more energy which equals more population. The spiral is only ended by the complete collapse of the ecosystem. Then what? We live on earth as if it were a hostile planet we recently discovered, complete with sealed domes?
Why are we insisting upon the most destructive route? Why do we want to destroy the most complex, beautifully designed and tested, sealed environment we know of and will likely ever know of? It would seem that you would want to emulate and fall in line with billions of years of experimentation. After all, Earth Lab got it right. We and the environment developed coterminously.
This hellbent desire to one up this most elegant design we enjoy seems much like a rock band in a hotel, except we can't move to another hotel.
And, finally, since that tired old saw that development slows and even reverses birth rates will inevitably be raised; give me a break. The developed world's inhabitants each have a footprint equal to 70 people living in the undeveloped world. To bring everyone on the planet up to Western standards would effectively mean a population increase of 210 billion people. (Assuming that you count China and India as developed. If not then we are well and truly screwed.) We are already at 20% overshoot. It is unlikely we will survive our current technological levels let alone a much more advanced infection.
The real world is in stark disagreement with doomer-land on this point.
Cherenkov, you're using a computer, so I guess you haven't made the jump to a lower energy level yet.
Maybe you're waiting so that you can convince more people to follow you out of the hotel room? But if that was the case, why so vitriolic and undiplomatic?
I just don't get replies like this. Maybe you should write a campfire article and explain your reasoning.
Do you think that the OECD countries have the highest birth rates? Thanks.
In terms of resource consumption - YES. The average OECD citizen consumes 32 times more resources than an average citizen in poor nations (Most of the world).
So The US population given resource consumption x population is somewhere around 9 billion +
So yes, the OECD has the highest birth rate in the world given the sheer amount of resource use.
But if the birth rate is below replacement ( as it is in many OECD countries), then by your reasoning they have the largest decline given the amount of resource use.
It would be interesting to see if increasing resource use per capita made up for the declining birth rates.
Cherenkov,
You deserve a pat on the back.
Your post is lucid, clear and spot on.
Thank you
lukitas
Iran claims it has its own supply of Uranium:
http://uk.reuters.com/article/topNews/idUKTRE51I6N420090219?&sp=true
Thanks. I had only seen the earlier version, saying it didn't.
Geologically, uranium isn't that rare. What we need to know is uranium resource vs uranium price relationship. Antagonists use static models which can't be right, protagonists use massive assumptions and simple algorithms ("ten times the uranium resource with each doubling of price" "it's true for metal x so must be true for uranium too") which can't be right either.
Empirical research would be welcome since it is largely non-existent.
That massive assumption was made by Kenneth S Deffeyes who did serious research on uranium distribution. The results were that its a log normal distributed element in the crust because of its lithophilic chemistry.
The simplistic assumptions hold true for the Rossing mine in hard rock mining (which is typically more expensive than shales) that price is inversly proportional to ore grade. We have experience with these price relationships empirically from not only uranium mining, but mining of any mineral ore from iron to gold. To say that empirical research is nonexistant when we're basic positions on empirical observations is just silly.
Uranium distribution is not necessarily uranium extraction cost (ie linear relationship). We don't know the very low grade extraction costs, because it's not being done, and we don't know the effects of extremely high uranium prices on uranium resources since that's empirically nonexistant (uranium has always been cheap).
I'd like to see Kenneth S Deffeyes work. Do you have a link?
With the same type of ore (e.g. hard rock of similar chemistry) and the same mining technology we have extensive empirical experience (from not only uranium but gold, copper, aluminium) that indicate an extraction cost is inversely proportional to ore grade. These aren't assumptions made in a vacuum. Most likely extraction costs are less than inversely proportional because of advances in technology, economies of scale, and so on, also from extensive experience in mineral reclamation.
We mine gold of very low ore grades at reasonable cost. We also have some experience with uranium being much more expensive (in constant dollars) than it is today at the dawn of the nuclear age.
http://www.osti.gov/energycitations/product.biblio.jsp?osti_id=6665051
Thanks for the link.
This is exactly what I'm worried about - that the distribution might not actually be standard log normal, because the ideosyncrasies of uranium deposits are different from, eg gold. The authors make a good case for the distribution being normal, but empirically somewhat incomprehensive, which they admit. This is strange, since it suggests somewhat of a bias towards optimism of the authors.
Gail,
Thank you for another interesting post that has provoked some good discussion.
Unlike oil and gas deposits which are limited to specific geology, a narrow range in depths, and flow, uranium like other minerals does not flow, is widely distributed in the earths crust so their is no practical limit to the resource size, just what is economic due to the cost of extracting including energy costs.
The graph you have included from the energy watch group appears to be misleading. Proven reserves are regions that have been drilled out on a 50m grid. Estimated resources are inferred by the size of a known deposit containing the mineral and an estimate of the ore concentration in that strata that can be mined at a particular cost. Usually in one mine, estimated resources will be x3 to x10 larger than proved reserves except when the formation is very uniform, for example coal seams, when estimated can be X1000 larger. They will also be based on the same cost.
In the case of Australia, only 3 uranium mines were allowed to operate until 2008, and one of these, Olympic dam is a copper mine with uranium as a by-product. Thus, although many uranium deposits are known to occur in Australia, with reasonably rich uranium concentrations, owned by over 100 mining companies, they were not proven reserves because of the expense of drilling out. Clearly none of these deposits were included in the "estimated resources" on your graph.
So, should have 3 categories for each price level($40/Kg, $130/Kg( today's price) and some price $500-1000/Kg? that would increase the cost nuclear energy by 10%);
1)proven reserves, 2)estimated resources, 3)possible resources based on known uranium concentrations of various deposits and geologic strata.
Even at $130/Kg possible resources are likely to be much, much higher than proven reserves at $130/Kg, because the only mines in operation until 2008 were built when uranium was $20/Kg so the only proven reserves and estimated resources at $130 are the same mines that were developed years ago for $20/Kg uranium or mines like Olympic Dam, "proven" for copper where uranium is a by-product.
A general rule for minerals is if you double the price, existing mines will be able to double or triple "proven reserves" with little or no more drilling, and possible resources will go up X10 fold, because many lower grade deposits would be economic to mine. They will still have to be drilled out before they are "proven reserves" and the size of this will depend upon the estimated long term price. Only a small part of a deposit is usually drilled to "proven reserve level" just enough to get financing to start the mine. When in operation, more drilling usually increases the "proven reserve" and thus extends the mine life or allows mine expansion.
If the uranium price stays at $130/Kg for >10years we will have many more mines in operation, a big jump in proven reserves and estimated resources. If that price then jumps, to say $260/Kg both proven and estimated resources will jump without any additional drilling or exploration.
An article by Charles Barton has convinced me that peak Uranium is not an issue.
From the Nuclear Green Revolution quoted in full
http://nucleargreen.blogspot.com/2008/03/cost-of-recovering-uranium-from...
The Cost of Recovering Uranium from Seawater
A Japanese report, Recovery System for Uranium from Seawater with Fibrous Adsorbent and Its Preliminary Cost Estimation, Takanobu Sugo, Masao Tamada, Tadao Seguchi, Takao Shimizu, Masaki Uotani, and Ryoichi Kashima has translated into English by The Analytical Center for Non-proliferation. The report, first published in the Japanese Journal Nihon Genshiryoku Gakkaishi, discussed the Japanese methods of recovering uranium from sea water. (Also continued here.) Much of the information in this Japanese report is startling and even amazing.
The report states:
"At the Takazaki Radiation Chemistry Research Establishment of the Japan Atomic Energy Research Institute (JAERI Takazaki Research Establishment), research and development have continued for the production of adsorbent by irradiation processing of polymer fiber. Adsorbents have been synthesized that have a functional group (amidoxime group) that selectively adsorbs heavy metals, and the performance of such adsorbents has been improved. Uranium adsorption capacity of this polymer fiber adsorbent is high in comparison to the conventional titanium oxide adsorbent. We have reached the point of being able to verify the attainment of 10-fold higher adsorption capacity on a dry adsorbent basis. This adsorbent can make practical use of wave motion or tidal power for efficient contact with seawater. This adsorbent has been used since 1996 in the actual marine environment by utilizing moored small-scale test equipment for recovery of trace metals, including uranium, from within seawater. As a result, it has become apparent that use of this adsorbent makes possible recovery of seawater uranium with higher efficiency than the earlier method."
------
A recovery system based upon this adsorbent uses ocean current to produce efficient contact between the adsorbent and a large volume of seawater. According to the basic conditions of Table 1, the required quantity of adsorbent (quantity at the time of mooring) becomes 40,000 tons, and the quantity exchanged due to adsorbent performance decline becomes 10,000 tons per year.
Adsorbent is used in the form of 15 cm wide strips of nonwoven sandwiching a spacer and coiled into a short cylindrical shape. This roll is loaded into a cage (adsorption bed = short cylindrical shape of 4 m diameter) as shown in Figure 4. A single adsorption bed is loaded with 125 kg of adsorbent. The quantity of adsorbed uranium per bed during 60 days is 750 g. These adsorption beds are strung and tied together by rope at roughly 0.5 m intervals to form 1 basic unit.
125 kg of adsorbent is loaded into a single adsorption bed. Specifically, the adsorption bed is a metal mesh container (cage), formed from stainless steel, that has specific a gravity of 7.8 and a mass of 685 kg. A 15 cm wide sheet of adsorbent (150 g/m2) is coiled so as to load 125 kg of adsorbent. A plastic mesh sheet is inserted between adsorbent windings as a spacer. The specific gravity thereof is 1.15 so total mass is 104 kg. Total bed mass becomes 914 kg. The weight in seawater becomes 611 kg, so the weight when pulled up becomes 1,161 kg.
-------
Although 40,000 tons of adsorbent must be produced beforehand prior to the start of uranium recovery, production then becomes 10,000 tons per year for replenishment during the time period of regular uranium recovery. We made a trial calculation of the cost of manufacture of 10,000 tons per year of adsorbent. Details of this calculation are shown in Table 2. Precursor material cost occupies a large proportion in comparison to production equipment cost. Even if we were to assume an increase in production equipment for annual production of 40,000 tons per year, the equipment cost increase would be held down to slightly more than 2-fold. From such estimates, production unit cost of adsorbent was estimated to be 493,000 yen per ton (493 yen/kg). The quantity of recovered uranium becomes 120 kg per 1 ton of adsorbent for the case of 20 reuses. Therefore the adsorbent production cost required for recovery of 1 kg of seawater uranium is estimated to be 4,100 yen/kg-U.
The most interesting aspect of this report is the cost of this radical recovery method. The report states. "The recovery cost was estimated to be 5-10 times of that from mining uranium. More than 80% of the total cost was occupied by the cost for marine equipment for mooring the adsorbents in seawater, which is owning to a weight of metal cage for adsorbents. Thus, the cost can be reduced to half by the reduction of the equipment weight to 1/4. Improvement of adsorbent ability is also a problem for future research since the cost directly depends on the adsorbent performance."
Would the Japanese sea water extraction technic make nuclear power too expensive? Not at all. The cost of nuclear fuel is only a minor part of the expense of nuclear generated electricity. And since alternative technology can extract 130 times as much energy from nuclear fuel as is being extracted now, consumers potentially won't notice the difference on their power bills.
Different methods of mooring the absorbents were investigated by the Japanese, and the cost of each estimated. The Japanese estimate that it would cost between 30,000 and 56,000 yen to recover one Kilogram of uranium from sea water. At the current exchange rate the yen is pegged at a little more that 100 per dollar. So the recovery cost would be between $250 and $135 a ton. The Japanese, in 2001 stated that they planned more research directed at lowering materials input,and increasing the efficiency of the process.
Spot Market prices for uranium, which had been as low as $7.00 a pound in 2000, peaked at $136 a Pound in June 2007. current prices range from $70 and $75 per pound, and prices are expected to rise to the $100 to $110 range during the next two years. Clearly as new reactors begin to come on line, the price of Uranium will rise to the point where sea water recovery of uranium will be economicallty viable.
How long can we keep extracting uranium from the see? There are approximately four and a half billion tons of Uranium in the see. If you are worried about that running out, Jim Muckerheide has an interesting observation: "The consistent 3.3 ppb U in seawater is in chemical equilibrium. If it were being depleted, we would expect that additional U would be leached and put in solution from ocean bottoms, hydrothermal vents and cold seeps, and terrestrial sources (primarily through tidal pumping on the continental shelves, with some from rivers and other discharges). If we extracted a billion tons over hundreds of years, it is more likely that the oceans will contain nearly 4.5 billion tons than be reduced to 3.5+ billion tons."
Jim then asked: "Is this a "renewable" energy source?"
It appears so. Estimates of the amount of uranium in the earth's crust is 40 trillian tons. If Jim Muckerheide is correct there is a chemical equilibrium between crustal uranium and uranium in the sea. Since the amount of uranium in the sea is a tiny fraction of the ammount of uranium in the crust, the uranium supply in the sea will keep on replenishing as long as the earth lasts.
Uranium is a renewable resource. Uranium is here for the long hall. And just think, Thorium is 3 to 4 times more plentiful than uranium.
And the FED will magically print away all the world's troubles as they have a printing press and can save the global economy at the touch of a button. Money is infinite! And Politicians will guarantee good weather every Sunday for eternity - blue skies and sun for all. And while we're at it why not eliminate world poverty and create heaven on earth with our lord J.C.?? :-)
We'll build a hydrogen powered economy with the help of infinite nuclear power and we'll have infinite water, food, land, freedom and prosperity!
Happy days are here again!
I think your assumption about how the reserves are derived is incorrect. I don't think there is really any connection with operating mines. This is the distribution of production and reserves by country, from the Energy Watch Group report.
Also, there have been some pretty big increases in prices, with a corresponding increase production. There are some mines on"standby status" that theoretically could increase production, if prices went enough higher. I would find your arguments more believable if France's production had been in line with, or higher than the reserves. There are a lot of ways one can come up with reserves. Adding up reserves by company was not what was used in this case--it is quite a bit higher than that.
Gail,
Australia has 25-40% of the worlds reserves because of one mine, Olympic dam that has been drilled out much further to justify copper expansion. It would never have been opened for uranium alone and would have had 0 uranium reserves, except for the co-located copper and gold. Uranium from Olympic dam is being sold under long term contracts at $18/Kg(seemed a good price at the time for a by-product).
The case of France I think is because they wanted to be self sufficient in Uranium during their nuclear weapons development. Same for China; high production low reserves. Similarly for E Germany and Czech, mined out small rich deposits for cold war nuclear industry cost not an issue until 1990.
You will notice that nearly all producing mines were discovered in 1960 and 1970's during first uranium price spike. Only in last 2 years are mines discovered much later starting to be developed. In time these mines will drill out additional reserves and inferred resources.Most deposits in Australia will not be developed until share-market or Chinese or Japanese capital is raised, and then reserves and resources will rapidly expand.
Other copper mines like OZ metal's Prominent Hill( which has had a take-over offer from China; awaiting approval) will "find" reserves of uranium, while drilling out for copper and gold. Its a bit like the early oil days when gas was discovered while looking for oil, but low demand for gas, limited gas exploration.
At current rates of usage, there's enough terrestrial uranium to last about 246 years. Increased enrichment and utilization of spent fuel could double this to about 492 years. CANDU reactors can use thorium. If spent so if spent fuel in the form of uranium 233 were reprocessed and used for these reactors then the supply of terrestrial uranium would last about 1200 years.
The world only runs into a terrestrial uranium shortage if the entire planet has to run on uranium. But even with out breeding technology, uranium from seawater to power the entire planet for more than 3000 years and more than 5000 years if spent fuel is utilized.
Of course, with breeder technologies, we could power all of human civilization essentially forever.
Refernces:
How long will the world's uranium supply last? (Scientific American)
http://www.sciam.com/article.cfm?id=how-long-will-global-uranium-deposit...
Fueling our Nuclear Future
http://newpapyrusmagazine.blogspot.com/2008/10/fueling-our-nuclear-futur...
How long will uranium last?
http://newpapyrusmagazine.blogspot.com/2009/01/how-long-will-uranium-las...
Supply Uranium
http://world-nuclear.org/info/inf75.html
Marcel F. Williams
http://newpapyrusmagazine.blogspot.com/
Why not someone in the nuke industry? Anyway, he assumes no growth. And increasing the energy spent to get the uranium will lower the EROEI.
The seawater technology is not commercial yet. And we also don't seem to have a commercial breeder which seems competitive with current diffusion enrichment. It will be interesting to see if the new proposed reactors fare better than the last generation.
I have a few points, some of which have been touched upon by excellent posts.
First, I'll reiterate the point that since the raw uranium ore cost is only a few percent of nuclear power's total cost, much higher ore prices (i.e., up to 10 times the $135/kg cutoff that is arbitrarily used in these "official" resource estimates) could be affordable. As the allowable price increases, the amount of usable ore (i.e., the "reserves") increases exponentially. This alone is worth well over an order of magnitude in eventual reserves.
Second, these estimates and reports do not account for (or vastly underestimate) new uranium discoveries. The official oil reserves estimates in the 1920s were on the order of 1% of what we now know the total endowment to be. And it's mostly not because we've been going to "lower grade", or harder to extract oil (in terms of money or energy input). No, it's mostly because we've subsequently found massive deposits of high-quality/grade oil. Back then, we didn't even know about Saudi Arabia (Ghawar, etc..). This was the result of billions (hundreds of billions?) of dollars in exploration efforts. Orders of magnitude more effort than what has been spent so far looking for uranium.
As most peak-oil folks have learned, resources like oil or uranium all have a discovery curve. The rate of discovery ramps up, plateaus, and eventually falls back to ~zero as all the material present is discovered. The question is, where is one on the discovery curve. Obviously, this is question is inherently difficult to answer conclusively, but one can make an educated guess. The reason why peak oil theory has the traction it does is that we've been finding very little new oil and gas despite massive efforts to do so. There's also the part about how the biggest fields are easiest to find and will be found first, etc.., something which is much less applicable to uranium.
The point is, we have made negligible efforts so far to find uranium, compared to the effort that has been made (and is being made) to find oil and gas. Added to this are the theoretical geological analyses (that some have posted here) which point to vast quantities of uranium in the earth's crust. For these reasons, it is likely that we are at a very early point on uranium's discovery curve. I expect that we will eventually find at least 10 times, and probably more than 100 times the amount of uranium (of all grades) that we currently know about. We will probably find enough high-grade ore to last over a century, even with large growth in nuclear and even w/o any breeding or reprocessing. A hundred years is more than enough time to develop breeders, fusion, renewables, or some combination of these sources, all of which can provide all our energy indefinitely.
I know of nobody in the industry (utility execs, etc.) who is even remotely concerned about long-term uranium supply. Near-to-mid-term, perhaps (i.e., getting new mines started). Long-term, never. The issue does not even come up. These are people w/o a political ax to grind, but (instead) are reposnsible for putting up huge amounts of their company's own money for plants that will run for 60-100 years. If there was a real problem, they'd know about it, would be a lot more concerned, and they would not build new plants.
One final point. I agree with some others that some of the EROEI values we're hearing for nuclear are nothing short of ridiculous. They are based on politically motivated, and thoroughly debunked studies (e.g., Storm, Lleuwen & Smith). The many respectable studies that have been done on nuclear's net energy inputs, and the related topic of nuclear's indirect CO2 emissions show that nuclear's EROEI is on the order of 100, even with the once-through cycle. Nuclear's energy inputs are simply not significant, and most of them could be provided by electricity, if necessary. Also of note is the fact that renewable sources like wind and solar actually require significantly more raw material like steel and concrete, per unit of energy generated. In any event, if oil and gas really are peaking, and if CO2 emissions are taxed/limited, any significant energy/fossil inputs to nuclear power (as well as other sources like renewables) will show up in their final price. This is something that the market can sort out.
Much the same was said about oil around 1990.
It would be isomorphic I suppose if uranium was produced by vegetation eons ago in rather thin traps in specific regions of isolated geology.
Its not. The analogy is ridiculous on its face.
JimHopf
Your post might a little too much on the optimistic side but overwhole I tend to agree on it, unlike oil we don't have enough history in the Uranium prospection and extraction to do extrapollation on the peak of production at this point. And the curves speak for themselves, if you look at the Energy Watch group graph you see that the production of U collapses after 1980 (and we know why...) by a factor 2 and stays at this level for most of the time. This implies that reopening the mines that had been closed at the time would bring back the production at the 1980 level without need for new discoveries which would also roughly balance the current demand for some times.
I expect that quite a few mines that were open then would not be acceptable now because of pollution issues.
Good article! I'd like to see an oil watch monthly type of report of all the reliable figures.
AREVA is a French-owned company that operates a reprocessing plant in France, where MOX fuel is routinely used in power reactors. AREVA has begun construction of a MOX facility in the US at Savannah River Site, with a projected completion date of 2016. The initial purpose of this plant is to downblend weapons plutonium into low-enriched MOX fuel for power reactors (AREVA already does this with weapons uranium at Nuclear Fuel Services site in Tennessee, and the downblended uranium fuel is used in TVA power reactors). However, converting the facility to reprocess spent fuel instead of just weapons Pu would not be difficult.
I worked with a guy who did uranium and enrichment purchasing for a major US utility. He said that the main impediment to reprocessing was that uranium was just too cheap for it to be economical. There was no big support for it in the industry, as dry cask storage was a short-term viable alternative to store the spent fuel. He said that the break-even point was around $100 a pound for uranium - of course it was higher than that for a while but now it is down around $50.
Anyway, bottom line is that new supply of weapons material and possibly reprocessed reactor fuel will come on line around 2016 - 2020 or so. Aside from that, none of the major players in the US industry are seriously working on breeder reactors, thorium, fusion, etc. They may be technically feasible but they are not even on the radar in terms of commercial construction and operation. Reprocessing on the other hand, seems very likely to happen in a relavent time frame.
Any idea about capacity?
It isn't the pessimists who make me nervous, they probably worried about Y2K. It's the optimists whose plans are simultaneously too big to be practical and too small to do the job. Yea I've been guilty as well. The problem with most energy proposals, including nuclear, is that are expensive up front, then cheap to run. This runs into the peak credit problem. From an ERoEI perspective: the EI is now and with the ER too far in the future. This jogged something in my memory: Long ago someone had this idea for very cheap nuclear power stations. The only problem was that after a while you had to just abandon the station and build another one. Google is failing me, does anyone remember more? Save the flames.
You could be talking about the Hyperion modular system mentioned upthread or the Toshiba nuclear battery.
Since EROEI X payback = plant life for a negligible fuel cost system for say 50 year plant we really want fast payback. That's because we can only spare a limited fraction of our current energy use to invest or embody in new generation. That's from a physical perspective which may or may not gel with capital markets. The starving predator has to burn body fat to hunt for its next meal or it dies.
Fast payback is also why wind should be expanded to its niche of x% of the energy mix. New transmission, storage and peaking backup will lengthen that payback however. Thus the non-wind new generation should also have fast payback, say less than 5 years.
A 50 year life nuke plant with EROEI of 10 would have a payback of 5 years. Better still is EROEI 50 and 1 year payback. We can afford that easier.
The EROI of nuclear is probably closer to 100, but you don't start getting that back until it's operating. That's several years after the start of construction; a wind farm with an EROI of 20 built in 18 months returns its invested energy after 30 months.
This isn't an argument for one or the other, because we need both. Large amounts of wind require energy storage of some kind, such as CAES; molten-salt reactors happen to be ideally suited to providing high-temperature heat, and could replace natural gas for the CAES reheat step. They fit together hand in glove.
Gail - I'm rather late to this party. Looking at Figure 5 it strikes me that there was a rather sudden abandonment of U mining around 1990 and this I imagine was due to combined factors of Chernobyl (1986) and nuclear weapons treaties. So the trends don't really tell us much about resource levels - pretty much like the oil production profile for Saudi Arabia.
I think the key and perhaps most interesting element of Fig 5 is how public opinion has moulded international energy policy. Elements of that same public seem to be against all forms of energy production.
Sadly, yes.
Here in Victoria, Australia we had some plans to whack up wind turbines along the windy coast. The locals, who were not true locals but had gone there to retire (a "sea-change" it was called) protested heavily - the blades would hurt their view, they said.
My first thought was, "would you find coal smokestacks prettier?"
Then I thought, well, we're in a democracy, people ought to have a choice about what they have in their backyard. They ought to be able to choose any locally viable power source (ie can't choose tidal in the middle of the desert), and if they want, choose to have nothing at all - but then of course they'd have no electricity at all. But if that's what they want, they ought to get it.
Thus the poll at my blog, which I think ought to be done in each electorate around Australia - tell people, five years from now all current generators will be shut down, and your electorate has to have some generation of its own - if it runs short, others will help out, but it has to have something. I'd make it preferential voting, of course, because that's the Australian way :)
It'd be interesting to see how people voted, then. I suspect those old sea-changers wouldn't mind wind so much then, and coal would be instantly abolished.
HI All.
In terms of filtering sea water to get uranium, I'm not sure the scale is practical. If my calculations below are correct (please check), it will take filtering 6 times more water than is consumed by mankind each year, or 22,000 cubic km to feed just the current reactors. I don't see how this can be accomplished. I agree if we can get breeders going, less will be needed, but considering we could be down to 50% in just 20 years, I'm not sure we can build reactors fast enough to make a difference. However, if we bridge over to them from renewable and conservation, then that would be different. The killer that we all keep running into is our institutions are not willing to publicly say how bad things are, so all large scale, rapid approaches are pretty much excluded. The bottom line is it will take a crisis before any meaningful large scale solutions are considered.
The one Japanese paper I read on this topic imagined solving the flow rate problem by putting the whole apparatus in the middle of some fast moving ocean current. The processing plant would look like an oil rig with huge nets and streamers. It would pull in older strands, clean them of useful minerals, and redeploy them. The current would always insure that fresh supplies of uranium were passing.
How many platforms would you need I wonder?
If any country would have good reason to try this, it would be the Japanese. They have not yet put it into commercial production, so I wonder where what is the catch?
I' m rather conviced that seawater uranium is a pratical strategy only with fast breeders : there is a factor of about 100 between the electricity produced for kg of natural uranium in breeders and currrent nuclear reactors technology; that means, if today we extract about 100 kg of coal equivalent of uranium per 1000 cubic meters of water, with breeders it becomes a more reasonable 10 tonns of coal (assuming a NON-reasonable efficiency of adsorption for 1000 cubic meters of 100%!). However, there is no need to pump all that water elsewhere, simply the water is going to be adsorbed in situ by special "filter rods", expecially where there are naturally intense flow of water, no need to transport so huge mass of water on the soil
On the other hand,however, breeders can be feeded only with the uranium currently stored in nuclear waste sites, so I don' t really understand the need of seawater uranium
It is a real pity that reserves, be them reasonably assured or inferred, be given in terms of monetary units per kilo of uranium extracted.
Because money is becoming more and more a fiction, a changing reference measure.
Will we have the same level of resources at, for instance, 130 US$/Kg when the oil is at 140 US$/barrel or at 30US$/barrel? Because oil is a primary element needed for exploration, extraction, refining, transporting the mineral and then the fuel.
Will it be the same if the US$ versus the Canadian Dollar suffer a drastic exchange rate movement? Will it be the same if, for instance, Nigeria says it will only accept payments in Euros and the Euros goes from 1US$ = 1 Euros to 1 US$ = 0.6 Euros in one year?
Will the inferred world uranium reserves be the same now that if the US$ in circulation go up in 20 trillion in one year time? Or if hyperinflation or devaluation makes a house to be worth 300,000 US$ to 100,000 US$ in seven months or a loaf of bread going from 0,5 US$ to 5 US$?
I miss an energy equivalent system of reference, to know when things will really stop being produced for either EROEI reasons, or if any of the complex and numerous links of the uranium cycle chain fails.
That's because resources are defined as what is economic to extract at a specific price.
In any case, the 3,000Gj/tonne for a low grade ore is still a good EROEI(50:1), other parts( reactor construction, decommissioning, fuel enrichment) are more important until we get down to very low ore grades
I don't see why giving uranium reserves in terms of cost of extraction is a problem, it's basically the same thing we have with oil. I mean, if oil stayed at $35/bbl then the old tar sands wouldn't be mined for long, and nobody would be talking about shale oil, or the deep sea stuff under salt layers off the coast of Brazil.