Mining the Oceans: Can We Extract Minerals from Seawater?
Posted by Ugo Bardi on September 22, 2008 - 5:15pm in The Oil Drum: Europe
Figure: Japanese researchers testing uranium extraction from seawater using a braided adsorbent fiber (JAEA 2006). Is this the way of mining of the future?
After a couple of centuries of mining, the best and most concentrated mineral ores are on their way to disappearance. In the future, we'll have to extract from less concentrated deposits and that will be more expensive. It is not just a question of money; mining low concentration deposits costs more energy and, with fossil fuels being rapidly depleted, that is a serious problem. Our society cannot survive without a cheap supply of minerals; so, it may not be too early to look for new sources.
If mines on land are gradually becoming depleted, could the oceans become our new mines? There have been several proposals for mining the oceans' floor, but that is just an extension of conventional mining and, besides, the task has proved to be complex and expensive. The real change of paradigm, instead, is in extracting ions dissolved in seawater.
The oceans are vast and contain immense amounts of minerals which, in principle, could be recovered without the need of digging, crushing, processing, and all the other complex and energy expensive procedures that we need for mining on land. Indeed, the extraction of minerals from seawater is a concept that periodically reappears in times of energy crisis. It had become popular with the first oil crisis of the 1970s, only to disappear during the phase of relatively low oil prices that followed. Nowadays, with the new crisis ongoing, recovering minerals from seawater is looking attractive again. For instance, over the web it is often stated as an obvious fact that any uranium supply problems that could occur in the future will be easily solved extracting uranium from seawater. Occasionally, we read that the same method could be used to solve all mineral shortages.
However, things are not so simple and we'll see in the following that extracting low concentration minerals from seawater is a huge, expensive and complex task. We are not going to see minerals produced from seawater taking the market anytime soon and the dream of fishing uranium from the sea is destined to remain just that: a dream. But let's go into the details.
1. Minerals in seawater
Open ocean water contains dissolved salts in a range of 33 to 37 grams per liter, corresponding to a total mass of some 5E+16 tons, (in the "E-notation", E+16 means 10 elevated to the power of 16). In other words, the oceans contain some fifty quadrillion tons of dissolved material. It is a huge amount compared to the total mass of minerals extracted today in the world: of the order of "just" a hundred billion tons per year (OPOCE 2000). However, most of the mass dissolved in the oceans is in the form of just a few ions and these are not the most important ones for industry.
The four most concentrated metal ions, Na+, Mg2+, Ca2+, and K+, are the only ones commercially extractable today, with the the least concentrated of the four being potassium (K) at 400 parts per million (ppm). Below potassium, we go down to lithium, which has never been extracted in commercial amounts from seawater, with a concentration of 0.17 ppm. Other dissolved metal ions exist at lower concentrations, sometimes several orders of magnitude lower. None has ever been commercially extracted.
But let's see exactly how we stand. In the table below I have listed the seawater concentrations and total amounts of some metal ions. The table excludes those already being extracted (Na, Mg, Ca and K) and those which exist only in traces so minute that extraction is simply unthinkable. The amounts available in seawater are compared with the reserves listed by the United States geological survey (USGS). The concept of "reserves" may be conservative but the results of a recent work (Bardi and Pagani 2007) show that it may be the most realistic estimate of what we can actually extract from land mines.
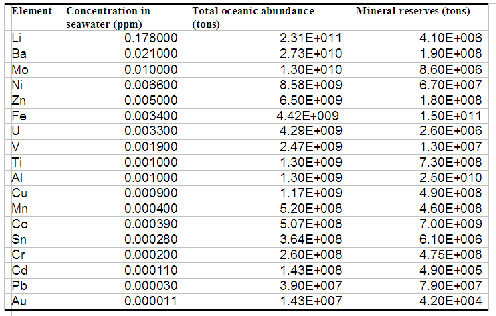
For data sources, see note (1) at the end of the text
As we see, there are huge metal resources in the sea. The question is how to extract them. The most general method consists in passing seawater through a membrane that contains functional groups that selectively bind to the species of interest. No known membrane is 100% selective for a single species, but it is possible to create membranes that can retain a small number of selected low concentration species. The adsorbates can be extracted from the membrane by flushing it with appropriate chemicals; a process called "elution". After this stage, the metal ions can be separated and recovered by precipitation or electrodeposition.
In practice, it is very difficult to extract low concentration ions at reasonable costs. Lithium extraction was tried in the 1970s (Schwochau 1984) but the tests were soon abandoned. The idea of extracting uranium has been around for a long time, at least from the 1960s (see Nebbia 2007 for a review). But just a few grams were extracted in Japan in the late 1990s (Seko 2003). Then, there is the old dream of getting gold from the sea. The German chemist Fritz Haber tried that in the 1920s but the task of extracting gold ions at concentrations of a few parts per trillion (ppt) was nearly desperate and, indeed, the attempt was a total failure.
Evidently, we have big problems here. That is not surprising: there is a lot of water in the ocean and, in comparison, very small amounts of useful metals. So, we have to process huge amounts of water. Huge, in this context, means really huge , as you can see in the following table. Consider, as a comparison, that the total volume of water desalinated today is 1.6E+10 tons.
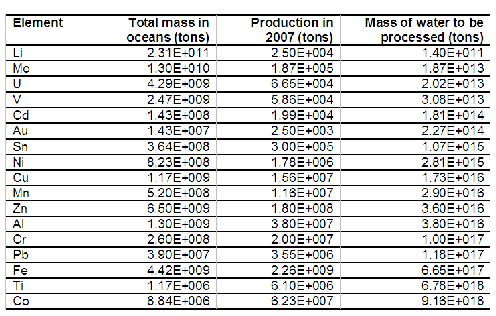
Table 2. Elements are ordered as a function of the mass of seawater that would need to be filtered in order to obtain the same amount of materials that we obtain today from traditional mining. That value is calculated in the optimistic assumption of 100% efficiency of the filtering membrane. For data sources, see note (1) at the end of the text
The table shows that, even for the best case listed, lithium, in order to recover the same amount we get today from conventional mining we would need to set gigantic facilities. We'd need to process at least ten times as much water as it is processed by desalination plants today. All the other metals would require to process amounts of water orders of magnitude larger.
Moving these gigantic amounts of water is not just a practical problem: it involves energy; a critical parameter especially if we consider the extraction of two elements that are to be used as energy sources: lithium and uranium. Uranium, in the form of the 235 isotope, is the fuel of the present generation of nuclear fission plants, whereas lithium, in the form of the 6-Li isotope could be the source of tritium to be used as fuel for a future generation of fusion power plants. In both cases, the feasibility of extraction is determined by the energy needed according to the well known concept of "EROEI" (energy returned for energy investment) (Hall 2008).
In the next section, we'll see in detail the case of uranium, perhaps the most important for practical applications and the one for which we have the best data available. It will serve as a benchmark for evaluating the feasibility of extraction of all the other elements.
2. Uranium extraction from seawater
At present, the mining industry can provide only about 60% of the uranium needed for the currently operating reactors which produce about 16% of the world's electricity. The gap is filled with stockpiled reserves, in large part obtained from dismantling old nuclear warheads. Raising mineral production to the level needed to satisfy demand is a huge and expensive task; even more if it were to occur together with the construction of new reactors. Whether we'll develop a serious uranium shortage in the near future is hotly debated, but the problem cannot be ignored (see, e.g. EWG 2007).
So, extracting uranium from seawater is a subject often discussed and, as we saw in the previous section, the amounts theoretically available in the oceans are more than sufficient to stave off all worries of shortages for a long time. Indeed, already in the 1960s, the idea had started to be evaluated (Nebbia 2007). The development of a membrane able to recover uranium from seawater (Vernon and Shah, 1983) was an important step forward and it led to experimental tests performed in the 1990s by researchers of the Japanese Atomic Energy Agency (JAEA). In these tests, a few grams of uranium oxide were actually recovered from the sea. From a web page dated 1998 (JAEA 1998), we see that these tests were performed in 1996 and 1997 and the results are reported in detail in a paper in English by Seko et al. (Seko 2003). Some results with braided fiber used as adsorbent are reported in a web page (JAEA 2006).
However, JAEA seems to have stopped all activity in this field, at least from what can learned from the examination of their site in English . There are no reports of further experiments, demonstration plants or of scaling up tests being planned. Something went wrong here, clearly, but exactly what? The question is complex, but we can try to answer it using the concept of energy return of the energy invested, EROEI.
From table 2 we see that we would need to process 2E+13 tons of water every year in order to produce enough fuel for the present fleet of nuclear reactors. Considering that the present worldwide production of nuclear energy is about 2.5E+3 TWh (terawatt-hour) per year (WNA 2007), we arrive to determine that the "energy density" of seawater exploitable by the present nuclear technology is about 1E-1 kWh/ton (one tenth of a kWh per ton). It doesn't look large but it is still much larger than the kinetic energy of the same mass of water moved by average strength currents (See note 2).
Now, in order to extract this uranium, there are two possible strategies: one is of actively pumping the water through the membrane, the other simply dropping the membrane in the sea and wait for the metal ions to migrate to the active sites. In both cases, energy is needed for a variety of operations: pumping, infrastructure building, moving the membranes, manufacturing them, etc. We don't have enough data for a step-by-step evaluation of the energy necessary but we can try an order of magnitude estimate by comparing with known processes.
Let's start with the first strategy: actively pumping water through a membrane. The process requires energy mainly because of the viscosity of water. This effect is described by Darcy's law which says that the energy required is inversely proportional to a parameter called "permeability". A finer membrane (e.g. sand) has a lower permeability than a coarse membrane (e.g. gravel). The permeability of a uranium extraction membrane is not reported in the available studies and it is probably not even known at the present stage. However, we can estimate the energy involved by comparing with a similar, known, process: desalination by reverse osmosis.
In reverse osmosis, seawater is pumped through a membrane that retains the dissolved ions; just as it would be done for uranium extraction. The energy involved in desalination by reverse osmosis is of the order of 2-4 kWh/ton; a value that includes all the energy used by the plant. For uranium, we would use membranes with a higher permeability, but the energy needed cannot change too much. If we take a value of 1 kWh/ton as a reasonable "order of magnitude" estimate, we immediately see that it can't be done. If what we can recover from the uranium contained in a ton of water is about 1E-1 kWh, it makes no sense to spend 1kWh/ton for the extraction, even if we could do that at 100% efficiency. This result is nothing new and there are other kinds of calculations that lead to the same conclusion (Schwochau 1984). Pumping water through membranes is so energy expensive that it can't be considered as a practical strategy for uranium extraction.
So, we are left with the second strategy: dropping the membrane into the sea and wait until currents or diffusion brings the uranium to the adsorbing sites. This method avoids the energy cost of pumping. Yet, it is also a less efficient way to use the membrane. As a consequence, we need larger amounts of membranes, a larger infrastructure, and we need to move the membranes in and out of the sea. All these are energy costs. We are looking at a complex and largely unknown process which is difficult to analyze in all its details. Nevertheless, we can try.
First of all, we can gain some idea of the size of the task. Dittmar (2007) has already noted that the task is huge, but exactly how much space would the adsorbing membranes occupy? We saw (see table 2) that we need to process at least 2E+13 tons of water per year. We also need a relatively shallow body of water, so that the infrastructure that carries the membranes can be anchored to the sea bottom at a reasonable cost. Now, consider the North Sea as a suitable area. It is a shallow sea (average depth less than 100 m) and it contains about 5E+13 tons of water. Assuming a recovery efficiency of 50% (which is probably optimistic), it means that we would have to appropriate the whole North Sea with adsorption structures in order to get enough uranium for just 16% of the present world's electric power production. For powering the whole world, we'd need the equivalent of at least six North Seas.
But it is unlikely that the North Sea would have sufficiently strong currents for sustaining uranium extraction for a long time. That is a problem which has not been studied in detail: where can we find currents strong enough to move the huge amounts of water we need?
Current strength is sometimes measured in "Sverdrups", a unit that corresponds to one million tons of water per second, or 3E+13 tons of water per year. So, one Sverdrup is almost exactly the flow of seawater that carries enough uranium for the present needs of nuclear plants. Some currents are reported to be much stronger than one Sverdrup. For instance, perhaps the strongest current in the world is the Antarctic Circumpolar Current (ACC) which carries about 135 Sverdrups. There is plenty of uranium being transported there. But the average depth of the Southern (Antarctic) Ocean is around 3000-4000 meters and the area is highly hostile to human activities. Anchoring there millions of tons of adsorbing membranes, together with all the processing facilities, is simply unthinkable.
Perhaps we could consider the Strait of Gibraltar as a more friendly environment where to find strong currents. Damming the strait in order to produce energy had already been proposed by Herman Sorgel in the 1920s with his concept of the "Atlantropa" dam. The dam was supposed to provide about 50 GW of hydroelectric power, a little more than 10% of the power presently provided by the nuclear industry today. The dam was never built; of course: it would have been a disaster for the Mediterranean sea.
Today, we seem to be a little more careful with these megaprojects, but still the Strait's current is very strong and we could appropriate a fraction of it for uranium extraction. The flow of seawater through the strait is about one Sverdrup , enough to satisfy our current uranium needs. Let's say that we could intercept 10% of it (and even that could have huge negative effects on the Mediterranean environment). In this case we'd need the equivalent of 10 Straits of Gibraltar just for satisfying the current needs of the nuclear fission industry and some 60 equivalent straits for raising production to match the present world's demand. Do we have the equivalent of 60 Straits of Gibraltar in the world? We can't say for sure that we don't; but of one thing we may be sure: the task would be colossal, devastating for the environment, and expensive beyond imagination.
All this doesn't mean that it is impossible to extract uranium from seawater in amounts comparable to our needs. But it gives us a certain perspective that we can use for the evaluation of the really critical parameter of the process: EROEI. The huge areas that we calculated to be needed bring us to compare uranium extraction to another industrial activity where large masses of materials are transported over the sea: oceanic fishing.
We have some data about the energy expenditure of the fishing industry (Mitchell and Cleveland (1993)) and we can estimate that the industry uses fuel for an energy of about 7 kWh for each kg of fish recovered. Another estimate derives from knowing that the total fish catch today is around 90 million tons (9E+10 kg) per year (FAO 2005) while the total amount of fuel used by the world's fishing fleet in 2005 is of some 14 million tons of diesel fuel (FAO 2008) (2E+11 kWh, considering that the energy content of diesel fuel is 43 GJ/ton). The result is about 2 kWh of energy per kg of fish landed. These are rough estimates that only take into account the fuel cost. Yet, it seems that fuel is the main energy expenditure involved in ocean fishing. So, if we take a midrange value of 5 kWh/kg, we can't be too far off in terms of the energy cost of extracting something from the open sea and bringing it back to land.
Now, if we want to use membranes for uranium extraction, it means that we have to carry the membrane at sea, submerge it for a while, raise it, bring it to land for processing, then back to sea, and so on. From the paper by Seko et al (2003) we see that we need about 300 Kg of membrane per kg of uranium extracted per year. We also read in the paper that the membranes were "pulled out of seawater using a crane ship every 20 to 40 days". In other words, the membranes have to be brought back to the elution facility every month or so. Recovering one kg of uranium, therefore, would require processing at least 3 tons of membranes per year. For the present worldwide uranium demand (6.5E+4 tons/year) we'd need to move 2E+8 tons of membrane every year. That is about ten times larger than the weight of the total catch of today's fishing industry. This is another indication of the colossal size of the task.
But the real problem is the energy involved. Using the ratio of 5kWh/kg that we calculated before for fishing, and assuming the yield and the conditions reported by Seko (2003) we can calculate a total energy expenditure of about 1E+3 TWh/year for the present needs of the nuclear industry. This is about the same as the total produced, ca. 2.5e+3 TWh/year. So, the energy gain (EROEI) is too low to be interesting.
Of course, there is a high level of uncertainty in this calculation. On the one hand, we need to consider that is possible to improve the efficiency of extraction process using braided membranes and working at higher sea temperatures (JAEA 1998, 2008). We might also build floating processing facilities in order to reduce the transportation costs. On the other hand, the calculation refers only to the fuel expenditures. To that, we need to all the costs for the infrastructure, for the chemicals used in elution, for the energy needed for recovering the species of interest and so on. We need also to consider that the membranes are synthesized starting from crude oil. Since there are no data available for how long a membrane could last in operation, we can't calculate how much oil would be needed, but surely it would not be negligible (see note 3 for an attempt of calculating this value).
We can conclude that there is a high risk that uranium extraction from seawater in these conditions would have an EROEI smaller than one. Very likely, it would be too low to be interesting. In practice, nobody will provide the huge financial resources needed to embark in such a task while that uncertainty remains. Moreover, investors are not likely to appear when they can't ignore that, at any moment, the development of an efficient fast breeder reactor would make their huge investments worthless. So, we don't know for sure whether the nuclear industry will be facing a fuel shortage in the near future but, if it does, the best bet to concentrate on conventional land mining and on developing more efficient reactors. Extracting uranium from the sea is not a practical possibility.
4. Lithium and the others
The case of uranium gave us the tools that we need for the evaluation of the perspective of extraction of all the other elements. First of all, we should consider lithium, which is more abundant than uranium in the sea and that could also be used as an energy source. The 6-Li isotope can be transformed into an isotope of hydrogen, tritium, which could be the fuel of a future generation of fusion reactors.
Fasel and Tran (2005) estimate that a water-cooled lithium–lead breeder blanket reactor of 1.5 GWe power will need 787 tonnes of lithium per year. This reactor could produce 12 TWh of energy per year. From the data of table 2, we see that for producing 800 tons of lithium we need to process 4E+9 tons of seawater. In other words the "energy density" of seawater in terms of fusion plants would be about 3 kWh/ton, more than an order of magnitude larger than that of uranium (1E-1 kWh/ton).
If efficient selective membranes for lithium adsorption can be developed, the energies involved in extraction would likely be about the same as for uranium, but we would need ten times less water for the same amount of lithium, hence ten times less energy. Extraction by active pumping would still be very uncertain in terms of EROEI, but with submerged membranes the task appears possible without destroying the North Sea or damming the equivalent of dozens of Straits of Gibraltar. Still, it would be a huge task and its feasibility remains uncertain. However, Fasel and Tran (2005) also mention the possibility of more efficient ways of using lithium in fusion reactors. So, we can conclude that the extraction of lithium as nuclear fuel from seawater cannot be proven to be feasible in terms of energy return, but it is nevertheless a process worth investigating.
Lithium is also an essential element for the new generation of batteries used in road vehicles. Tahil (2006) studied the availability of mineral lithium if we were to substitute the present vehicle fleet with vehicles based on lithium batteries. He concluded that we would face a lithium shortage. This is not a problem for the near term future, nevertheless it could become serious one day. From a look to table 2 we see if we were to get the present lithium mineral production by filtering ocean water through a membrane, we'd need around 1.5E+3 TWh which is 10% or the present world production of electric power. It is a very large amount but not an unconceivable one. Using submerged membranes, we would be able to substantially reduce that amount of energy, perhaps of one order of magnitude. However, according to Tahil (2006), we would need to step up lithium production of approximately a factor of ten if we were to keep up with the present trends of growth. That is clearly impossible using lithium extracted from seawater, at least as long as we rely on the present energy sources. Nevertheless, it is not impossible that seawater could be one day a significant source of lithium for vehicle batteries, provided that lithium is recycled and vehicles are built in such a way to be lighter and more efficient.
For all the other elements listed in table 1, extraction from seawater seems to be impossible or, at least, extremely difficult. Consider copper as an example. The total amount that exists in the oceans is about 50 times the current yearly production (see table 2). So, in 50 years we would run out of copper from seawater, even if we were able to filter all the water in the planet's oceans. But that is unthinkable, of course. Similar considerations hold for most metals of technological interest. The old dream of fishing gold from the sea remains just that: a dream.
5. Conclusion
Perhaps, one day, we might develop futuristic robotic facilities anchored to the deep sea floor. These machines would be powered by uranium extracted from seawater and would use marine plankton to manufacture organic "tentacles" for adsorbing mineral ions. Processing would be made in place and the recovered metals would be shipped to the surface in neat packages. But that looks like a dream of the 1950s, on a par with atomic planes and weekends on the Moon for the whole family. With the possible exception of lithium, the best we can conceive today is that mining the oceans could produce only truly "homeopathic" amounts of minerals, thousands of times lower than the presently produced amounts. In today's industrial system, such amounts would be useless. This result is true also for uranium, where extraction from seawater can't be seen as a solution for the present shortage of mineral uranium.
Adding together very large volumes of low concentration mineral resources easily leads to optimistic estimates of availability "when the market price will be right". But this optimism is misplaced. Eventually, it is the paradigm of the "universal mining machine" (Bardi 2008) that rules. It is not the absolute amount of a mineral resource that counts but, rather, its concentration. Extracting from low concentration resources, no matter whether dissolved in seawater or in the earth's crust, is so expensive in terms of the energy needed that it is beyond our possibilities for the present and for the foreseeable future.
_______________________________________________________________________
- Acknowledgement: I wish to thank Pietro Cambi and Joe Doves for their comments and suggestions about the energy involved in desalination.
- Notes
(1) data sources for the tables : seawater elements concentration from J Floor Anthoni (2000, 2006) www.seafriends.org.nz/oceano/seawater.htm. Oceanic abundance calculated assuming a total ocean volume of 1.3E9 cubic km. Mineral reserves are from USGS 2007 mineral commodities summary (http://minerals.usgs.gov/minerals/pubs/mcs/) except for uranium reserves which are from Energy Watch Group (www.lbst.de/publications/studies__e/2006/EWG-paper_1-06_Uranium-Resource...). All reserves are in terms of the pure element, except for Aluminium, iron, and titanium, given in terms of oxides.
(2) Comparison of the energy density of seawater in terms of fissionable uranium and as source of energy for underwater turbines . A strong sea current may move at speed of a few m/sec. Let's consider a representative speed of 4 m/sec and calculate the energy as 1/2mv^2. In this case, one ton of water would carry about 2E-3kWh, much smaller than the value calculated before in terms of uranium content (ca. 1E-1 kWh/ton). However, an underwater turbine could well have a better EROEI than the complex process of uranium extraction from seawater and utilization in a fission power plant.
(3) Tentative calculation of the energy involved in manufacturing membranes for uranium extraction . From the only work published in the international scientific literature (Seko 2003) we can infer that we need about 300 Kg of membrane per kg of uranium extracted per year. Trying an educated guess on the basis of the paper by Vernon and Shah (1983) we might assume that repeated immersions of the membrane would degrade its performance and generate the need for replacing it approximately every year. A Russian site http://npc.sarov.ru/english/digest/132004/appendix8.html says that the membrane can be "assumed" to be usable 20 times before it has to be discarded. If this is the case, it can be used for about one year and a half. Taking one year as the lifetime of the membrane, we would need to synthesize about 300 kg of activated fiber per year. Assuming an overall yield of 30% (again, an educated guess) for the synthesis process, we see that we need about one ton of crude oil in order to extract 1 kg of uranium per year. Since crude oil has an energy content of about 12 kWh/kg, we would be using some 12 MWh that, used in a high efficiency combined cycle gas turbine would produce about 6 MWh of electric power. One kg of uranium in a nuclear fission plant can generate about 40 MWh of electric power and, therefore, the process could have a reasonable EROEI of about 7. However, note also that, in order to obtain sufficient fiber for supplying enough uranium for the production of the total of the electric energy today, we'd need about 2-3 billion barrels of oil per year. This is a small amount compared to the present production (more than 30 billion barrels per year) but not negligible and would become more and more important as oil production dwindles down because of depletion.
References
Bardi U., Pagani, M., 2007, "Peak Minerals" http://europe.theoildrum.com/node/3086
Bardi U., 2008 "The Universal Mining Machine" http://europe.theoildrum.com/node/3451
Busch, M. Mickols, B, "Economics of desalination— reducing costs by lowering energy use" Water and wastewater international, http://www.pennnet.com/display_article/208957/20/ARTCL/none/none/1/Econo...
Dittmar M., 2007, "The Nuclear Energy Option facts and fantasies", Proceedings of the ASPO-6 conference, Cork, Ireland.
www.aspo-ireland.org/contentfiles/ASPO6/3-2_APSO6_MDittmar.pdf
FAO 2005 http://www.earth-policy.org/Indicators/Fish/2005.htm
FAO 2008, http://www.fao.org/docrep/009/a0699e/A0699E08.htm.
Fasel, D., Tran. M.Q., 2005, Availability of lithium in the context of future D–T fusion reactors. Fusion Engineering and Design 75–79 pp. 1163–1168
Floor Anthoni, J., (2000, 2006) Oceanic abundance of elements, www.seafriends.org.nz/oceano/seawater.htm.
JAEA 1998 Development of the Adsorbent at the Takasaki Research Laboratory http://www.jaea.go.jp/jaeri/english/press/980526/ref01.html
JAEA, 2006 "Confirming Cost Estimations of Uranium Collection from Seawater" JAEA R&D Review, http://jolisfukyu.tokai-sc.jaea.go.jp/fukyu/mirai-en/2006/4_5.html
Mitchell, C. and Cleveland C.J., 1993. "Resource scarcity, energy use and environmental impact: A case study of the New Bedford, Massachusetts, USA, fisheries. Journalof Environmental Management, volume 17, Number 3 / May, 1993, p. 305
Nebbia, G., 1970, "L'estrazione di Uranio dall'acqua di mare"
http://www.aspoitalia.net/index.php?option=com_content&task=view&id=192&...
OPOCE (Office for official publications of the european communities) 2000. Environmental signals, http://reports.eea.europa.eu/signals-2000/en/page017.html
Schwochau, K., 1984, "Extraction of Metals from Sea Water", Springer series "Topics in Current Chemistry" vol 124. http://www.springerlink.com/content/y621101m3567jku1/
Seko N., Katakai A., Hasegawa H., Tamada M., Kasai N., Takeda H., Sugo T., Saito K. 2003, "Aquaculture of Uranium in Seawater by a Fabric-Adsorbent Submerged System" Nuclear Energy
Volume 144 · Number 2 · November 2003 · Pages 274-278
Tamada, M.; Seko, N.; Kasai, N.; Shimizu, T., 2005
Synthesis and practical scale system of braid adsorbent for uranium recovery from seawater
FAPIG (169), p.3-12(2005) ; (JAEA-J 00045)
Tahil, W, 2006, "The Trouble with Lithium Implications of Future PHEV Production for Lithium demand" http://tyler.blogware.com/lithium_shortage.pdf
Vernon, F., and Shah T., 1983, "The extraction of uranium from seawater by poly(amidoxime)/poly(hydroxamic acid) resins and fibre Reactive Polymers, Ion Exchangers, Sorbents Volume 1, Issue 4, October 1983, Pages 301-308
WNA, World Nuclear Association, 2007, http://www.world-nuclear.org/info/inf16.html.
Prior comment from "thirra":
So, it looks like a long term nuclear industry will be dependant on fast breeder and thorium reactors. Scope for another article there.
Looking at the uranium part of your argument it is clear that the figures are entirely reasonable providing one uses fast breeder reactors or other more efficient designs.
Since that increases efficiency of burn manifold, and in any case it would make sense to further develop from the protoypes for such reactors which have been built first, as uranium prices are still very reasonable, you seem to have provided a neat proof that a solution of obtaining uranium from seawater works fine in it's basic figures.
The quantities needed of membrane and expanse would also shrink commensurately with the increased efficiency, and your point as to the desirability of processing the membranes on a platform at sea is a good one, and should be perfectly practical by the time we need to do so.
The scale of the operations will also be much reduced if one considers that in reality assessments which use assumptions like 'if all our power came from this source' are never going to happen in fact, and by the time we need to build facilities to extract uranium from the sea, which is probably after we have made use of thorium which is four times more abundant than uranium, then solar energy etc will be making a massive contribution to our energy needs, as will conservation.
You have confirmed that not only is there no current problem with uranium supplies, but we can have a reasonable game-plan with no apparent show stoppers to get a substantial contribution to energy supply from uranium for as fas as we can see into the future.
well, you seem to infer a little too much from my conclusions. If we have an efficient breeder reactor, conventional mining will be sufficient for a very long time and there won't be any need for mining the oceans for uranium. But it is a marginal point: the point is whether we'll ever have an efficient breeder reactor. But that is another subject
Russia has had a prototype breeder for many years, and are building a full scale one.
Molten salt reactors etc have already been prototyped as far back as the 60's, and what is involved in building them is well understood.
The main thing holding back more efficient reactors has been the low price of uranium and fossil fuels, and so considering that a relatively small exploration effort has greatly expanded known uranium resources and we know perfectly well how to build reactors to burn the more abundant thorium then very modest progress is required to reach an efficiency at which the extraction of uranium from seawater has a perfectly acceptable EROI by the time we need to do so.
So, as I previously stated, we have sufficient uranium and thorium for our needs at the moment, and as far as we know there are no show stoppers to prevent their making a major contribution to energy supply for many thousands of years.
Thank you for clarifying that in detail.
An alternative to extracting uranium from seawater would be to extract thorium from it, which is much more abundant and so would have a greater EROI without going to a breeder program.
The estimates given here for the abundance of thorium seem excessive, as they show it as 3,000 times more abundant in seawater than uranium!
http://environmentalchemistry.com/yogi/periodic/Th.html
http://environmentalchemistry.com/yogi/periodic/U.html
they nevertheless serve to illustrate that we could, perhaps, simply burn thorium extracted from seawater in CANDU reactors.
There is also a good understanding of how to increase the efficiency of CANDU reactors to very high levels.
I've tracked down better figures for uranium in seawater - the previous source I was suspicions of has it's decimals tangled.
Should be around 3ppm:
http://213.253.134.43/oecd/pdfs/browseit/6608031E.PDF
6608031E.PDF
This sounds reasonable, as thorium being around 3 times as abundant in the sea as uranium would tie in nicely with crustal abundances.
So by simply burning thorium instead of uranium, assuming the technology to get that from seawater is similar, which there seems no reason to doubt, the efficiencies Ugo suggests could be multiplies by 3 times, or maybe 4 if uranium could be extracted in the same process.
Since this has taken a just a few hours to work out, it does not seem as though any suggestion that this article proves the impossibility of ever obtaining all the nuclear fuel we need from the sea would be well founded.
David,
Your link says 3-4 ppb on p. 27, not ppm. This is in agreement with Ugo's table. On the other had, that figure is not better in any sense than what Ugo has given.
Chris
Sorry for the confusion - in any case, the point is that thorium is 3 times as abundant as uranium in the crust and seawater, if the decimal places would behave themselves.
Of course, no research has been done on the practicality of extracting thorium from seawater, as it is so cheap and abundant. if we do need to at some time in the future, decades away at least, at first blush it would appear that the economics of extraction and the EROI would be several times better than Ugo has suggested, even without assuming advanced burning technologies.
David,
I see the difficulty now. No, thorium is much less soluble than uranium: http://www.marscigrp.org/ocpertbl.html
Chris
Thanks for setting me straight on that Chris.
Scratch seawater thorium!
Good job there is plenty on land! ;-)
"simply burning thorium"
Burning thorium isn't that simple, I'm afraid. In short: There were (and are) several attempts to do this in pilot plants, but so far this wasn't successful.
My understanding is that thorium burn has been successful in CANDU reactors:
http://www.nuclearfaq.ca/brat_fuel.htm
If this is in error, perhaps you would supply better data.
Thanks.
I'm not an expert in this technology, but this was my conclusion I remember from a general research on this technology a few months ago. As for your weblink: Keep in mind that this document is from the Atomic Energy of Canada Limited, so the author may tend to have a rather optimistic look on the outcome. To be sure you should also check it with statements from neutral or sceptical entities.
I just grabbed the first one that came up on a list by googling, as it is, AFAIK, common knowledge.
If you don't like my link, do you actually have any links at all or information to support your statement?
Yes, of course, but from a certain point I concluded to discard this technology, so I didn't collect much data.
But if you find something that might convince me please free to post it right here or send me a short email.
Not a problem, and if I chance on anything authoritative I will forward it, as I have not bothered investigating as AFAIK it is not in question - incidentally the Westinghouse reactor is also supposed to be adaptable to burn thorium.
Perhaps it is worth pointing out though that I believe the conventions of this site, at least as I have observed them, are that when you are asked for your sources for statements such as you made you substantiate or withdraw - not liking the link I gave is on a different level to not having any backing at all.
I am not too bothered about it though, so unless I hear something from you which substantiates in some way your comment, I will continue in my previous persuasion.
I've spotted a more detailed discussion here:
http://www.world-nuclear.org/info/inf62.html
Thorium
You are correct in that not every issue is fully resolved especially the high cost of fabrication as thorium is cheap enough that reprocessing issues are hardly critical for the present, but the main reason for the lack of development appear to be the same as for all the more advanced techniques, that uranium is so cheap and plentiful at the moment that no-one has bothered to develop alternatives.
But to conclude from that that the difficulties are so severe as to warrant 'discarding the technology' as you have done is strange, and may perhaps indicate that you were in no mood to reach a favourable conclusion regarding the technology, IOW perhaps looking for an excuse to reject it.
Everything has some level of difficulty, and thorium technology is hardly unique in this.
Wasn't successful? I'm aware of two demonstrations said to be highly successful:
I think you've sucumbed to the hype here about the molten salt reactor. It did not have a breeding blanket. It did run on U233 for a little while.
Chris
what does breeding have to do with burning it? The U-233 bred from Th-232 burned just fine in both, and was bred nicely in Shippingport.
The MSRE did not require a breeding blanket to prove the system. The elements of neutron economy were proven in the reactors which made the U-233 for its test, and Shippingport demonstrated an all-up system in a converted LWR.
The MSRE is an example of the kind of reactors which
Do you propose that the U233 used was derived in a manner that was self-sustaining? If so, please provide details. Otherwise, stand corrected.
Chris
Define your terms, troll.
You're right, Chris.
The 1977-1982 Shippingport mini-reactor contained .55 tons of U-233 bred at a different breeder reactor and 40 tons of thorium to produce 235 MW thermal of power(60 MWe).
http://www.presidency.ucsb.edu/ws/print.php?pid=6972
If you want to evaluate the practicality of a thorium program you should look at India's nuke program which was required to use domestic thorium as fuel. They have 17 existing small(most <<500 MWe) mainly CANDU style heavy water reactors for a total for India of 3779 MWe, which is smaller than the largest single US nuke, Palos Verde and the largest nuke plant Japan's Kashiwazaki is 8500 MW.
The new generation of reactors for India are
uranium based and many of their current reactors
have been running half-loads due a shortage of uranium and are buying model Russian VVER-1000 design LWR instead.
They actually have a goal of 20000 MW of nukes in 2020. I believe they only think they can get there with uranium based technology.
Why is India ABANDONING thorium?
Well, they simply haven't been able to push the technology
and are still desparately dependent of uranium as I'm sure you can see as it is in the news(Singh-Bush agreement).
Thorium is certainly a fuel, but it currently will not satisfy nuclear cornucopians who are addicted to uranium whether they admit it or not.
http://world-nuclear.org/info/inf53.html
You are right to question the practicality of the thorium as a major nuclear program based entirely on thorium in India is switching to
uranium.
Rather than citing theoretical studies I'd like to hear the explanations for why this is happening in India from the thorium lovers.
Also CANDU heavy water reactors require much more heavy water than LWRs because deuterium is required for moderation AND cooling.
Abandoning the Nuclear Non-proliferation Treaty was a very grave error. One hopes that someone will have the guts to repudiate that move.
Chris
Russia current breeder reactor is a commercial size reactor 600 Megawatts.
It generates about 3800 GW·h/year.
Over 25 times bigger than Nevado One (one of the largest solar thermal electricity generators)
It is over 6 times more than all of the solar PV generated in the USA.
http://www.eia.doe.gov/cneaf/alternate/page/renew_energy_consump/table3....
http://www.world-nuclear.org/info/inf98.html
Construction has started on Beloyarsk-4 which is the first BN-800, a new, more powerful (880 MWe) FBR, which is actually the same overall size as BN-600. It has improved features including fuel flexibility - U+Pu nitride, MOX, or metal, and with breeding ratio up to 1.3. However, during the plutonium disposition campaign it will be operated with a breeding ratio of less than one. It has much enhanced safety and improved economy - operating cost is expected to be only 15% more than VVER. It is capable of burning up to 2 tonnes of plutonium per year from dismantled weapons and will test the recycling of minor actinides in the fuel. Further BN-800 units are planned.
Russia has experimented with several lead-cooled reactor designs, and has used lead-bismuth cooling for 40 years in reactors for its Alfa class submarines. Pb-208 (54% of naturally-occurring lead) is transparent to neutrons. A significant new Russian design is the BREST fast neutron reactor, of 300 MWe or more with lead as the primary coolant, at 540°C, and supercritical steam generators. It is inherently safe and uses a U+Pu nitride fuel. No weapons-grade Pu can be produced (since there is no uranium blanket), and spent fuel can be recycled indefinitely, with on-site facilities. A pilot unit is being built at Beloyarsk and 1200 MWe units are planned.
A smaller and newer Russian design is the Lead-Bismuth Fast Reactor (SVBR) of 75-100 MWe. This is an integral design, with the steam generators sitting in the same Pb-Bi pool at 400-480°C as the reactor core, which could use a wide variety of fuels. The unit would be factory-made and shipped as a 4.5m diameter, 7.5m high module, then installed in a tank of water which gives passive heat removal and shielding. A power station with 16 such modules is expected to supply electricity at lower cost than any other new Russian technology as well as achieving inherent safety and high proliferation resistance. (Russia built 7 Alfa-class submarines, each powered by a compact 155 MWt Pb-Bi cooled reactor, and 70 reactor-years operational experience was acquired with these.)
In China, a 65 MWt fast neutron reactor - the Chinese Experimental Fast Reactor (CEFR) - is under construction near Beijing and due to achieve criticality in 2008. There has been some Russian assistance in its development. R&D on fast neutron reactors started in 1964. A 600 MWe prototype fast reactor is envisaged by 2020 and there is talk of a 1500 MWe one by 2030. CNNC expects the technology to become predominant by mid century.
In India, research continues. At the Indira Gandhi Centre for Atomic Research a 40 MWt fast breeder test reactor (FBTR) has been operating since 1985. In addition, the tiny Kamini there is employed to explore the use of thorium as nuclear fuel, by breeding fissile U-233.
In 2002 the regulatory authority issued approval to start construction of a 500 MWe prototype fast breeder reactor (PFBR) at Kalpakkam and this is now under construction by BHAVINI. It is expected to be operating in 2010, fuelled with uranium-plutonium oxide (the reactor-grade Pu being from its existing PHWRs) and with a thorium blanket to breed fissile U-233. This will take India's ambitious thorium program to stage 2, and set the scene for eventual full utilisation of the country's abundant thorium to fuel reactors. Four more such fast reactors have been announced for construction by 2020. Initial Indian FBRs will be have mixed oxide fuel but these will be followed by metallic-fuelled ones to enable shorter doubling time.
======= from Wikipedia
Currently operating
Phénix, 1973, France, 233 MWe, restarted 2003 for experiments on transmutation of nuclear waste, scheduled end of life 2014
Jōyō, 1977-1997, 2003-, Japan
BN-600, 1981, Russia, 600 MWe, scheduled end of life 2010
FBTR, 1985, India, 10.5 MWt
Under construction
Monju reactor, 300MWe, in Japan. was closed in 1995 following a serious sodium leak and fire. It is expected to reopen in 2008.
PFBR, Kalpakkam, India, 500 MWe. Planned to open 2010
China Experimental Fast Reactor, 65 MWt, planned 2009
BN-800, Russia, planned 2012
In design phase
KALIMER, 600 MWe, South Korea, projected 2030
Generation IV reactor US-proposed international effort, after 2030
Gas-cooled fast reactor
Sodium-cooled fast reactor
Lead-cooled fast reactor
JSFR, Japan, project for a 1500 MWe reactor begin in 2010
It seems that these days we can't even get a conventional reactor that is efficienct enough to be economical. Lovins and Sheikh argue that the cost of new nuclear power is prohibitive and much higher now compared to superior alternative: http://www.rmi.org/images/PDFs/Energy/E08-01_AmbioNucIllusion.pdf
Our flirtation with breeders has mainly led to meltdowns. It seems very unlikely that breeders could overcome the issues already facing conventional reactors since their present poor circumstances are not yet primarily owing to fuel costs.
Chris
"Our flirtation with breeders has mainly led to meltdowns. " -- Reference please?
We have mainly focused on sodium cooled reactors for breeders. They populate the early portions of the list of nuclear accidents: http://en.wikipedia.org/wiki/List_of_civilian_nuclear_accidents.
Chris
There are 2 sodium reactor accidents out of 23 accidents listed there, neither of which appeared to hurt anyone or lead to major contamination.
Of those two accidents, one (1966) created no contamination outside the reactor vessel, and the other (1959) released an unknown amount of radioactive contamination (interestingly, the source does not support the claim of the wikipedia page that this release was "substantial", nor that 10 of 43 is "one-third"; some guy just arbitrarily re-wrote it to say that in April 2007).
Yes, sodium cooled reactors proved to be pretty unreliable so we don't use them now. Stupid design really since sodium burns in water.
Superphenix used to leak quite a lot of the stuff: http://query.nytimes.com/gst/fullpage.html?res=9B0DE7DA113CF936A25757C0A...
This video from Japan shows the results of one of these breeder accidents: http://www.youtube.com/watch?v=SiSqW6pFuR8
Basically, the things are very very dangerous.
Chris
You say that as if some evidence has show it, but no such evidence has been presented.
Are sodium-cooled reactors more or less dangerous than other reactors of similar development level (i.e., experimental vs. experimental)? We have not seen evidence of that, so we cannot reasonably conclude whether or not that is the case. You may be right or you may not be, but simply pointing to the existence of an accident involving a sodium-cooled reactor doesn't tell us one way or the other, as the number of accidents involving sodium is only a small fraction of the total.
So? From the sounds of it, leaking sodium didn't cause any damage or injuries.
Nothing is 100% safe, so the goal is to make the expected and worst likely cases as safe as possible. The risks of nuclear need to be understood in the context of 600,000 yearly deaths from air pollution, much of which is due to coal-fired power generation. Coal killed thousands in London in four days in 1952; compare that to 60 direct deaths from Chernobyl. Even counting all the eventual cancer cases, Chernobyl will still not match coal's toll from those four days.
The question is not "is this technology safe?", because the answer to that is "no", pretty much regardless of what technology you're talking about. The question is "is this technology safer than the alternatives?"
Importantly, "safer" has to take into account more than direct effects: it would most likely not be "safer" if all the world's coal plants shut down tomorrow - despite the hundreds of thousands of fewer pollution-related and mining-related deaths, the net result would probably be negative due to the societal disruption and loss of services such as refrigeration.
As I've pointed out elsewhere in the thread, our spending on nuclear power rather than renewbables is pretty much responsible for all coal related deaths in the last twenty years since we'd have more than replaced coal with the same level of spending on renewables. The opportunity cost of nuclear power is enourmous.
I invite you to investigate the history of the liquid metal cooled reactors. A large fraction have had meltdowns and others fires. This is not a very promising technology.
You seem to minimizing the direct death toll from nuclear power generation. A bias perhaps?
Chris
That doesn't make any sense. The technology for replacing coal with renewables wasn't available over the last 20 years, so how could spending on nuclear then be "responsible" for coal?
Your claim here is, on the face of it, kind of nutty. It requires a whole lot more explanation than you've offered.
No - reality is. There simply hasn't been much of a direct death toll from nuclear power generation, at least based on all the evidence I've seen. Go here for an introduction to the topic.
If you think I'm wrong, though, please by all means provide some evidence. If you believe the direct death toll from nuclear power generation for all time is higher than the direct toll for that one incident for coal, please provide some evidence showing that. As of now, all available evidence says the opposite.
Absolutely - I have a very strong bias in favour of evidence.
I can link again: http://www.repp.org/repp_pubs/pdf/subsidies.pdf
As you can see nuclear power was subsidised early and often but never produced electricity too cheap to meter and thus never displaced coal. We know for certain now that wind will be cheaper than coal and can thus displace it. The technology for today's wind might easily have been developed with the sort of subsidy nuclear has received much earlier and thus coal would no longer be in use.
Wind is now way ahead of nuclear in adding new capacity and will soon surpass natural gas, the current leader. We might have done this forty years ago and have been paying less for electricity all these years. Instead, we tried to pick a winner by putting all the subsidy into jee-wiz nuculur. Now coal mining deaths are on the rise again all because of this wasted detour with nuclear power.
All those comic books the government distributed in schools with propaganda for nuclear power: a complete waste. It has led to nothing but death and poisoning across our country. And now the pampered industry is so complacent that it can't even look to see if a problem it has already had is recurring: http://www.timesargus.com/apps/pbcs.dll/article?AID=/20080920/NEWS01/809...
There is no reason to have any confidence in the safety of nuclear plants in the US.
As to deaths from the one accident in Chernobyl, one estimate comes in at 30 to 60 thousand: http://www.greens-efa.org/cms/topics/dokbin/118/118559.torch_executive_s...
Other accidents have occured.
Chris
Quoth the troll:
Nuclear was also heavily penalized by the legal tactics of so-called "environmental" groups, many of which turned out to be financed by coal interests. The anti-nukes were objectively pro-coal.
Coal was also subsidized, with government research into variations such as MHD. Wind technology wasn't up to the job at the time; it cost upwards of 30¢/kWh in the early years, and hadn't a prayer of competing. It required advances in things like fiber composites to get where it is now.
If the external costs of coal were included, nuclear would have put it off the market 20 years ago and we wouldn't be having this discussion.
Wind has plenty of potential, but it's wrong to think that a system requiring materials and processes not developed until the 70's or 80's could have been competitive at the time with something feasible with techology of 30 years earlier. Only now is it closing that gap.
It takes some real illogic to pin the blame on a technology for deaths due to not using it.
Recent US energy funding analysis from Management Information Services. Which is more a more all inclusive study, looking at all energy and all kinds of support.
Europe went deep into feed in tariff support for wind and solar but they have not displaced their coal energy.
Chris, I really can't be bothered to get into yet another debate on the general subject of nuclear power, particularly since you have made it clear on many occasions that you are ideologically opposed to it anyway, and so though I spoke with the tongues of men and angels you would find another objection from somewhere, after having cherry picked your sources from those of like mind.
I am grateful to Ugo though for having clarified on a numeric basis why, with any reasonable assumptions of future technological progress, far less extreme than those assumed for renewables, we not only have plenty of nuclear fuel now but are likely to continue to do so for as far as we can see into the future.
For those who object to breeder reactors, there are other uranium burning designs possible, quite apart from the the thorium burning CANDU reactor.
I will continue to support the deployment of solar and other renewables as and when they become practical, but unlike others I feel that if we are in a shortage situation of low-carbon fuels, the thing to do is jolly well get on with it and build what we can.
David,
Why reply if you are not interested in discussion? I did not reply to you in the above comment. As Ugo points out, you have incorrectly interpreted his article. You seem to wish to persist in that error here. That's rather sad I think.
Chris
I replied so as to affirm the boundaries of what I was and was not prepared to debate.
Since Ugo's comments are relevant to the topic of this thread rather than being a more generalised discussion I will comment that although it is clear that although he wishes to infer from the data he has presented that the extraction of nuclear fuels is impractical, I am perfectly at liberty to draw a different conclusion from the same data that he has amassed, that form his figures either the extraction of thorium is likely to be practical, or uranium with modest advances in present reactors.
It is therefore clear that there is no misunderstanding of the case he wishes to present involved, but a different conclusion.
Well David, that seems just a little campy. But, I suppose you can continue to misread the article if you choose.
Chris
Just as you can and will continue to entirely misread what I write.
It is pretty clear. I understand very well what he is arguing based on the data he gives.
I disagree with his conclusions whilst accepting the data.
There is nothing very complicated there.
Dave, I think you miss the point, there are at least a trillion barrels of oil in the world but they get less and less affordable the more depleted the reserves get, so consumption will peak - in short, consumption of oil has nothing to do with oil reserves, but the affordability of reserves.
The same is true of uranium, thorium, indium, lithium, phosphorus etc etc the reserves have nothing to do with consumption it has to do with affordability - low concentration reserves will be less affordable than those in current use, so production will peak - production will NOT keep growing forever.
But most important of all, if the alternatives to what we are using now are less affordable than now we will use less than now - and less and less as time goes on, not the more and more you envision.
I think we are pretty close to peak nuclear power now just because the Price Anderson liability is now so large that a single payout could bankrupt the government. There might be an opportunity to privatize the risk using AIG as a vehicle but that won't help to delay the peak because the actuaries will want to close most of the Entergy plants owing to poor operations and a number of others besides owing to too high real estate values near the plants. http://www.theoildrum.com/node/4555#comment-411423
I don't think it will be fuel shortages that end the use of nuclear power but rather other costs which are too high.
Chris
I based my reply on the arguments Ugo made.
He found the EROI marginal for seawater extraction of uranium and the area needed large.
I pointed out that this was assuming present day efficiencies for reactors, indeed he specifically says that they would not apply to breeder reactors.
It is therefore clear that if we use either breeder reactors, which we have a good idea of how to do and working examples his figures prove that this would be entirely practical.
Other designs are also perfectly practical by the time we need them, as we have enough uranium to go on with, and in addition thorium is around 3 times as abundant as thorium in seawater as well as the earth's crust and so it seems that he has set our minds at rest about not only present supplies but future availability as we know perfectly well how to produce power with thorium reactors.
It boils down to the flow rate being just fine, according to Ugo's figures, given modest efficiency improvements in burn over the next 20 years or so.
Cost estimates for the production of uranium from seawater are available here:
http://jolisfukyu.tokai-sc.jaea.go.jp/fukyu/mirai-en/2006/4_5.html
4-5 Confirming Cost Estimations of Uranium Collection from Seawater
Uranium when burnt with any reasonable efficiency is such a dense energy source that it is extractable at very low concentrations, and so is thorium - note that I did not try to make the same argument for lithium, as I don't bother worrying about far out technologies like fusion which may or may not work, and if you need batteries there are a number of alternatives, one being zinc which Toyota is looking at as a replacement for lithium.
No way lithium could be economically extracted from seawater, but Uranium and thorium could be according to the best figures we have.
The reason we have not bothered is because there has not been a shortage of cheap uranium, the same reason as for the lack of reprocessing in the States, or the slow development of breeders.
Perhaps it is more productive to consider the numbers for specific resources, rather than going for broad semi-philosophical statements, like we will have to cope with less and less - presumably, the counter to that is some sort of faith in the inexhaustible nature of human ingenuity, which is not a statement which I would go along with or particularly useful.
If we are said to be running out, I want to know of what and why?
It appears that from the figures Ugo gives that either if we use thorium or modestly increase present uranium burn efficiency, we are in no danger of running out of nuclear fuel at any rate, whatever may be the case for lithium or phosphates.
There is no problem at the moment, and as far as can be calculated, likely to be no problem for the foreseeable future.
I believe he'd be infringing on your territory if he did. You have a history of doing this, as you did to me here.
I corrected him once as did Ugo, thus my remark. I corrected you once but you seem to have lost all sense of the thermal issues. 500 feet down? Isolated from the environment? A complete fantasy.... This is much worse than your insistence that home cogen is a more efficient use of gas than modern turbines. Sometimes you get the numbers wrong. Here you've completely missed basic physics. Every once in a while when I correct David, he thanks me. You might give that a try too.
Chris
Behind a multi-foot concrete barrier? Isolated from the environment? Standard practice since the 1950's. (Extracting heat is simple: you pump cold water INTO the concrete vessel through a pipe, and take steam OUT through another.)
But not good enough to satisfy the more paranoid among us, so the reactor should be put behind more mass but less physical distance. This provides isolation from anything less than a multi-megaton nuclear weapon, and allows the heat to be brought up to heat homes, businesses and industry with a simple thermosiphon loop.
I won't expect you to agree with this. Your ability to appraise physics goes out the window when something violates the tenets of your ideology.
Are you really serious that all you want to do is to provide heat? No electricity? I'm sorry if I've misunderstood you. In that case, this seems just stupid though not crazy as it seemed at first.
You can store solar energy in the ground at less depth for winter heat. There is no need to try to run a seasonal reactor. I guess you're just in it for the jee-wiz effect. Nothing practical in this I think.
Chris
No. Who would operate a system at 650°C or more just to provide space heat for houses? You have to be willfully blind to even think that (which I am sure that you are).
The point is that all heat engines create substantial waste heat, and that heat has value for many uses if it can be delivered to where it's needed. 500 vertical feet is a very reasonable distance; several miles horizontally is not.
The reactors would provide high-grade heat to gas turbines as the primary heat engines, driving electric generators. The cold side of the gas turbines would be cooled by water, generating hot water or low-pressure steam. This hot water and/or steam would be sent to the surface for various domestic or industrial uses. The heat exchangers would require no pumps, just gravity.
There are a number of engineering riffs on this theme I won't go into, for the sake of brevity and not giving you anything else you can maliciously misinterpret.
So, half the year you need a 500 foot cooling vent and the other half you need a complex array of miles of distribution plumbing.
Small reactors are not cost effective. That is why we use big ones. We don't put them in the ground because that is too expensive. And, they are hands on. You need access.
Also, your cooling system is going to need a secondary lower temperature system because heat will build up in the rock otherwise and make operation difficult. This stuff runs on delta T, not just T.
Finally, you are right there with the ground water. You are not isolated from the environment at all. In fact, your leaks may well be the worst kind, like those at Los Alamos, impossible in principle to clean up.
Sorry you are so thin skinned about this.
Chris
You are getting increasingly irrational and trollish. If you can't bring yourself back to rationality, I'll act accordingly.
Half the year you use steam for space heat, and the other half you use steam to run absorption chillers for your A/C.
You mean small PWR's are not cost-effective. PBMR's appear to have different economics. MSR's have many of the same engineering properties as PBMR's.
Massive forgings would require much larger tunnels to move. Small, modular systems would only require small ones. You could also group several reactors together to make a larger power station but with a much smaller maximum component size. This was mentioned elsewhere in this thread, but you're blind to it. Or trolling.
Your blindness is showing again. Nothing says you've got to plug up all the tunnels after you finish construction, you just put sturdy doors in them.
You've gone from blindness to nonsense. Reactor containment buildings do not need secondary cooling systems, and there's always cold water if you need it.
If you go down a ways from where I'm sitting right now, you reach a layer of rock salt; if groundwater went there, it would have dissolved away millions of years ago. It's both easy and profitable to mine, to boot; putting reactors down there would have a revenue stream before any equipment was installed.
You are just getting silly.
Perhaps you'll see the problem if I were to suggest dumping biochar down a 500 foot hole to burn for carbon neutral cogen.... Do you begin to see why your thing invites derision? Maybe not, maybe that is you next proposal. If so, please don't give me credit for suggesting it.
Chris
Quoth the troll:
If you're going to stop at biochar, terra preta is a better option than dumping it down a hole.
An ideologue like you will deride any competing concept, especially if it's superior (and thus threatens your own).
Actually, it was proposed some time ago, and I have independently proposed it both stand-alone 3 years ago and as part of a comprehensive solution (step 8), which was received with no small amount of enthusiasm at the time.
But none of this will affect your position, because you're either a blinkered ideologue or a worthless troll (or both).
I see no mention a 500 foot hole in your biomass proposal. Stop trying to be a weasel.
Chris
<E-P comes in after a long day in search of an evening's relaxation. He fires up the web browser and sets it to load a set of tabs while he fills a highball glass with ice. Returning to the desk, he's reaching for the drawer where he keeps the cherry cordial as he spies a trollish name in a reply. He stops in mid-motion and shakes his head. Returning to the bar sink he dumps the ice, then goes to the freezer whence he extracts a frosty bottle of 151-proof Sarcanol. He pours himself 3 fingers neat and downs half of it. Properly fortified, he returns to the computer.>
Quoth the troll:
Indeed, no 500-foot holes are mentioned anywhere. Obviously the depth of the hole is paramount and must be exact. It must be 500 feet; neither 499 nor 501 will do, let alone 1000 or 5000. And carbon can only be sequestered as biochar in those exactly-500-foot holes, not in any other form or in any other place. Why, if you tilled it into soil somebody might come along with tweezers and pull it all out to grill some hamburgers or something. The world climate could be completely wrecked by careless Americans preparing food over Labor Day weekend.
I bow to your superior perspicacity and humbly beg your forgiveness for my impudence.
<Sarcanol finished, E-P goes in search of ice to pick up where he left off.>
Reread when you've come to your senses to see why you're in a hole on this one.
Chris
Here's a crutch for that comeback.
The global "flirtation with breeder reactors" has generated several times more commercial electrical power than from global solar power.
27 years for BN-350 (probably 1000 GWh/year) probably 25,000+ Gwh total
27 years and counting for BN-600 (3800 Gwh per year) probably about 80,000+ Gwh
Superphenix connected for two years 7700 Gwh total
How are any of the issues that you are bringing up going to alter plans in Russia, South Korea, China and India ? Japan is going to go along for the ride with Russia because their domestic issues will probably prevent them from going as fast as they would like.
Russia being flush with oil and gas money has ensured the funding of their breeder reactor program. Russia wants to stay an energy power long term and nuclear and breeder tech is their plan. Whatever happens in US politics and policy is irrelevant to this result.
Looks like the contribution of energy or fuel from these is "homeopathetic." At least Superphenix was quickly shut down. As we know from experience, the russian program is built on high risk.
Chris
You can tut-tut the Russian program all you want, but their program has been running and generating power for five decades. It will be greatly expanded, as will the India and Chinese programs and probably South Korea and Japan.
http://cns.miis.edu/pubs/week/070522.htm
Plans call for BN-800 to be commissioned by 2012, and the work on the next fast neutron reactor, BN-1800, to start immediately after that. [1800MW version of a fast breeder]
http://www.eng.runtech.ru/node/354
BN-1800 would complete 2018-2020
The real world which includes Russia and India and China. The real world will have a lot of breeder nuclear reactors.
Nice article Ugo as it made me think. What about the concentration of anions in seawater? Do you have any information? I was specifically thinking of phosphate as a source for fertilizer.
I recall that mineral phosphate is currently being deposited in some places on the ocean floor, but this is the best info on it I could find with a quick search.
If phosphate is precipitating today, it is near saturation and should be relatively easy to recover.
test
My connection goes on and off.... let me see if I can answer this comment. Yes; there is of course a whole field in anion extraction. Just think of sodium chloride, the main mineral extracted from seawater. I didn't examine this area in my paper; it would have been too long. But indeed it is a good question: can we extract phosphates from seawater? I think the concentration is way too small to be feasible, but that should be looked at in some detail
Davemart,
What article were YOU reading?
(BTW, Ugo first rate material!)
Sure we have enough uranium for a couple of decades with our current reactors.
Davemart, PLEASE READ THIS.
http://www-pub.iaea.org/MTCD/publications/PDF/Pub1104_scr.pdf
Look on page 49. This is Peak Uranium for actual uranium reserves(REASONABLE accessible resources-RAR).
It peaks in 2026 with a medium demand! EAR-I(directly inferred undiscovered resources-geologically indicated is maybe 25% more) EAR-II(indirectly inferred undiscovered resources- is another 25%).
For the high demand case on page 58 the peak of RAR+EAR-I+EAR-II is in 2030(ignore SR)!
SR(Speculative resources-uranium in shales, granite rocks or phosphate ore) in black is equivalent to seawater uranium. It is a 'black box' considered by the IAEA as unrealistic.
The only way to extend uranium for more than one more generation is by fast breeders which have yet to be proven despite 50 YEARS of research.
Incidently, what will you do with all the LWR(light water reactors) when you need to MAKE fuel in breeders?
Fusion is totally unproven--the JET tokamak reactor only managed to produce 65% fusion energy out for all the electricity input and that was only for a few milliseconds!
Nuclear energy is simply not a solution for our energy crisis and if we persist in deluding ourselves by building more nuke plants we will simply exhaust a rare and dangerous source of energy to light and air condition office buildings, run computers, etc.
You need to put your 'faith' in something else.
(There is a huge amount of nuclear energy coming to us in the form of photons from the sun--thousands of times what we can get from nuclear reactors.)
marjorian: "fast breeders which have yet to be proven despite 50 YEARS of research." ?? Yet to prove economical is the only problem.
"Fusion is totally unproven" -- ??
I'm personally a huge proponent of solar thermal, see my posts on many sites, and personal investments. But "arguments" such as yours, apparently designed simply to spur on your opponents by confirming their opinions of "our side", are countering the objective more than anything.
lengould,
IOW, we should NOT refute their arguments because we will appear to be 'unfriendly'.
I disagree. I don't think Davemart is a deluded fanatic but only that he lacks information and a clear picture at what is going on.
The fact that something is unproven is not a signal to 'meet the challenge' but pragmatically
give real time constraints whether it should be investigated at all.
Let's assume that we can perfect a breeder reactor with a regeneration ratio of 1.
That means we have enough fuel so that the first charge will be completely changed into fissionable fuel, even though this is illogical--all reactors produce some nuclear waste by decay to non-fissile elements.
Now that is fine for that particular reactor but then there's no fuel for other reactors, what happens to them?--400 or so commercial nukes have to shutdown when U-235 is exhausted.
The maximum regeneration rate theoretically possible is 1.6 by the fission of plutonium atoms.
So if we chose to fuel all those LWR(light water reactors) non-breeders with fast breeder produced fuel we will need a huge number of breeder reactors which mainly produce fuel not electricity.
Yet the technology for +1 breeders has not been successful and whats more the nucler industry is not pursuing it.
They want to REPLACE LWR reactors with liquid metal breeder reactors with a regeneration rate of 1.0, proposed advanced burner reactors(ABR).
These plants will use far less fuel than LWR, but will still produce some nuclear waste from decay and are very highly radioactive in operation.
An ABR is a totally different animal than a LWR.
But ABR do nothing for the existing 400 commercial power LWRs. So ABR doesn't address the problem of LWRs, the source of all nuclear power running out of fuel.
At this point the thorium people jump in and say that they will breed thorium to U233 and feed that to the LWRs. This has been done, but the implication is again a huge number of breeder reactors build not for power but for making fuel for LWRs.
All we want is electricity and the nukers want to sell us huge new fleets of nukes to REPLACE what we have now. It is not sustainable.
They will produce a lot of electricity since most of the energy released is in the atom nucleus fragments from the fission and not in the neutron that gets absorbed by U-238.
Hi marjorian,
Glad to know you do not think me a fanatic!
Actually, I just think we should get on with things and produce energy in the handiest practicable way, and not fanny around with remote theories based on speculative projections.
Although by no means an expert, I have taken the time and effort to keep fairly well in touch with energy issues, so any misjudgements I may have made are unlikely to be due to a lack of information.
I simply do not understand some of the interpretations you are putting on the data.
As calgarydude pointed out, the reference you gave did not support in depth the construction you were putting on it, and was in any case rather old data, the latest shows a considerable increase in projected supplies.
On your point that the 400 present LWRs will need replacing, that will happen anyway as they age, and, for instance, in the US if modern twin 1.6GW reactors were used to replace them , then without any more sites around the total baseload of the US could be generated.
As for thorium reactors, what on earth makes you think that they would be purely to breed uranium?
Without going into the minutiae of the fuel cycle, which neither I nor presumably you, are qualified to do, you don't have to run dedicated reactors in great numbers to transmute the thorium, and power is still produced whilst they are breeding.
Your comments in another post regarding the difficulty and expense of supplying heavy water seem to take no account of the fact that the latest designs of CANDU, precisely because heavy water is expensive, use around 1/10 as much as older ones, as they have a separate cooling circuit not employing heavy water.
It should also be borne in mind that no technology which produces lots of power is entirely clean and pollution free - solar cell production in China, for instance, recently had a scandal when a lot of nasties were released.
That is without taking into account that in practise if you don't use nuclear at the moment, you burn coal - look at Germany, which is a very rich country which has been trying hard to move to renewables, is still effectively coal and oil powered - with the remaining nuclear plants, of course.
As Marx might say, objectively speaking to be against nuclear energy has always been to be pro coal, and that is about as deadly and polluting as it gets.
So really, although I return the compliment and regard you as no fanatic, if I am honest it does seem to me that you are arguing from a prior position, and what is more one which allows a great deal of leeway to what renewables can do - a realistic assessment shows that currently in actually running a large part of a modern economy they are not at an advanced enough state to do that at the moment - if they move on a lot in five years, great, reassess.
It also appears that you have rather fastened upon any negative reports on nuclear power, whilst heavily discounting the positive, and in fact not being very current in your information, presumably because you have already made a judgement.
I have nothing against renewables, but they need realistic evaluation and using where appropriate and economic whilst proceeding vigorously with their development.
Meanwhile I would much prefer not to freeze, which in Britain at least seems likely in the next few years due to opposition to building nuclear plant, and there is no way on God's green earth that enough power can be generated using only renewables at this latitude and with this high a population.
Most of the people who advocate it seem to dislike BAU and consider that we should contemplate loads of people dying with equanimity, as it will return us to the path of righteousness and nuclear is ...dangerous!
I don't think you are that terminally silly, but I think the same as you in reverse, that you have not really kept up on what is the score now.
Here is support for my comments on the inability of renewables to provide enough power for the UK:
http://www.withouthotair.com/
Sustainable Energy - Without the Hot Air (withouthotair.com)
Please note that this deals only with the physical constraints - if the realistic economics are considered the idea becomes even more absurd.
Everything should be investigated, the only question is what should be relied on when making plans.
For example, I am in favour of fusion research. It seems very promising, and even if they can never make a reactor achieve a self-sustaining reaction for more than six seconds, many useful scientific insights and technologies will likely be developed from the research.
But I wouldn't say, "hey, keep burning all our fossil fuels as fast as we can, no worries, we'll have a fusion reactor some day."
Likewise with extracting minerals from seawater, breeder reactors and so on. At present they don't seem to be practical, they're horrendously expensive, require large amounts of resources, and some of them have great dangers to humans and the environment.
If they were all we had to choose from then of course we'd have to go for them. But we have other choices, things which are more practical, not as expensive, don't require as much resources, and are far less dangerous to humans and the environment.
The main thing to remember is that there is no single technology which will solve all our problems for us. And even if there was, it wouldn't be put in place all by itself. The best solution is a mixed solution, with a variety of technologies put in place - and even if it isn't the best solution, it's what we'll get.
No country is going to build 500 nuclear reactors in 20 years, or 100 square kilometres of solar photovoltaic cells, or dam Gibraltar, or drive its people out of the cities and into self-sustaining eco-villages. Nor is anyone going to build a super global electric grid. It's just not going to happen.
What will happen is a mix of things. So the only question is the exact make-up of that mix. When we build solar PV, solar thermal, "clean coal" plants, light water nuclear reactors, and so on, will it be more of one or the other? When we recycle more minerals, will we begin recycling them now, or wait until we run short and start mining landfills? When cities face water shortages, how much will people reduce waste, and how much will they start collecting their own water, how much will they drink and wash in wastewater and enjoy cholera? And so on.
It's easy to come up with grand plans reducing the world's complexity to a simple equation. I call it the "XY=Z Syndrome", where we try to reduce all human issues to some X times Y = Z equation, X wheat yield times Y wheat eaten = Z population we can sustain, X uranium concentration in seawater times Y extraction = Z uranium we can get, X kilometres walked per person times Y less heart attacks = Z lives saved. And so on.
But the world just isn't that simple. We muddle along with very mixed solutions and problems.
Actually, my case for nuclear is based purely on known reserves and present technology.
At the very least it would provide a breathing space to enable the maturation of the renewable technologies so that they could begin to approach the practicality which is being erroneously claimed for them now.
It is also current technology, the CANDU reacor, and I believe the Westinghouse, to be able to burn the far more abundant thorium.
In contrast if one froze the debate so that only present technologies and resources could be considered then you would have to discard solar PV and geothermal for a start.
Actually I make no such harsh assumptions, and think that solar PV will be making a very substantial contribution to our energy needs by around 2015.
It is nevertheless the case that far less radical assumptions need to be made in the case of further progress in nuclear technology to envisage it's continuance for many, many years into the future than for renewables, which need enormous technological leaps in many areas, notably storage.
My understanding is that not all reactors would need to be of the fast breeder type, as they would produce fuel for others.
In any case, CANDU and other thorium burning designs could also be used.
It is easy to come to a conclusion which is negative if you make the most unfavourable assumptions for nuclear power on the one hand, and the most favourable and extreme extrapolations of current technology for renewables on the other.
If you really, really want to base your case for renewables on current technology and practises that is fine - but the fact that a substantial proportion of total electricity is generated by nuclear and a tiny portion by renewables with the exception of hydroelectricity will give some idea of the outcome.
Critical to resource estimation is anyway the consideration of what price is paid for it. The raw materials for the fuel in nuclear reactors is in any case a tiny proportion of total costs - processing costs far more - and so could rise many fold without materially affecting total energy costs.
Simple re-processing such as is done in France would greatly stretch supplies in most places, such as the US.
In the case of the UK, from depleted uranium to hand we could run a substantial nuclear program for around 60 years with re-processing, so I am not too concerned with all of these supposed long term problems which are trotted out interminably, always with the proviso that all the technology to deal with them should be available right now, whilst the alleged alternatives are supposed to effortlessly and almost magically advance technically.
So even taking the lowest estimates of nuclear fuel availability and assuming no technological progress at all, it is well worth while building reactors.
Of course, if a fraction of the progress in improving efficiency that is routinely assumed for renewables is taken into account, one arrives at much more realistic estimates of the likely ongoing vast contribution of nuclear power.
David,
It is very clear that new nuclear power is so expensive that it poses and unacceptable opportunity cost. You can read more here: http://www.rmi.org/images/PDFs/Energy/E08-01_AmbioNucIllusion.pdf
Chris
I am well aware of the costs of nuclear power. Also I am aware of the cost of renewables.
I am perfectly happy to build whatever is most economical and practical at the time and place, or preferably conserve.
You OTOH have made your opposition to nuclear power on ideological grounds perfectly plain, which does not put you in the best place to impartially assess their relative merits.
majorian,
I suggest that you re-read the report that you cited.
The gist of the report (IMHO) is that if we anticipate a rapid worldwide build-out of nuclear reactors, then we should start proving up additional resources as soon as possible - that is, doing additional drilling and assays to quantify the extent of resources instead of just guessing.
After reading the entire report, I don't have any disagreement with it. It doesn't talk about some absolute upper limit for future uranium production - they are talking about the next four decades.
Companies spend money to prove up resources when only they are sure that they can sell them at a high enough price and within a reasonable time period. If they underestimate demand, they can be caught short with inadequate capacity. This report suggests that they may be underestimating the coming demand.
BTW, CANDU Power Reactors have been in operation since 1957.
Thorium
IMHO, even without *any* new technology (eg breeders, molten salt, etc), we aren't going to run out of nuclear power in this century.
Calgarydude,
Ah, the heavy water reactor(CANDU) will save us.
So your solution is for world to replace 400 LWR(light water reactors) with heavy water reactors which have a totally different design.
There are 29 such reactors in the world and most of them are smaller than LWRs.
One problem is that they require thousands of gallons of very expensive heavy water which is toxic for animals, though not radioactive.
Again, a solution that builds lots of nuclear plants but doesn't make a lot more electricity.
(Like the Red Queen in Alice in Wonderland, running faster just to stay in place.)
I never talked about replacing anything.
You are assuming a major leak. A leak of almost any inducstrial liquid in common use (eg liquid chlorine, ammonia, benzene, toluene, gasolene, etc) will kill wildlife. Engineers make every effort to ensure that this doesn't occur.
CANDU is simply one solution which is currently in production. For seven years, when I lived in Toronto, most of my electrical power came from CANDU nukes: 24/7, rain and shine, and during the most severe periods of winter.
What I don't understand is how you can criticize technology which has been providing practical electric power in real-world settings for many decades.
Baloney! And it doesn't matter which way you slice it, it is still baloney.
Whow, this would be the first time an industry admits the peak of its own base resource. Just unbelievable!
I had a second look to this (very interesting and detailed) report - and in fact as far as I see they don't see it the way we tend to see it:
1. The shortage issue depends on the level of uranium demand:
"Production from high confidence RAR is projected to be adequate to meet all requirements in the low demand case." (p.3)
2. The IAEA doesn't see a peak but sees it as the good old market-will-do-it problem. In fact their "alarming" can also be interpreted as a PR activity to encourage investments in "their" uranium mines: "However, if significant and timely exploration is conducted and sufficient resources are discovered, there could be an adequate supply of lower cost uranium to satisfy demand."
So from a cornucopian's point of view nothing new.
I think some of the difficulty with mining metallic ions in seawater could be bypassed by doing some selective breeding on various seaweeds.
Many already have metallic ions in their membranes many, many times higher than seawater itself. Since plants are self renewing and powered by solar energy, the EROI should be greatly improved for all but the rarest and, obviously, most toxic metals...
I find it odd in fact, that this article isn't about this angle rather than using artificial filters, which seems intuitively impractical to me even before mathamatical analysis is done..
It is an old idea, it was even the plot of a Donald Duck's story that I remember having read many years ago. But there is also plenty of serious literature on the subject. Might work, but I am skeptical about the EROEI. The artificial membranes developed for uranium extraction are highly sophisticated and extremely efficient. And yet, the volume of water to be processed is gigantic and the associated energy is large as well. With seaweed, we'd probably need even larger volumes, and to transport immense amounts of seaweed back and forth. And then, how do you get the metals out? You'd probably need to dry and burn the seaweed. It seems hugely complicated to me. But....
I guess it just depends on how much concentration is possible in the seaweed. This is the unknown that drives tha whole EROI issue. It would also vary greatly I'm sure, dependent on the inherent toxicity of the ion desired, to biological systems...
Toxicity can be managed, as cells sometimes build special proteins that bind toxic metal ions so that they will not endanger the rest of the cells. That is also the reason for most heavy metal ion accumulation by some organisms like algae
Are you sure you're not thinking of the Beagle Boys using a large bell which rings at just the right frequency to cause gold to settle out of the water onto a long carpet they're trailing behind their boat? (The story ends with the bell being cracked and rendered useless by a harpoon.)
I am sure it was Donaldd Duck. They went with uncle scrooge trying to extract gold out of seaweeds, but of course it didn't work. I think I remember that they used that seaweed to make a weight loss potion!
Sort of like the ancient Roman method of purifying gold by flushing whole mountains in Spain out into valleys where they grew a plant species which selectively took up gold. This sounds like a very rational approach. Perhaps the harvested biomass of the seaweed would cover the energy input requirement, or more. Always a good thing to have ash recovery being the primary driver of a boiler system, then you know none will escape as pollution.
Would the economics shift if the recovery was co-located with desalination activity?
We see countries investing in desalination plants to provide fresh water for their populations. It would seem possible to combine mineral extraction with such activity and lower the economic threshold for recovery. Is this a potentially viable undertaking?
Thanks for the article, Ugo.
I had been thinking about that, too. What you are proposing is to extract minerals from brine. As far as I could say; I don't see any advantage in terms of energy; what you would be doing is first passing water through a membrane, and then through another. You shouldn't gain anything, probably lose something. But that is in terms of energy; in terms of money you'd have clean water as a byproduct so, perhaps that could make the process interesting in some cases. But there are no data and no tests available; so it is impossible to say
You would also need a very large demand for the clean water. In essence you would really be doing no more than mining dirt. If the brine were economic, we'd be strip mining salt flats now.
Chris
The term "homeopathic" here is beautiful.
In fairness, there are only a few of the strange-love-for-nukes crowd who propose this in the absence of a dangerous breeder economy. This also seems to be the main out when they combat Storm-Smith: nuclear power will be better in the future so it is not correct to describe nuclear power as it is today.
Thanks for carrying out these calculations.
Chris
The main "out" when discussing Storm-Smith is that the silly handwaving done leads to an overestimation of the energy costs of mining uranium by two orders of magnitude when compared to real world, e.g. the Rössing mine in Namibia.
Storm-Smith already disposed of that silly claim. When you cherry pick your examples (e.g. you mine in Nambia), you skew your estimates compared to what is reasonable for the general case. The general case is required in order to estimate how soon nuclear power collapses. You should not deceive yourself so.
Chris
Hey, guys. Someone ought to inform Stormy et al. of this discovery in northern Canada. These guys stumbled onto a field of boulders just laying on the surface which assayed on average 1.3% Uranium. There's areas up there that have never been seen by humans, much less been evaluated to any depth for minerals.
"The Mistamisk Boulder Field is approximately 500 X 250 meters in size and
contains many radioactive boulders. In a Virginia Mines report, 70 boulders assayed an average of 64.9g/t Au and 1.3% U (with values up to 640 g/t Au and 4.11% U)."
http://www.secinfo.com/d12MGs.u8y.htm
http://www.uraniumstar.com/news/pdf/070608.pdf
Very poor academics.
Assuming it eventually becomes efficient enough to be usable, what effect could large scale adoption have on the ecosystem in the ocean? And it seems like yet another way to treat a symptom instead of the illness. Sustainability should be the ultimate goal of humanity, and on all fronts, not just energy.
I thought I had commented on this comment, but somehow my answer doesn't appear. Sorry for taking useless space; I am testing whether my connection works..... there it goes!
All right, my connection seems to work, now. By the way, I am in a Hotel in a place called Trollhattan in Sweden. They have a wonderful hydroelectric plant here; and lots of trees, too. You can produce energy without destroying the environment!
So, about the question of damage to the environment, yes, of course the size of the plants needed for extracting anything out of the sea would be a disaster for the marine ecosystems. I said that extracting uranium for the present needs would mean appropriating an area about 6 times the North sea; I didn't comment on that being devastating for the environment but it seems pretty obvious. Just think of the damage done to marine species by oceanic fishing, and minerals extraction would be on a scale order of magnitudes larger.
What I have been thinking, however, is that had the EROEI been good, the North Sea would be already gone, and probably the Strait of Gibraltar as well!
There are many parts of the sea which have very little growing in them, because they are deep and there are no upwellings of nutrients. If those could be put to use, the impact might be positive.
Those deep places with no upwellings of nutrients would be cold waters, and cold water lowers the yield. Which has implications for economics and EROEI. Only surface waters would be interesting, considering practical issues with going deeper, and the higher temperatures. Perhaps the warm currents of the thermohaline bands can be used, but the impact on climate and ecosystems would have to be assessed.
It does look like substantial improvements in the performance of the membrames is very possible. More research programmes are definately justified.
Great article. I think DaveMart raises valid points about breeders, but I also agree that breeders would make seawater "mining" irrelevant. I don't know how much depleted uranium we have stockpiled but if we have stored everything from enrichment, that would suffice to power fast breeders for tens of thousands of years. We could stop uranium mining altogether.
Majorian talks about "photons from the sun". Forget about it, EROI is not good enough for renewables and the practical problems are too large. We couldn't sustain more than one or two billion people using renewables. Breeders are currently our best and only hope, and as DaveMart points out, it is a technology that is quite well understood and definitely feasible to implement. Combine with HTE - high temperature electrolysis - to get hydrogen and then convert it to methanol to power the world transport infrastructure. Clean, environmentally friendly and actually possible to do full scale.
EROEI for wind is about 20:1, and for modern solar is about 15:1. Poor EROEI is not a valid argument against renewables.
This claim requires support.
Depleted uranium?
There are 470000 tons of the stuff in the US waste from making maybe 50000 tons of enriched uranium fuel and nuke weapons. Add this to 50000 tons of spent fuel rods to get maybe 520000 tons of potentially fissile 'waste'.
A kilogram of natural uranium in a fast breeder will produce 6660 Mwh(thermal) or 2000 Mwh(electricity).
520000000 kg x 2000 Mwh/kg = 1040000 Twh of electricity
which is about 260 years of current total US electrical production of 4000 Twh.
Applying this to the whole world, 3.4 million tons of RAR uranium would provide power at the CURRENT rate of 14000 Twh for 4900 years( which ignores the demand for US lifestyle worldwide which NO cornucopian can deny!).
Add in 50% for all EAR undiscovered but probable estimates( but leaving out speculative fantasies) plus add in 300% for probable thorium deposits and you get 30.6 million Twh.
If the whole world ends up using 50% of the average americans electrical consumption and the world population stops at 9 billion people at 58500 Twh per year that should last the planet about 530 years.
No way does this add up to tens of thousands of years.
OTOH, 200000 square miles(516000 sq. km-size of Texas) of nice clean solar PV at 1 Mwh per m2 per year(insolation), .1 Mwh electricity would produce
51600 Twh per year of electricity FOREVER.
Oh and BTW, your breeder technology doesn't even WORK.
:-p
In the end, the nuclear power industry, including the NRC, can't even seem to handle cooling water all that well: http://www.timesargus.com/apps/pbcs.dll/article?AID=/20080920/NEWS01/809...
Supposing that they could manage sea water for mining seems a stretch even before it is pointed out that the whole concept is untenable.
Chris
I've been waiting for a breeder reactor since the 50's----
What seems to be the problem?
Newly mined uranium is too cheap.
That's too simple an excuse. Fast breeders are more capital intensive, more complex, less reliable, larger chance of substantial core damage. Those things do not sound good to investors. Investors rather put their money in proven and safe technologies like lightwater reactors. Also, breeders have suffered from very strong social resistance (due to safety perceptions), as well as a perception of thermonuclear weapons connexions.
Fast breeders were intended to solve minor problems, but as time passed by, it was perceived (so far correctly) that solving these minor problems would exagerbate the major problems. In short, fast breeders have been penny-wise, pound-foolish.
That's not to say breeders shouldn't be researched further. The LFTR is technically interesting so I wouldn't mind a couple billion in a crash development program. It's a bit of a stretch, though, to argue that we can 'start' with a 100 GW of thorium reactors, as the Engineer-Poet puts it. First we have to get a 100 MW or so, then see how it works technically and commercially. Right now there isn't even 100 miliwatts.
It worked beautifully with 7.4 megawatts of LFTR, and 60 megawatts of light-water thorium breeder reactor. The only reason we haven't had tests of 100 megawatts and up is that the technology didn't advance the economic interests of the nuclear industry at the time.
I humbly suggest that the situation may now favor this technology.
7.4 MW thermal yes. No 'tricity, capt'n. No Braytons were available at the time.
I wouldn't assert that Brayton cycles (He or otherwise) are commercially mature enough right now for a 100 GW(e) plan. There is no evidence to support such a claim. Most of the engineering issues appear manageable though; if not, perhaps ultracritical steam cycles have to be considered.
More importantly, the design proposed by Sorensen and others deviates on many fields from the initial 7.4 MW(th) experiment. Lots of nuclear engineering is unsolved and the LFTR still remains a paper design. Of course there will be technical issues with the nuclear part of the system. There always are with new designs.
This new information by Ugo is rather discomforting. On the other hand, the lack of data available means we can't jump to conclusions just yet. For one thing, the rapid advancement of absorbent technology over the last decade still holds promise, especially long-term.
You are right though, a breeder looks desirable. But may I suggest it be thermal (slow) breeders? If fast breeders, then please NOT sodium primary coolant systems. They are not the brightest idea. Something like the fluoride thorium or chloride uranium breeder is what's needed in a couple billion $ crash program. Private investment is absent, they don't want to invest in a promising but risky design which wouldn't generate any revenue before 2020 at least. If all goes well (exponential growth) your 100 GW(e) target might be met before 2030.
There are a lot of other technologies that can't get private/bank funding but are technically interesting. These could be included in a crash RD&D program as well.
Brayton cycle not ready?! Are you kidding? There's about 395 GW of gas-fired electric capacity in the USA, and probably 90% of that is Brayton-cycle or combined-cycle plants. Existing turbines run under far more stressful conditions (1100+°C turbine inlet temp, oxidizing conditions) than a plant running helium in a closed loop at 650°C hot side.
Even adding a breeding blanket would be a substantial deviation.
We've got chemists and laboratories and materials tested under years of neutron bombardment in other reactors. We certainly have enough experience to be able to design a prototype, build it and start working out the bugs. We should do that right away.
If you'd prefer something like the Shippingport reactor thorium test writ large, I'm game. But, being PWR technology, this has the same issues of availability of large forgings preventing rapid construction and relatively low thermal efficiency. We'd be much better off with liquid fluoride, because we could begin by reprocessing and burning all of our used PWR fuel as the starting charge for a thorium cycle.
That is 395 GW of combustion gas turbines. Non-combustion gas turbines are not proven. They're mostly in pilot/research stages. You say that the conditions in non-combustion lower temp operation are more reasonable than in higher temp combustion gas turbines, but the fact that they are not commercially competing with Rankine steam cycles, even in the higher temperature regimes, should caution us not to trivialize the engineering/commercial issues.
I agree with everything else you say.
You ignored the 600MW Russian reactor for the last 20 some years and did not watch the French and other country breeders that were operated for a many years then were stopped.
Watch what the Russians, Chinese, South Koreans and Japanese do with Breeder reactors.
Thanks Mr. Bardi for performing an analysis, much more thorough that I and I'm many others had the time to perform. I had started to work with some of the figures that were coming out of Japan and they seemed to indicate scale factors that when considered operationally would have been formidable. The larger perspective on the ion concentrations and the EROEI boundaries for the process that show that this is not an energy source with current technology is invaluable.
Thanks for resolving concretely something that appeared in my gut to be the case on initial examination. Excellent work.
Let me add my thanks to those of koob; Nice work again Mr. Bardi!
Well, thanks!
Japan is still pursuing uranium from seawater.
I have an article on the latest proposal which has been translated to english.
The secondary alternative is that 195 million tons of uranium is recoverable eventually based on the Japanese plans of accessing the 4.6 billion tons in the Oceans. [seaweed would mainly be grown and harvested in shallow coastal waters. Energy of growing and harvesting offset with both biofuels and uranium]. Japan uses 7589 tons of Uranium per year now. 40% of Japan's consumption would be about 3000 tons of Uranium per year. So there is the reserve amount recoverable and the annual production based on the planned scale of the initial operation.
The 2001 IAEA paper referenced by Majoran is outdated.
Current conventional uranium reserves are 5.5 million tons (up 17% from last year). There is another 10 million tons expected to be developed in the same geological formations. There is 22 million tons of Uranium in phosphate reserves. So 195 million tons would be a lot, but it would only be part of the 4.6 billion tons in the oceans. 65,000 tons per year of uranium are used worldwide now.
Uranium from seawater can last twenty thousand years supplying one hundred times current world energy usage and five billion years at current energy usage levels [when river runoff is considered]
Russia has fully funded its breeder reactor development program for a 800MW system to go along with the 600MW system which has been running since the 1980s. S Korea and Japan are interested in the Russian breeder technology.
China continues working on breeders and factory mass produced high temperature (HTR) nuclear reactors. The HTR's will be over twice as efficient with nuclear fuel as current reactors.
Hyperion Power generation has a deeper burn factory mass produced uranium hydride reactor, which has letters of intent for ten unit sales. They expect to have commercial deliveries in 2013.
Australia's anti-uranium mining government has lost and the Australian uranium mine projects are proceeding.
Namibia's uranium deposits are larger than originally thought and expanded production there will be coming online soon.
Brazil's nuclear company is pushing forward expanded and aggressive nuclear power plans. UK's nuclear power projects are proceeding.
http://www.mri.co.jp/COLUMN/TODAY/KATORI/2007/0214KY.html
http://babelfish.yahoo.com/translate_url?doit=done&tt=url&intl=1&fr=bf-h...
======= previous info on the braid adsorbent system (the genetically engineered seaweed appears likely to be targeting a similar area for deployment)
http://www.iupap.org/wg/energy/annex-1b.pdf
In 2002 and 2003, the uranium adsorption of braid type adsorbent was evaluated in the sea of Okinawa area. The average ability of the adsorbent became 1.5 g‐U/kg‐ad for 30 d
soaking. The nonwoven fabric adsorbent in the size of square with 2 cm one side attached on the braid type adsorbent showed 3.0 g‐U/kg‐ad. Temperature of the seawater in Okinawa was 30 °C (10 °C higher than that of Mutsu area). The rise of 10 degrees in the seawater temperature increased by a factor 1.5 the uranium adsorption for the nonwoven fabric adsorbent( square size with 2 cm side). Therefore, the braid type adsorbent had 2 times higher adsorption ability of uranium in seawater than the stacks of nonwoven fabric adsorbent ,due to the better contact between seawater and adsorbent.
The Environmental Science Research Laboratory, the Central Research Institute of Electric Power Industry reported that 1,200 t‐U/y could be collected from seawater if the 2.1 millions braid type adsorbents, 60 m length, were set in 134 km2 sea area with an interval of 8 m. Since the higher temperature of seawater accerelate the uranum adsorption, the sea area should have the Japan Current, the depth from 100 m to 200 m, and no fixed net. The sea site (6000 m2 ) proposed for the recovery of uranium from seawater is located between Nansei islands and Tosa bay.
The recovery cost of uranium, by using stacks hanging system, was estimated at 400‐
500 $/kg‐U in the following conditions: The scale of uranium recovery is 1,200 t/y. The
adsorbent can collect 6 g‐U/kg‐ad for 60 d soaking. The adsorbent stacks can be repeatedly used 20 times.
Ugo :
No comment on Japan's genetically modified seaweed for biofuel and uranium from seawater project ?
No comment about regular mining projects which seem likely to raise production to 88,000t-117,000 tons by 2015 ?
btw: Congrats on getting your uranium from seawater article published at the Oildrum. When I submitted my uranium from seawater article to the editors it was ignored. I guess I had to say that uranium from seawater was not going to work. [I noticed that you would comment on congratulations so maybe you can also reply to the data and links while replying to this congrats]
This is a comment from Ugo Bardi:
Advancednano, thanks for all the links that you sent. I'll comment only on the one about seaweed which is the only one relevant to my post.
So, from the link from Mitsubishi it is very interesting to see that the Japanese have indeed abandoned the idea of using synthetic adsorbants for fishing uranium from the sea. They must have followed a line of reasoning similar to mine. Conclusion: 50 years of work and a lot of hype, but it just does NOT work. I guess by now everyone agrees on that.
How about seaweed? Well, note that the Mitsubishi proposal is just that. The Japanese are often very creative in their projects; but creativity doesn't mean it will work. With seaweed, we are entering a completely new ground; starting basically from scratch. In my opinion, they'll be facing the same kind of constraints that have doomed extraction using synthetic adsorbants: colossal amounts of water and of adsorbing stuff to be processed and - consequently - huge amounts of energy. If they were reasoning in terms of EROI they wouldn't embark in these tasks. But, on the other hands, if people reasoned in terms of EROI they wouldn't do the stupid things they do.
Sorry for the delay in answering, I have troubles with my connection and I hope that someone at TOD can relay this message for me.
I do not see evidence that the adsorbant concept was abandoned as unworkable.
If the seaweed concept has gained preference over the absorbant process it appears that they feel that the seaweed concept is superior for biofuel and for uranium. Plus there is the issue of how much regular uranium there is and how much is in phosphates to indicate when a better uranium from seawater project would need to be deployed.
In terms of colossal amounts water - The adsorbant is just left in the water where there is current. So the only relevant part of the energy calculation is for moving any adsorbant in and out of the water. If this was an issue for efficiency, there could be more processing of the adsorbant in place or nearer to where the adsorbant is hung.
Ugo Bardi said:
This appears to be a totally unwarranted and unsupported conclusion.
The costs which they have estimated for uranium from seawater are higher than present prices for mining, so there has been no reason to go into production.
Because uranium is cheap and plentiful is hardly a reason to infer that if the price rises somewhat then this will not work.
It is also completely unwarranted to put words into Mitsubishi's mouth that they 'agree that uranium from seawater does not work' as they are clearly still investigating a number of alternative processes, and would not bother if they felt it impractical.
It therefore appears that the people who actually know more about the process than anyone else, having paid for and undertaken experiments, as opposed to assembling an article based on others work, feel that it is an option worth pursuing, although with the plentiful and cheap supplies of uranium that we currently have there is plenty of time to optimise the precise technology to be employed.
I'd like to point out the fact that Japanese are always looking for ways to gain energy independence, just like the US, and it's a relatively hot topic debated these days in a country with virtually no oil/gas/uranium reserves. Mitsubishi's interest in this field could be due to government grants, national publicity, or other financial benefits and not squeezing uranium out of the same waters they drill for methane hydrates.
Gentlemen, please, look at the facts.
1) The Japanese started a research project on the extraction of uranium from seawater using synthetic adsorbant polymers in the late 1990s. They concluded it in 1998.
2) Some papers were published in the early 2000s, but no further research is reported to have been performed in this field up to now
3)In 2008, Mitsubishi proposes to start again from scratch with uranium extraction, this time using seaweed instead of synthetic polymers.
4) Conclusion: they have understood that extraction of uranium usyng syntetic polymers doesn't work as a source of fuel for nuclear reactors.
You are doing well until point 4.
There are no grounds for concluding that that is their reason.
With the price uranium was in the early 2000's, no extraction industry would be interested in powering on with developing alternative more expensive technology.
You are replacing the universal behaviour of these industries with an hypothesis of your own with no evidence at all - indeed, contrary to the evidence, as if they know the attempt to extract uranium from seawater is impossible, why have they re-started research?
Clue: slightly rising uranium prices and rapidly expanding construction of nuclear plants makes it worthwhile, and in the interim they have discovered another approach which may be even better, and that their earlier research gave them grounds to think it worthwhile.
5 million tonnes times 17/117 is 725,000 tonnes, about 2,000 tonnes per day; thermally equivalent to 200 million barrels of oil per day.
But this is based on the periodic IAEA "Red Book" reports, as excerpted by people who can afford to buy them, right? The periodicity with which they come out is two years, not one, as I recall.
So high-grade uranium has been being prospected faster than oil has been getting burned, but only a little faster, not twice as fast. And the rate of prospecting is about ten times the rate of use, not 20 times.
--- G.R.L. Cowan, author of 'How fire can be tamed'
Eherm, we seem to have taken a major detour into the politics of nuclear with some of the answers above -even though it was just being used as an example: back on thread...;
The idea of using Seaweed sounds good -seaweed is currently used as fertiliser -possible mass replacement for NPK?
How about bio-engineering algae? OK, this is scitech at the mo -but it might be possible to make algae that not only had high fatty-acid content to turn into liquid fuels but also the 'ash' might contain -by design- high concentrations of Lithium, Uranium, etc. I have no idea how this might be achieved -ask Craig Ventura...
OTECs shift vast quantities of water, indeed they need to to produce power. Lithium production has been demonstrated in conjunction with this technology (although I do not have any figures on costs): Xenesys would eb a good starting point.
I guess my point is that what is really needed is a shotgun blast of R&D into this sort of stuff combined with a level headed approach to evaluating the practicality, scaleablity and impacts of a solution. We should only look to the recent food-chain-bio-fuels debacle to realise that a holistic approach is required.
Regards, Nick.
This might be of interest
Water Desalination International, Inc. will unveil the Passarell VES process. A desalination process separating potable water from seawater by a way of distilling seawater. A subsurface intake process, leaving a wet crystallized salt eliminating waste brine returned to the sea and thus preserving the environment. The processing of seawater into drinking water is costly but now with the VES process the price as low as $.30 per thousand gallons is available far below reverse osmosis processing. Extra income benefit are obtained from the crystallized salt through the commercial marketing, lowering the cost of drinking water. Soon-to-be operating in Saudi Arabia
Frank
http://213.253.134.43/oecd/pdfs/browseit/6608031E.PDF
(from pages 26, 27)
4.2 million tons of Uranium in black shales in Chattanooga USA and Ronneburg Germany.
Lots in Monazite coastal sands
Uranium mining forecast
World Uranium mining can reach 117,000 tons per year by 2015.
there is a list of countries and mines that is fairly comprehensive out to 2012.
Hi Brian,
Unusually for you, I could not find where your figures for the Uranium mining forecast were sourced - have I missed it?
the uranium mining forecast was from the Uranium 2008 report (joint report of the IAEA and OECD). I linked to the 400 some page report (read only copy).
The forecast is page 48, 49 of the report.
Thanks Brian.
This new report gives 5.5 million tons Reasonably Accessible Resource + Inferred and 10.5 million speculative. It's really about the same as the 2005 report I linked to.
I came up with 3.4 RAR plus 50% for Inferred(5.1 million tons) plus 9 million tons speculative.
http://www-pub.iaea.org/MTCD/publications/PDF/Pub1104_scr.pdf
How big is 5.5 million tons of uranium oxide?
It is a cube 209 feet on a side. This is not an inexhaustable source.
Nothing is changed the peak will be in 2024 or 2030 and then production will decline.
Still no breeder reactors, still no reprocessing, no granite or shale with uranium traces, still no thorium to the rescue.
Don't buy this charade.
Nukes are too expensive and will soon run out of fuel.
----------------------------------------------
The most curious part of the thing was, that the trees and the other things round them never changed their places at all: however fast they went, they never seemed to pass anything. 'I wonder if all the things move along with us?' thought poor puzzled Alice. And the Queen seemed to guess her thoughts, for she cried, 'Faster! Don't try to talk!'
Not that Alice had any idea of doing THAT. She felt as if she would never be able to talk again, she was getting so much out of breath: and still the Queen cried 'Faster! Faster!' and dragged her along. 'Are we nearly there?' Alice managed to pant out at last.
'Nearly there!' the Queen repeated. 'Why, we passed it ten minutes ago! Faster!' And they ran on for a time in silence, with the wind whistling in Alice's ears, and almost blowing her hair off her head, she fancied.
'Now! Now!' cried the Queen. 'Faster! Faster!' And they went so fast that at last they seemed to skim through the air, hardly touching the ground with their feet, till suddenly, just as Alice was getting quite exhausted, they stopped, and she found herself sitting on the ground, breathless and giddy.
The Queen propped her up against a tree, and said kindly, 'You may rest a little now.'
Alice looked round her in great surprise. 'Why, I do believe we've been under this tree the whole time! Everything's just as it was!'
'Of course it is,' said the Queen, 'what would you have it?'
'Well, in OUR country,' said Alice, still panting a little, 'you'd generally get to somewhere else—if you ran very fast for a long time, as we've been doing.'
'A slow sort of country!' said the Queen. 'Now, HERE, you see, it takes all the running YOU can do, to keep in the same place. If you want to get somewhere else, you must run at least twice as fast as that!' -Through the Looking-Glass
------------------------------------------------
Well, Marjorian, you have plainly moved from a numbers backed debate to declarative statements, and haven't even attempted to look at points which were raised regarding the fact that the so-called unrealistic breeder technology has produced far more power than your speculative projections of what solar 'might be able' to do, at some point in the future - because it sure doesn't now.
We presently have nuclear technology, which produces a reasonably large portion of the world's electricity demand.
Solar is a bright idea, not presently doing much of anything at any substantial scale and at large, heavily subsidised cost, and with substantial engineering difficulties before it can do much better.
If you wish to imagine that simply assuming that all problems will be overcome to produce your preferred result, then that is up to you, but no present realistic costed engineering proposals are possible to run most of society on renewables.
The technology, aside from considerations of density an storage, simply is not mature enough.
It is a pretty odd gamble to make with the fate of the world, aside from the huge damage already done by CO2 releases and pollution from what has been the actual, as opposed to desired, alternative to nuclear, coal.
It is a waste of time to try to counter faith-based belief systems with rational arguments, and so with no personal disrespect involved I will now discontinue the debate.
DaveMart - "Solar is a bright idea, not presently doing much of anything at any substantial scale and at large, heavily subsidised cost,"
However let us not forget that the most heavily subsidised energy industry is in fact the nuclear industry whose total subsidies over the years completely dwarfs any investment in renewable energy. Even in Australia a report on getting a nuke up and running here could not see any way to do it without a 30% handout from the government.
Also for one who diparages declarative statements this is a classic. Because you cannot kill people with solar energy of course it has not had the same investment as the commercial excuse for the truly massive expenditure required for nuclear weapons programs. Solar energy because it can not be used as a weapon has lagged in investment and is years behind nuclear power. This does not mean that it can't do it as you seem to imply. BTW with this new found interest in breeder reactors that can save the world I assume that any country in the world can have them? Or are they only for trusted nations?
"If you wish to imagine that simply assuming that all problems will be overcome to produce your preferred result, then that is up to you, but no present realistic costed engineering proposals are possible to run most of society on renewables."
And in exactly the same way you are imagining that the huge engineering bottlenecks in the nuclear industry from steel forgings to certified welders will simply disappear so that this new fleet of nukes can be built in time to save us and give us your preferred result. Notwithstanding the already insurmountable problems of nuclear proliferation that the world is having enough trouble with, added to the rougue states with nuclear weapons and the fact that so far only a small fraction of the world's nuclear waste is actually stored safely in long term storage sites you want to add to the problem by mixing in tons of weapons grade plutonium that on the scale needed to produce sufficient power would be completely overwhelm the already insufficient resources of the Atomic Energy Commission.
All this so that you can think you yourself that when I want a light on, I want it on no matter the cost or the problems involved. Try reading about the new smarter renewable grids that will supply a large fraction of current demand without leaving a dangerous legacy for others to clean up. Yes we will have to make some changes to the way we do things for this to happen however over all humanity will become more efficient and more resilient to natural disasters.
I don't back off of the numbers!
The amount of insolation is 1 Kw/m2 in the USA and most areas of the country easily get 1000 kwh per year from that m^2, in the UK it is slightly less at 875 kwh/m^2. The efficiency of PV cells is actually much higher than 10%. The scale is huge but so is the scale of low EROEI
fuels.
http://en.wikipedia.org/wiki/Solar_cell
For a perspective, high grade ore is 20000 ppm of uranium, lowest grade ores are 1000 ppm and things like the Chattanooga shales are 60 ppm, or certain coal deposits are 300 ppm or phosphate rocks are 100 ppm or granite is 4-30 ppm,etc. Therefore you have to mine 10 or even a 1000 times the ore just to get a decent day's production plus there are losses in all mechanical operations.
Underground mining will be impossible, imagine gigantic openpit mines covering hundreds of square miles just to extract the same amounts of uranium available in commercial grade ore as in the present mines.
It is almost inconceivable that these speculative 'resources' will be developed in the next 50 years.
Peak uranium is approaching according to the IAEA.
The IEA puts the peak in a couple of decades. After peak, more and more reactors will be closed as fuel production slows.
Show me a working 1GW of average hourly output solar plant which is in any way affordable, together with the storage system and means to make up for the relatively low winter solar incidence at any non-equatorial region, and you will have some sort of serious proposition.
You are not comparing working systems which can be ramped up to produce substantial amounts of electricity anytime soon.
You are comparing an idea with an actual, proven large scale power generation system.
You also seem to be entirely unaware that most uranium is extracted by leaching, not open pit mining, or that the very substantial requirements for steel and concrete for wind power and solar would involve large mining operations.
Anyway, discussion is pointless, as your objections are not of a nature which is open to persuasion.
In contrast all I require to be keen on renewables in a particular location and climate is some evidence that it will do the job on the right scale and cost.
I really can't understand how any intelligent person would seek to compare what is still basically a bunch of not too well thought out theory and supposition with a real-life, working reality.
David,
Since 3.8 GW of solar PV was produced in 2007 and growth was 50% http://www.earth-policy.org/Indicators/Solar/2007.htm , presumably the ~6 GW we might expect this year will count as new solar capacity with 1 GW average production. Will there be any new nuclear capacity in 2008? There has been none through the beginning of September: http://www.thebulletin.org/web-edition/reports/2008-world-nuclear-indust...
In fact, we've been having fewer rather than more reactors over the last five years.
Hitching your wagon to a declining industry seem like poor judgement. Perhaps if you realize that it is a dying industry, you'll stop the pointless promotion. In any case, it does not seem to be able to compete with solar now in new capacity or price and if fell behind wind quite some time ago.
Chris
Sept 08 439 reactors generating 373,247MW
http://www.world-nuclear.org/info/reactors.html
Jan 08 439 reactors generating 372,059MW
http://www.world-nuclear.org/info/reactors-jan08.html
1188MWe added from uprates.
10,000 GW-h. More than solar addition even without new reactor completions.
http://www.world-nuclear.org/info/inf17.html
2008 will be a slow year in terms of reactor completions.
Existing Nuclear power can be uprated. Over 2008-10 EdF (France) plans to uprate five of its 900 MWe reactors by 3% [135Mwe, 1.1TWh/yr]. Then in 2007 EdF announced that the twenty 1300 MWe reactors would be uprated some 7% from 2015 [1820MWe], within existing licence limits, and adding about 15 TWh/yr to output.
Spain has a program to add 810 MWe (11%) to its nuclear capacity through upgrading its nine reactors by up to 13%.
The USA will be adding about 2 GW of power via uprates over the next 4 years.
3 more nuclear reactors are starting up in 2008 (hopefully only 2 as the third reactor is Iran's)
7 reactors in 2009 (5200MW)
7 reactors in 2010 (5200MW)
7 reactors in 2011 (6600MW)
9 reactors in 2012 (9075MW)
16 reactors in 2013 (17120 MW)
This includes Watts Bar 2, 1180 MWe reactor is expected to come on line in 2013
47+ GW added by 2013.
350+ TWh
Brian,
The plants in India don't seem to have their fuel supply lined up yet. http://www.business-standard.com/india/storypage.php?autono=333970&chkFlg= India has been reducing generation because of a lack of fuel. In any case, these are smaller plants and won't equal the additions of solar capacity this year. You should also be aware that some plants will be shutting down as time goes by.
It is time for you and David both to admit that nuclear power just can't scale up the way renewables can.
Chris
Most nuclear plants are getting operating extensions so shutdowns are being pushed out for decades.
The 40,000+ GW-h/year of new nuclear power from new reactors and 10,000+ GW-h/year from power uprates from 2009-2011 while keeping pace with solar are years where the nuclear industry is scaling up for big production. And by 2018, there should be two or three factory mass produced nuclear designs (Hyperion, China HTR, Russian FBR) and the AP1000 will be virtually mass produced for China.
Factory mass produced nuclear reactors appear likely to start commercial deployment in 2013 from Hyperion Power generation Plans so far for three worldwide factories and a production of 4,000 of the initial 27MWe designs. Initial orders (actual customers) starting to be taken. These 4000 nuclear reactors (each the size of a hottub) would in total generate more power than the current US nuclear deployment.
The AP1000 already has a factory for major components in China (the factory was built in 11 months) and several more will be built around the world. The AP1000 will have partial mass production. China is upsizing the AP1000 to 1600MW. Current batch is 1250MW, Next batch is 1400MW. Build times will head to 3 years as process improves for the 100 China intends to have built or in the process of constructing by 2020. China's current target is 70GW of nuclear power online by 2020.
China will factory mass produce their high temperature reactor (about 200MW each) first one to complete 2013. Build times going to 2 years.
Russia's factory production design is the Lead-Bismuth Fast Reactor (SVBR) of 75-100 MWe. An integral design, with the steam generators sitting in the same Pb-Bi pool at 400-480°C as the reactor core, which could use a wide variety of fuels. The unit would be factory-made and shipped as a 4.5m diameter, 7.5m high module, then installed in a tank of water which gives passive heat removal and shielding. A power station with 16 such modules is expected to supply electricity at lower cost than any other new Russian technology as well as achieving inherent safety and high proliferation resistance. (Russia built 7 Alfa-class submarines, each powered by a compact 155 MWt Pb-Bi cooled reactor, and 70 reactor-years operational experience was acquired with these.)
A lot of large forging makers
http://nextbigfuture.com/2008/09/soon-ten-or-more-large-forging-makers.html
More powerful uprate technology
http://nextbigfuture.com/2008/09/annular-fuel-50-power-ultra-uprate-to.html
Nuclear power will be taking the new clean power lead back from wind power by 2015 and from now to 2020 will add more power than wind and solar.
http://nextbigfuture.com/2008/08/nuclear-power-will-be-added-faster-than...
Brian,
All I can say is you must really love a police state. The stock of donut companies is going to go through the roof with all the guards needed for those little reactors.
For years the nuclear power industry has been all promises and no delivery. It is almost a sure bet that nothing on you list will be on time. Thus, you projections seem unlikely to be accurate.
Chris
Nuclear power is delivering 2800 billion kwh/year.
Solar and wind is delivering less than 280 billion kwh/year combined.
What is your projection for solar and wind based on what will happen in the real world.
In the last couple decades there was a relative lull in nuclear power support and there was billions/year for feed in tariffs in Germany and Spain etc...
http://nextbigfuture.com/2008/01/energy-costs-with-externalities.html
China current nuclear builds are ahead of schedule.
You obviously do not like the current reality and the reality that will happen. But it will happen anyway.
I support building more solar, wind, geothermal and nuclear and hydro. But I look at the reality of the projects that are funded or are strongly being pursued to project the expected energy mix.
Putting them 500 feet underground would eliminate the need for the guards, but the mental compartmentalization forced on you to maintain your ideology against attack from self-contradiction will prevent you from considering these two ideas at the same time.
Some day I expect to hear a loud, distant "BANG!" and later learn that it was your head exploding.
Chris, I have no idea why you imagine it is nuclear power or renewables.
They are complementary as both are rather good at filling in for the weaknesses of the other.
It is a lot easier to build a solar power system in a hot sunny area, for instance, if all or most of the baseload is taken care of by nuclear.
Conversely, in the same scenario the nuclear works a lot better if the peak air conditioning needs are taken care of by solar.
Building clever combinations of resources reduces costs many-fold, and makes a lot of otherwise tough engineering problems a lot simpler and less costly.
A massive nuclear build would not mean that any less renewables would be needed, as the demand is so huge - but it would mean that more of them would be sited where it would do most good.
It really is a little perverse of you to wish to stick so many of your solar panels where the sun don't shine!
David,
As I've pointed out before, the opportunity cost of nuclear power is much too high. If we'd put as much effort into renewables as we have into nuclear power we would not be burning coal today. This is an enormous price to pay for a failing technology.
Chris
Love your theories, Chris. Most of the power that is non-fossil fuel is actually nuclear, and facts trump your theories.
Still, I am wasting my time trying to discuss it with you, as you are committed to some all-renewables dream regardless of reality, or local conditions, or local availability of resources, or anything else, for ideological reasons.
Do you know how I know I am right? Because I change my mind when new evidence comes in.
There never is any question of you changing your mind or amending your beliefs.
That is the definition of a faith, which just picks up and uses odd bits of rationality as they happen to be convenient.
David,
Then be prepared to change your mind. The leverage of subsidies for the development of wind is enourmous. Not so for nuclear power. Had we invested as much in renewables as we have in nuclear power, we'd be coal free today, http://www.repp.org/repp_pubs/pdf/subsidies.pdf and paying less for electricity.
This is a clear example of the huge opportunity cost of nuclear power. Nuclear power is responsible for all the coal related deaths that we've suffered in the last 20 years. If we come to power shortages and deaths, it will be nuclear power that is responsible for our lack of abundance.
Chris
It did not happen. So it is meaningless.
If a lot more was spent on nuclear then it to could have displaced coal buildup as was done in France. Germany spent the most on wind but still is looking to build more coal power. So even your meaningless "what if" is wrong.
What is a going forward plan that will be implemented ?
David,
I should not let this pass because I think it will help you to point this out. The reason you are often forced to change your opinion is not because of new evidence. It is because you make things up in the absence of evidence and asert them as true. The human mind is prone to work this way and it takes training to avoid this pitfall. But, do not take the fact that you require fairly frequent correction as evidence that you are correct more often than others. In fact, you are playing with a handicap. If you have read "The Tailor of Panama," what you have is fluence as the term is used there. This is a useful gift, but it does have its drawbacks if you are not careful to separate what you imagine to be true from what you know to be true. A habit of taking exernal correction when pushed to it is not quite as useful as applying intellectual rigor to your thoughts before you speak them so that there is less frequent need for external correction because internal correction has already been achieved. Then it is more likely that what you have to say will be a contribution rather than a distraction.
Chris
!!! You are certainly an expert on that, Chris! I rather admire your powers of invention, and the legal profession has possibly missed it's brightest jewel in your ability to distort straightforward evidence, you are perhaps one of the few who could out-do the counsel for O J Simpson in this respect.
David,
I tried to say that softly, but still I did not expect graceful acceptance of my point. Still, it may sink in after a while and help you. I am speaking from experience of the sorts of errors I have corrected for you. I may be wrong in my interpretation of the orgin of the errors of course.
Chris
That is a good point that hopefully DaveMart will accept. That it isn't all in one power plant shouldn't detract from it. It could be argued that the distributed nature of last year's 3.8GW nameplate is better than a single power plant.
DaveMart's comment of solar not doing much and at heavily subsidised cost may have been true a decade ago but does not apply today. Hundreds of MW are being installed today, by private companies, unsubsidised - the relative costs between solar and fossil fuel is only going one way.
In some equatorial regions, Sub-Saharan Africa for example, PV is totally cost completive against oil. Given that European latitudes only receive 3-4 times less insolation we are well within an order of magnitude of cost competitiveness. With PV prices falling some 18% with every doubling of global production and oil prices rising at 25-30% pa the curves are likely to cross before the UK can get a single new nuclear power plant on line (circa 2020).
Chris, I do not and never have had a problem with using renewables wherever they are possible and reasonably economic.
I do not reject any of the alternatives - it is others who, when we are is a desperate situation regarding energy supplies, do not seem to feel that the challenge of switching energy sources in an unprecedentedly short time is challenging enough, and wish to rule out the use of the only low-carbon non-fossil fuel resource which actually works at a very large scale.
Many of the grounds used for this are absurdly thin, an alleged 'concern' for possible shortages in a hundred years time, when we are in great need now, or on safety grounds, when for the peak oil aware it is plain that billions are likely to die, either through climate change or starvation.
This rejections tends to be absolutist - we do not need nuclear power anywhere, as renewables will do the whole job, without reference to what the actual resources of the area are, which in itself does not respect and take account of the properties of renewables, which are inherently local.
So you end up with sweeping blanket statements and 'solutions', which should arouse suspicion in the first place.
To make a concrete comparison, consider the problem for two different areas, Australia and Britain:
To take Australia first: what would be needed to run this sunny, low-population density country with renewables?
Solar is the obvious first thought, and the resource is plainly adequate, however both cost and storage remain significant technical hurdles.
Hot rock geothermal is very much in it's infancy, and will need further work before it can even be realistically assessed.
If a stop to coal burn as wanted as soon as possible, what would be by far the easiest thing to do and for which we have off-the-shelf technology would be to build nuclear plants to take care of base-load, which is where they are most suited, and work in the renewables later.
Not to be too prolix or ignore other resources, but it is perhaps clear that it would be reasonable to expect that it would be possible to run Australia solely on renewables eventually, but that significant technological hurdles remain, and the cost it unknown.
To comment a little further on solar, the next step in our engineering would be to build plants in sunny areas which would supply peak power, as that avoids whole areas of difficulty in storage.
The most suitable places for this would be as near the equator as possible, as much more problematic for solar power is annual variation in incidence, which means that either you have to top up the solar power in winter, or greatly overbuild.
Once we have plants running in those conditions, and have substantial experience with running them, then it is sensible to move on to more challenging conditions, requiring off-peak storage and so on.
If we come to the example of Britain, we can see why the 'all renewables. right now, everywhere' attitude is pretty daft.
The UK is everything Australia is not.
It is densely populated, not sunny and with a high variation between winter and summer sunshine, has limited land area to build the less expensive on-shore wind turbines which look good on the American plains, and so on.
How sensible is a 'solution' which assumes that renewables are just as do-able in the UK as Australia?
This is not thinking about an issue, it is expressing a prejudice.
Any real solution would take proper account of the actual conditions, the realistic stage we have reached in out engineering and the considerable time it takes to fully test solutions before rolling them out on the largest scale, and so on.
Above all, if we are in a desperate situation for energy supplies, it would jolly well get on an build what we know how to do.
Attempting to tackle our energy problems without using nuclear power is like going into a race and chopping off one of your legs because you don't like the style of the shoe.
I believe in using all means available to deal with our problems. Others seek to rule out our biggest non-fossil fuel resource on the basis of umpteen hypothetical 'concerns'
In Britain at least the lights are shortly going to start going out, with immense suffering the consequence, when with renewables the most that can be done is to make a small contribution at immense cost.
The delays in fuller deployment of nuclear power here is going to cost very many lives.
Okay, I also do not and never have had a problem with using nuclear wherever it is possible and reasonably economic. However in my mind this excludes large parts of world including equatorial Africa where PV is cost competitive (expensive elec and lots of sun) today and the UK when nuclear is too late to address the energy shortage.
You say yourself that "in Britain lights are shortly going to start going out, with immense suffering the consequence", do you think nuclear has a roll to play addressing this?
You seem to be suggesting that solar doesn't work at very large scale. With many GW of nameplate being deployed annually on a ~40% growth curve it seems to me that solar does work at very large scale. Similar numbers exist for wind. The critical differential is that renewables deliver from the first year.
Equatorial Africa is good, but although it is nice to be near the equator due to the low annual variance of solar incidence, for many technologies cloud cover can cause problems.
It kills solar thermal, the best bet may be amorphous silicon, which although not as efficient by area as crystalline, looses a lot less power when it is cloudy:
http://www.solarvoltaic.com/images/doc/solar%20abstract.pdf
I don't have the profile for some of the exotic thin-film technologies in cloudy weather, if you come across any info perhaps you would pass it to me at the energy security website.
This leads us to another consideration: in many monsoonal areas you get a lot less sunshine for months of the year.
One also has to take into account what would happen if you had, say a volcanic eruption, and so solar incidence was greatly reduced for a year?
It all boils down to the fact that any sensible system of supply tries not to put all it's eggs into one basket, so you use variety of sources.
Lots of other things to say about the real and important role solar has to play as a contribution, but not to go on too long some work is being done on solar thermal at a very large scale, and the next challenge is building some storage to extend the hours it is available - for instance on molten salt storage there is still a lot of work to be done to prevent it freezing solid, which itt does at a couple of hundred degrees C, and ruining the plumbing.
It is hopeful but not there yet.
For me the first area where solar is likely to be truly competitive would be in medium size installations, at first in sunny, remote locations where it can be combined with wind and biogas and avoid connection charges, stepping down as you can have it at the voltage it is needed, and you can build it on the ground so you avoid fooling around on people's roofs for installation and maintenance:
http://nextbigfuture.com/2008/04/solar-thermal-municipal-power.html
Next Big Future: Solar Thermal Municipal Power
Please note that Brian has made an error in his title - this is thin-film solar PV, not thermal - just noticed it myself!
The use of this power will not be confined to the type of locality I have described, but it is the right place to start and accumulate hands-on experience.
On what will happen in Britain, Government plans assume that LNG supplies will be available, and we have spent billions building terminals to import it.
However, massive shortfalls in the projected expansion of production are now certain:
http://www.tehrantimes.com/index_View.asp?code=175879
tehran times : LNG project delays may cut 100 million tons of supply
On the power front, Britain is now running at near to minimum levels to keep the lights on on a cold day, and has much lower natural gas storage capacity than other European countries.
Out of a total capacity of around 75GW, 30GW is due to be retired within a few years.
Everyone, including the British wind energy association, knows that the Government 'plans' to generate 33GW from wind by 2020 are a fantasy - most of this is to be off shore, and we currently have exactly one vessel capable of putting the turbines into place!
It should be borne in mind that from the 33GW installed, only around 10GW of average hourly output will be obtained, and that the cost is vast - on the most generous reckonings, making conservative estimates for the likely cost of the wind power and taking the most expensive estimate I could find for nuclear power, then the cost to build is somewhere over twice the cost of a similar nuclear build, although it should be noted that if interest rates go through the roof nuclear costs rise heavily - but that probably just means that we won't be able to build either, because although the effects are not so severe for wind, they still do not do costs any good and they start from a much higher base.
Can nuclear be built in time to fill the gap? No.
It should be noted though that much of the time for a nuclear build is in regulatory approval - for instance, the Government want to fanny around putting a 'British' stamp of approval on all designs, when they have already been passed by countries like France, Finland, and Canada - I don't know what they imagine they know that regulators there don't.
It illustrates nicely the total lack of urgency by the authorities here to the looming catastrophe though.
The good news is that actual build times can be as low as four years, but there is bad news about that too, as since everyone is trying to ramp up their nuclear production at the same time serious shortages may delay things.
There is also the possibility of getting the French to build a couple of reactors on their side of the channel and export the electricity to us, which might be faster.
In reality though, we are likely to end up building several coal plants.
Here is a discussion on our situation, including the influences which are likely to force us towards a coal build:
http://fellsassociates.awardspace.com/site/LinkedDocuments/Pragmatic%20E...
Pragmatic%20Energy%20Policy1.pdf
It all boils down to severe shortages soon- see Euan's articles, with the first relief coming in around 2017, and anything like reasonable supplies in about 2020 or so.
Here are my ideas for conservation to help tide us over:
http://energy-futures.blogspot.com/2008/02/conservationour-best-route-to...
Please note that the £45bn estimate for wind referenced there is now at least £66bn, and that I have since changed my mind about residential solar thermal as it is better than nothing even in this climate, and with our energy policies nothing is probaly the alternative.
Lastly, it is now apparent that we will have to struggle through in a quite awful financial environment, so there simply will not be the money to pay for fancy, ideal solutions.
My guess would be that we will end up with a lot of coal power, for many, many years.
In the absence of hydropower, in reality opposition to nuclear energy has always resulted in coal burn.
As Marx would say 'objectively speaking', opposition to nuclear power is support for the coal industry.
I think this is wrong. The largest uranium mine in the world is the open-pit Olympic Dam in Australia.
It's true that leaching is the only uranium mining in the USA but the USA produces only 2% of world production~900 tons per year.
http://en.wikipedia.org/wiki/Uranium_mining#In-situ_leaching
Yep. Solar has great potential.
You haven't worked through these numbers.
Currently, a 1GW reactor takes about 170 tons of uranium per year. 170 tons / 60ppm = ~3M tons of shale per reactor per year. At 4-5 tons/m^3, that's about 0.6M m^3 per reactor-year.
Currently existing open pit mines range in size up to about 0.5 cubic miles, which is about 2,000M m^3. Even assuming 2/3 of that is overburden removal, an open pit mine of today's size range could supply 100 reactors with 10 years of fuel. A mine covering "hundreds of square miles" would supply thousands of reactors for decades.
I'm not saying it's a good idea; I'm just saying the scale of it is not so vast as you suggest.
Oh Mr. Pitt,
The Chattanooga shales contain 4.5 million tons of uranium at 57 ppm according to the IAEA. The strata of these shales is 10-20 m thick(use 15 m). Therefore you would have to mine 79 billion tons of shale. Using your 4.5 tons per m^3, you get
17.5 billion cubic meters divided by 15m thick this deposit would be 1.1 billion square meters or 424 square miles--which is hundreds of square miles as I said.
My calculations are that a 1 GWe reactor requires 196 tons of uranium per year, therefore
4.5 million tons would run 500 such reactors (producing 100% of US current electricity for 46 years).
But this wouldn't satisfy a REAL cornucopian-uranium is must be INFINITE! ;)
Let's assume that that these Black Shales can be mined like tar sands(oil shales?). The biggest tar sand mine is Syncrude Base which mines 520000 tons of oil sands per day and leaves 440000 tons of talings. This mine covers 15 square miles and the seam is 60 meters deep, so a similar chattanoga strip mine would have to cover 60 square miles and would produce 10818 tons per year, which is enough for 54 1GWe nuke plants.
Interestingly Syncrude mine produces about 1 quad of syncrude per year and the uranium of the imagined Chattanooga uranium mine would produce
1.5 quads of electricity per year.
But this assumes that all 57 ppm would be extracted.
Typical uranium mining tailings have 24 ppm of uranium left in them. If you could only achieve this level( net 33ppm) you could only count of a 2.6 million ton resource(500 1GWe reactors for 26 years).
And would it be economical to reduce this level to say .5ppm(56.5ppm)?
Gold costs about $20000 a kg and can be economically extracted down to 0.5 ppm. Yellowcake costs $200 a kg and extracted down to 24 ppm(tailings). How high would the price of uranium have to rise for extraction methods to go to .5 ppm?
I would say that given the fact that the price per kwh of oil today is slightly less twice that of electricity, the chances of a high purity operation is next to zero.
If you can imagine yellowcake going for the price of gold, then I can believe you think this is possible.
If this shale was "mined" by leaching from horizontal well bores, 424 square miles of shale could be processed for its uranium with minimal disturbance on the surface and no change to contour.
The analogy with gold is not very useful, because with gold, it doesn't matter so much how much of the cost is labour, equipment, or energy, while with fissiles (or fertiles) energy balance is a fundamental condition.
Old and new uranium from phosphate mines are starting up. those get counted in the 9 million tons of speculative.
http://uk.reuters.com/article/rbssIndustryMaterialsUtilitiesNews/idUKN19...
Reserves estimates are still increasing and exploration levels are still tiny.
Three years passed and more reserves so any "projected peak" moved out 7-10 years and ignored the uranium from phosphate which has produced thousands of tons of uranium per year. A proven method.
Reprocessing works and is producing 6000 tons of MOX per year.
As constantly noted, there is a the BN-600 breeder reactor working since 1981 and generated by itself more commercial electrical power than all world solar power over the same period.
Thorium Power is commercializing thorium fuel rods that can be placed in existing nuclear reactors.
http://www.thoriumpower.com/files/platts_11_19_07.pdf
Full size thorium/uranium fuel rods going into Russian VVER-1000 reactor by 2010.
http://www.technologyreview.com/Energy/19758/page2/
Thorium/U-235 and zirconium mix. Would allow refueling to occur every 4 years instead of every two years. Thus reducing operating down time.
India is also commercializing thorium as a fuel for nuclear reactors.
India has started construction of a 500MW thorium fast breeder. Thorium blanket bred into uranium.
http://www.world-nuclear.org/info/inf53.html
Started construction of a 500 MW prototype fast breeder reactor at Kalpakkam and this is now under construction by BHAVINI. The unit is expected to be operating in 2010, fuelled with uranium-plutonium oxide (the reactor-grade Pu being from its existing PHWRs). It will have a blanket with thorium and uranium to breed fissile U-233 and plutonium respectively. This will take India's ambitious thorium program to stage 2, and set the scene for eventual full utilisation of the country's abundant thorium to fuel reactors. Four more such fast reactors have been announced for construction by 2020. Initial FBRs will be have mixed oxide fuel but these will be followed by metallic-fuelled ones to enable shorter doubling time.
Breeders will put plutonium out of circulation and can power the world on uranium for the duration of civilization. They function nicely at the back end of today's thermal-reactor cycle.
The technology is ready for commercial demonstration, and GE and others are prepared to do it as part of the Global Nuclear Energy Partnership (GNEP). GE expects the economics to be competitive. See http://www.gnep.gov/afciparticipants/industryinvolvement.html.
For background, see the piece from the Dec. 2005 Scientific American, to be found at
http://www.nationalcenter.org/NuclearFastReactorsSA1205.pdf
George - "Breeders will put plutonium out of circulation and can power the world on uranium for the duration of civilization. They function nicely at the back end of today's thermal-reactor cycle."
So you can trust every country in the world not to make any nuclear weapons from the material from the breeders and you also can have inspectors in every nuclear reactor on the planet to ensure every gram of Plutonium from the breeder goes back into the thermal reactor?
I don't think so. Civilization will not last long enough if any tin pot country with a grievenace has easy access to plutonium especially as we are coming to the end game of oil. As this whole site is about Peak Oil do you really want desperate nations fighting with nuclear weapons for the last of the hydrocarbons?
Actually, it would be a very good start if only we could trust that the USA won't "make any nuclear weapons from the material from the breeders".
All the countries developing breeder reactors already have nuclear weapons (Russia, China, India) or easily could but choose not to (Japan, South Korea).
The point would be to displace fossil fuels with biofuels, nuclear power and renewables.
The order-of-magnitude estimations are informative and show fairly conclusively that for each individual substance (e.g. U or Li), extraction is not feasible.
How about a system/process which extracts several resources in one go? Could we devise a system which processes about the same (still gigantic) amount of water but extract both U and Li, as well Ba, Mo, Ni, Zn and Fe using only a little more energy? How to guesstimate the energy needs of such a combined process? Colocating with desalination plants might also help since they already move fairly large amounts of seawater at high pressure.
A goof poiny. If you could use something like an elution column, you could have all the elemets nicely separated by sections. But it has never been done with seawater; not even proposed. The problem is, I think, that the differences in concentrations are enormous; so your elution column would be immediately clogged with sodium, calcium, magnesium and potassium. Then you would have to regenerate it every time in order to get infinitesimal amounts of the metals you really want. Or so I think, at least....
I agree that we should have 'one stop shops' that combine thermal plant and desalination now and mineral extraction later when economically justified. I note seaweed is a naturally evolved membrane technology for mineral concentration, notably potassium and iodine. Dairy farmers near here sometimes let pregnant cows graze on bull kelp washed ashore. A Japanese seaweed concentrate is known to accumulate up to 3 ppm of arsenic. Perhaps a mineral concentrating seaweed could be rendered for alginate, the residue burned for heat and minerals taken from the ash.
On uranium it looks like there might be a new version of 'petro-politics'. Australia has refused to supply India with U3O8 as they haven't signed the nonproliferation treaty. Despite a 2007 deal between Putin and former PM John Howard the new government won't supply Russia until they agree to resumed IAEA inspections.
Well, it is a well known fact that the lowly Ascidians are capable of concentrating Vanadium at levels of over 100 times that of the surrounding seawater in which they live. I also think I read somewhere that Vanadium can be used to make a pretty decent battery. Maybe we can use old and useless oilrigs as artficial reefs and farm organisms such as tunicates on them to concentrate minerals for us. Now imagine if some of these organisms could be genetically tweaked a bit to concentrate lithium or Uranium, Ok, that's science fiction but maybe not completely beyond the realm of possibility.
Very interesting posting, Ugo.
Maybe it could be more rewarding to extract these minerals not from seawater but from wastewater. I remember a German study about material efficiency, which showed for example, that although copper can be recycled about a quarter of copper is lost - for example as small particles or dissolved in paints. One day some of this "lost" copper might be retrieved more efficiently from our garbage dumps and incineration plant ashes (a special sort of "urban mining") or also from our sewage plants.
There are already activities to re-use the sludge from these plants for agriculture as agricultural fertilizers (which might solve the phosphate problem!), however there are still concerns or problems with nocious particles in the sewage.
This may sound utopic, but I remember that some 20 years ago the German industry complained that the politically demanded recycling wasn't technically and economically feasible - meanwhile it is a standard process - and very profitable.
Yes! Our next mines are not in the oceans but in our landfills.
Thank you for the informative article.
What I didn't find, and maybe I didn't look hard enough (just finished 12 hours at work)was a discussion of the impact of the technology on the ocean. Perhaps that mention of diverting the gibralter current was it? The imagery drawn from those few sentences was powerful and disturbing.
My concern is that we as human's have created disasters thru our creation of "mega" installations. the few examples I can think of now are things like the Aswan dam which has caused the exact opposite of it's intended purpose - a decreased productivity in the nile valley due to decreased amount of eroded soil being transferred to the valley from upstream resulting in a dependence upon heavy fertilizer application. The draining of the Aral sea due to large scale irrigation diversions creating a massive salt flat. these are two that I can think of right at the moment.
I just wonder what unintended effects we might see if this type of technology were deployed to the scale required for profit? Massive planting of uranium concentrating plants also leaves me uneasy as I contemplate further biodegradation resulting from an attempt at oceanic monoculture.
It may be financially profitable to attempt these projects, and since Japan has no (?few?) national uranium sources other than seawater it may be in their own best interests to do so. I just wonder what the comparison of the impact of mining versus seawater on our planetary ecology would show?
We have seen the ecological disaster which has resulted from our megascale reliance upon FF for energy, transportation, agriculture, etc. I wonder what effects will arise if we scale up our use of nuclear/renewable energy sources. They all require an infrastructure - we have to look at the cost in invironmental terms of that too. Likely any form of large energy development will leave a footprint - it would be nice to have some idea of what size shoe we are looking at. Apologies if all this has been covered in earlier blogs.
Regards,
Al
I didn't get into details, but it seemed to me that filling an area 6 times the North sea with adsorbant structures was horrible enough that I didn't have to mention that it is - indeed - horrible. think of the disaster for the sea that has been done by oceanic fishing. Uranium fishing would be at least ten times more invasive and would do damage to marine life at levels that are impossible to estimate. Fortunately, the EROEI is bad enough that it will never be done. But, it must be noted, if the EROEI were by any chance good, I think that it would be done.
I saw further back that the a(d/b)sorption process works best at warm seawater temperatures.
It seems to me that proposing use of the North Sea for the process is an arbitrary choice, perhaps intended for illustrative purposes only. To me what's logical is to propose use of beds of a floating seaweed (what's the Sargasso Sea weeded with?) in the area where the Gulf stream crosses the Equator. http://www.britannica.com/EBchecked/topic/419398/North-Sea. Need 4x th area of the North Sea? 1100 km by 1000 km well distributed in the Atlantic's Gulf Stream near the Equator, and the same amount well distributed say in the Japan Current of the Pacific which is 14 million sq km in area. The Pacific beds would occupy a total of 7.8% of the Pacific surface.
Of course by adjusting the article's assumption slightly to include re-use of spent fuel and breeding of fuel, the REAL fuel reqirement and the area requred to collect it drops by a factor of at least 100/0.7 = 143, so the surface area becomes 7.7 km x 1000 km in each ocean, entirely reasonable.
Ugo, this has been bothering me for a while but I didn't have time time to get into it, BUT:
What makes you think that processing 6 times the water volume of the North Sea every year means a collecting system of 6 times the static volume?
As an example, the Japan Current moves roughly 15 Sverdrup units per year. That is the amount of water passing a certain area, not resident in a given volume. If you could erect a curtain across 7% of the current and process the water going through it, you'd handle 1 Sverdrup/year. That would be a mighty big curtain, but a very small static volume.
You can forget the energy cost of moving the collectors around. They're in a freaking ocean current; if you shape them correctly the water will do the moving for you, just as a correctly-shaped layer of fabric stuck up in the air will move a ship around for you.
This could even be combined with ocean-current energy systems. The same concept for an aerial "laddermill" wind-energy system would work underwater, and the surfaces of the wing sails coated with ion-capture polymers to perform double duty as water flows over them. The uranium collector would be a net energy producer.
I think the real issues are in the lifespan of the collecting polymer and the energy cost of extracting ions from it, but you have little hard data on this. It is impossible to rule out the feasibility of oceanic uranium capture based on the information you've presented here.
Hi EP,
Perhaps this link will help:
http://npc.sarov.ru/english/digest/132004/appendix8.html
Projects | Review - Issue 13
In the experiments the adsorbant declined in effectiveness by 20% after 5 uses, ans they base their figures on 20 times re-use.
The weight reduction they refer to is by using plastic instead of metal cages, I believe - some of my links have expired.
It looks like useful amounts of vanadium and nickel would also be recovered as a by-product!
Hope this gives you more to chew on! - I look forward to reading your analysis.
Vanadium and nickel? How interesting. ISTR that the ocean is so full of manganese that it precipitates as nodules on the ocean floor, so it may be that such a system could have the side effect of making manganese very cheap as well.
You are right, EP. I let myself to be carried away in that comment of mine. Of course, the North Sea is just an example of the volumes needed; it is not meant to be seen as a place where you would actually place adsorbant structures. And your "laddermill" idea is indeed very similar to the concept that I sketch in the conclusions of the paper. I think you don't really need a laddermill. Just place your structure at the bottom of the sea and it will adsorb forever metals and you can just have the packaeges float to the surface. It is physically feasible, so the theoretical feasibility of oceanic uranium capture can't be ruled out. My point was, however, that is not feasible now and it will take a long, long time before we can even think of oceanic laddermills for mining uranium.
Xeroid has made the most sensible comment of all. What is technically possible is very different to what will be achieved. For something to develop on a large scale requires an investor to have confidence in receiving decent return. No return, no investment. The more expensive things become (battery vehicles for example) the less affordable to the masses they will be. If its not affordable it won't be profitable. As energy costs rise spare cash is eroded. Just observe what £100 dolar oil is doing to the world economy (just as predicted by all those pessimists such as JHK and Heinberg!!).
Pitt likes evidence, all he needs to do is open his eyes and observe what a 6 billion population, plus a growth of 60,000,000 a year, is doing to world food and energy resources hence cost.
Sorry all, but we are heading for the buffers, just a question of how far away they are. I hope its 40 years before we hit them, should see me out.
I have never seen a sentence start that way and end with evidence. "Open your eyes and you'll agree with me" is nothing more than "I really, really believe my own opinion".
6 billion was the population in 1999; over the next five years production of cereals rose by 9% and of energy by 15%, outpacing population growth of 6.4%.
So what a population of 6B appears to be "doing to world food and energy resources" is increasing them, or at least increasing the available supply of them. I suppose that's interesting, but I'm not sure why you've pointed it out.
If you don't believe in your own opinion then there's no point in having an opinion at all! In fact the definition of opinion is close to being "one's beliefs" without proof!
As for poulation numbers, I only work in round figures. 6 billion is close enough for what I was saying.
Are you and Engineer-Poet one of the same person? If not you must be lost brothers.
The real issue is if your opinion is worth anything. For instance, if the year's added population has a subsistence existence as 99% of humanity has had since the species evolved, it would have no impact on fossil energy demand.
Never met Pitt the Elder. No relation, but sounds like he's another guy who can look truth in the eye without blinking and change his opinion as required. It's a worthwhile skill, you should try to develop it.
The problem is establishing the truth! You can have two experts head to head, faced with the same set of data and yet they draw opposite conclusions. It happens on this blog, radio and television interviews and in technical journal letters' sections. The truth is nobody knows very much and most are biased to their needs. Quoting data and its source is no guarantee of data reliability. If one uses the power of observation one can learn a great deal. Perhaps you should learn that trait.
If the facts available do not permit a firm conclusion, this is to be expected.
The solution to that is to go scientific:
EP
You must have a very large scientific facility at your disposal, coz you know everything. You even claim your turbo is on boost when decending a hill because you can hear it whistle, yet my method of using a pressure guage was not valid. You also suggested manufacturers refrained from throttling diesel engines to provide engine breaking. Utter tosh, Infact I refrained from replying to such nonsence at the time.
I suggest you change the rules to suit your mood, though my evidence is circumstantial, I have to admit.
There is an excellent analysis of nuclear resources and potential energy in the future by Jan Willem Storm van Leeuwen on her website updated in February this year. http://www.stormsmith.nl/
Figures for collecting uranium from seawater are in the same ballpark as this post. When you factor in the costs and energy required to create and maintain such colossal infrastructure, you have to wonder..just why are we still talking about nuclear power? Because it's a stop gap for business as usual for another few decades?
Alternative energy sources such as solar, wind and geothermal require serious investment now in order to meet future energy demands at any level.
Anyone who'd actually gone to that website and read the first line would know that Jan Willem Storm van Leeuwen is likely not to be referred to as 'her'.
An arbitrary assumption, the selection no doubt designed to make the core proposition of the paper, which is that fuel processing energy costs make nuclear power costs prohibitive, carry even a whiff of resemblance to reality.
In Chapter Two, discussion the fuel cycle which is where the entire thesis is made or broken, they present on page 10 a table showing a broad selection of references showing that Gaseous Diffusion enrichment uses at least 8 times more energy than the Ultra-Centrifuge method.
(Note - very difficult to quote him because he's locked the document so copy-paste doesn't work).
Then they immediately follow on page 9 with the above completely unsupported statement, based solely on their hypothesis that maintenance costs of U C eliminate its advantages. Ridiculous, and certanly not anything close to scientific.
If Fuel enrichment were a serious economic / energy issue for nuclear reactors, then the CANDU reactor, which uses un-enriched natural uranium fuel, would have a huge economic advantage over LWR's, which is not in any way apparent in the industry.
Similar throughout.
It is a little difficult to take what you say seriously since you refer to Chapter 2 while the update is in lettered parts. In any case, the ratio of specific energy between centrifuge and diffusion used in Part E is 3.5 not two and references are given to justify this. It is worth remembering that centrifuges are subject to catastrophic failure and may well need more maintainence.
You may have forgotten that CANDU reactors use heavy water?
Chris
And?
Heavy water costs about $600/kg and CANDU reactors require about 500Mg of it, or about $300M worth, which is somewhere around 5% of the cost of a new reactor.
If you're concerned about the energetics of it, consider that if the entire cost was due to electricty @ 10c/kWh - 3B kWh or 3TWh - that would represent around 4 months of operation of a 1GW reactor. When compared to an expected lifespan of 50 years or so, it's not really a concern.
You are correct that it is not that big of an energy input. It is an input that light water reactors don't have though which is why I mentioned it. You can enrich uranium or you can enrich hydrogen. But, you do use up the uranium.
The comment about the CANDU is a distraction because it is clear that Storm-Smith is not about diffusion or centrifuge enrichment in the end but ore grade. Ugo's work here is interesting because there is an ore grade issue. That the Storm-Smith work is more complete wrt. light water reactors than CANDU reactors is not really a reason to complain. It is important that the detailed consideration demonstrates that comparable industry estimates are unreliable, but what is really looming is the ore grade problem and the waste problem.
Chris
Ugo,
Looking again at your figures it is apparent that you have simply used the average efficiency of present reactors for your EROI calculations.
Since we would certainly at least go to reprocessing before extracting uranium from seawater, then the EROI calculations would not appear to be realistic.
Most places still use a once through process, and in addition the present fleet contains many old, inefficient reactors, so if an impartial assessment is to be arrived at then at minimum the latest designs would be considered, and reprocessing assumed.
So perhaps in it's present form this is rather like looking at a Newcomen engine and saying that the efficiency is so low that railtravel would be forever impossible.
If you wish to argue the entire impracticality of a technology, then it seems fair to make the most favourable assumptions possible for it.
This does not appear to be the case here.
Please correct me if I am in error in any way.
I have now had an opportunity to check the figures, and it appears that present levels of reprocessing although raising the efficiency would not materially affect the figures, and the efficiency of the overall fleet would be in the right ball-park, although the difference due to the more efficient burn in the latest reactors would also raise the EROI if general throughout the fleet by the time uranium is extracted from the sea.
Something from my research I found interesting: the concentration of U-235 in at least some "spent" PWR fuel is greater than in natural uranium. If you extracted the uranium from the spent fuel as UF6, you could feed it directly into the enrichment cycle again. If the finished fuel is about 4% U-235, 5 loads of spent fuel would have enough U-235 to make a new load (somewhat less after losses in the tailings).
The French get around 30% extra by reprocessing apparently:
http://www.world-nuclear.org/info/inf40.html
That looks wrong.
Depleted uranium has .2-.4% U-235 while natural uranium has .7% U-235.
http://en.wikipedia.org/wiki/Depleted_uranium
I think that has been done on some small scale -- the uranium is known as 'repU' -- but it contains 236-U, so the depleted stream from such re-enrichment is dirty with man-made radioactivity, small in amount but not zero, and can't be put in the same field of drums with depleted uranium from all-new natural uranium feed. Or maybe the same field, but not the same drums, and one has to keep track.
The proposed DUPIC process involves pulverizing spent UO2 and heating it in oxygen, a way of partially de-ashing it, enough that a CANDU reactor can then burn it some more. With the spot price of newly mined U3O8 down this week from to US$0.270 per mmBTU from last week's US$0.288, none of this much interests plant operators.
--- G.R.L. Cowan, author of How fire can be tamed
You mean that I used the current technology data for carrying out the calculations. Of course I did that and it is clearly stated in the text of my post. I never wished to argue for "the entire impracticality of a technology" and this, too, is clearly written in my text. My point is that uranium extraction from seawater is not practical now, and it will not be practical until some much more efficient nuclear technologies will be available. But, if such technologies become available, why bother with seawater extraction? It will be easier to use conventional mining.
If I were making this kind of calculations for coal at the time of the Newcomen engine, I couldn't have used the data for a steam turbine.
Please note my later post, in which after checking the figures it was apparent that there was in any case no major discrepancy, and I acknowledged this.
Cheers.
300 kg per (kgU-per-year)? That would make sense if the membrane made exactly one round trip per year. Is that it, or are the words "per year" just wrong?
--- G.R.L. Cowan, H2 energy fan 'til ~1996
It is not wrong, but maybe it is not clear (sorry). It means that 300 kg of membrane can produce 1 kg of uranium in a year, but in order to do that, the membranes need to be processed at least once per month.
That implies the membrane is removed from the sea when it is, by mass, less than 0.00028 uranium. As such it fetches, per extraction, the heat of barely three times its mass in oil.
Since you have taken the trouble to seek out the web-inaccessible Nuclear Technology*, 144, (Nov. 2003) pp 274-278, and it apparently disagrees with the 0.002-to-0.006 mass fraction takeups considered in Tamada M. et al., Cost Estimation of Uranium Recovery from Seawater with System of Braid type Adsorbent, Nippon Genshiryoku Gakkai Wabun Ronbunshi. Vol.5, No.4 2006, p.358-363(in Japanese), as translated and made web-accessible at http://jolisfukyu.tokai-sc.jaea.go.jp/fukyu/mirai-en/2006/4_5.html, it would be nice if you would quote exactly the passage from which you learned of the less-than-0.00028 takeup.
--- G.R.L. Cowan, author of How fire can be tamed
* Not "Nuclear Energy" as it is given in the reference list.
I said two days ago,
One reason it would be nice, if as I suspect Seko et al did not say what you needed them to, and therefore you changed it, is that it would require you either to correct a convenient error, or utter a direct lie.
Of course, this is just the net. You don't have to be serious if you don't want to.
--- G.R.L. Cowan, author of How fire can be tamed
There are assumptions here that I take issue with.
First off, we will not pump this water using petroleum or nuclear energy, We will use some combination of solar, wind, tidal, wave, and ocean currents energies.
We will not be pumping the water for the sole purpose of recovering minerals, in fact we will be desalinating massive amounts of potable water to send thru pipelines over a large portion of the water hungry areas of the United States. The minerals recovery will be a by product of the potable water production. The value of the minerals recovered, whatever that may be, will be used to offset the cost of desalinating billions of acre feet of water for human and agriculture uses.
The technology will be constantly improving and there will be recovery of more and more minerals as time goes on.
Larry Hagedon
Ugo, here are a few thoughts to consider.
At what price does uranium become expensive?
If all U.S. electricity was made with coal, an average persons year’s supply of coal (14,200 lb) cost $218 in 2005 and is much higher now and climbing. A year’s supply of natural gas (115,000 cubic feet) cost $850 in 2005.
To make all U.S. electricity with current reactor designs, we only need 0.72 pounds (330gm) of uranium / year / person.
For uranium to match the price of coal or natural gas, using current reactor technology, the uranium price would be $303 or $1,180 dollars per pound respectively.
Using breeder reactors we need 0.35 pounds (159 gm) / 80 year lifetime.
For uranium to match the price of coal or natural gas using breeder reactors, the uranium price would be $51,500 or $194,000 dollars per pound respectively.
So sea water uranium is cheaper than coal and much cheaper than gas with today’s reactor technology. Of course the land based reserves would also be vastly larger at these prices.
To produce all our electricity from fission at the U.S. rate (1,550 watts per person), we need only 99 kg (218 pounds) of membrane per person using today’s reactors, and 596 grams (1.3 pounds) of membrane with breeders. These are modest amounts compared to digging up 14,200 pounds (6,440 kg) of coal each year, transporting it hundreds to thousands of miles and disposing of the waste.
These numbers come from this paper
http://coal2nuclear.com/energy_facts.htm
I see the adsorbent deployed from processing ships anchored in a row across the Gulf Stream and other suitable currents. It could be deployed in long loops that would be continuously rotated through the ships processing systems. Ships power can be generated with water turbines mounted on the ships, like wind turbines but more compact due to the density of water. Only the product would be moved off the ships, a very small mass, easily transported.
We do not need breeders for a long time but we should move forward with breeder R&D to reduce mining and waste volumes.
Why are there no sea water uranium extraction plants?
Historically the price has been under $60 / pound with a few big spikes.
http://www.uxc.com/review/uxc_g_hist-price.html
http://www.uxc.com/review/uxc_g_2yr-price.html
U3O8 is 85% uranium by weight.
Would you bet your life savings on uranium staying above $200 / lb? I don’t think so, and neither do professional investors, however if sea water technology keeps improving the cost may drop enough to make it happen sooner than most people think.
Sea water uranium is very important because it puts a cap of $200/pound on the maximum sustainable cost of uranium for thousands of years.
Sea water uranium does not have to supply all of our uranium in order to cap the uranium price at $200/pound. It only has to replace the percentage of land based uranium sources that cost more than $200/pound, and that percentage is zero for the foreseeable future.
The uranium supply is effectively unlimited.
Thanks for the link GRL Cowan, I used Ugo’s number without checking.
http://jolisfukyu.tokai-sc.jaea.go.jp/fukyu/mirai-en/2006/4_5.html
This works out to six 60 day cycles per year. At 4g per cycle that is 24g / kg adsorbent / year. At this rate we only need 41kg of adsorbent to produce 1kg of uranium per year.
25,000 yen/kg equals $238/kg, equals $108/pound.
To make all U.S. electricity with our primitive steroidal submarine reactors, we need 0.72 pounds (330gm) of uranium / year / person.
For uranium to match the price of coal or natural gas, the uranium price would be $303 or $1,180 dollars per pound respectively. Seawater uranium is already much cheaper than our cheapest fossil fuel.
To produce all our electricity from fission at the U.S. rate (1,550 watts per person), using seawater uranium, our 0.72 pounds per person will cost $77.80 per year. We will need only 13.6 kg (30 pounds) of membrane per person using today’s primitive reactors.
Using breeder reactors we need 0.35 pounds (159 gm) / 80 year lifetime.
For uranium to match the price of coal or natural gas using breeder reactors, the uranium price would be $51,500 or $194,000 dollars per pound respectively.
To produce all our electricity from fission at the U.S. rate using seawater uranium in breeder reactors our 0.35 pounds per lifetime will cost $37.80 / lifetime, 47 cents per year. We will need only 83g (0.18 pounds) of membrane per person
These are very modest amounts compared to digging up 14,200 pounds (6,440 kg) of coal, transporting it hundreds to thousands of miles, burning it, disposing of thousand of pounds of toxic solid waste and trying to pump 36,000 pounds of CO2 into the ground for each person each year.
Existing reactors produce 15 lb. of spent fuel per lifetime, 0.19 lb/yr, 85g/yr, and breeders will produce 5.4 ounces of fission products per lifetime, of which only one ounce (28g) will still be radioactive at end of life.
The uranium supply is effectively unlimited.
What are your thoughts on these numbers Ugo?
Bill, you're being inconsistent again. This whole fuel cost argument is a half truth, what matters is the total cost per kWh, including the installation (nuclear, wind, solar etc) and all other fixed and running costs plus backend costs. By your reasoning, solar and wind have no fuel costs so are infinitely cheaper than coal (!).
New nuclear in the US has levelized costs of 10-20 cents/kWh (there's a lot of uncertainty at this point). Not cheap at all, but affordable.
Wind, even at today's high commodity and labour prices, and the tight market, is less than 10 cents/kWh in a good location. That's levelized cost, so including transmission plus grid integration. Cheaper than pretty much all new nuclear, but not by a large margin. Integration costs are going up with increased penetration levels. So at some point we might see saturation of wind, depending on what kind of wind and storage and grid developments occur.
However, this is up to the market to decide, not you Bill.
Cyril, the topic is uranium, I stayed on topic, and you did not. I have written many pages on all the issues you raise. Submit an article on the issue you think is most important and we will debate.
What are your thoughts on this analysis Ugo? Why are you not responding?
On or off topic is not the most important issue Bill.
Full disclosure is important when you want to make a point. You are the champion of partial disclosure Bill. Congratulations.
Cyril, the author of this post wrote a report with the intention of proving that the uranium supply was a show stopper for nuclear power. I presented an analysis that shows that we have an abundant supply of uranium in seawater that is much cheaper per kWh than our cheapest fossil fuel.
Seawater uranium is great news for anybody who cares about reducing human suffering. It is amazing to me that some people are so embittered towards nuclear power that they are disappointed by this fact.
You could have acknowledged this fact with a compliment, “Thanks Bill for showing why the uranium supply is not a show stopper.” Instead you acknowledged this fact with an insult, “Bill, you're being inconsistent again. This whole fuel cost argument is a half truth...”
The U.S. completed about 5 reactors per year from 1970 – 1990, a time when fossil fuel was abundant and cheap, and most people never heard of global warming. France ramped up to 80% in a similar timeframe. There is no reason we cannot do the same thing now that fossil fuel is getting expensive and global warming is an issue.
There are two reasons the U.S. has not massively built nuclear power plants.
1 The failure of our education system allowed Ralph Nader to scare Americans away from nuclear power, and so 20,000+ Americans die form coal emissions each year, a massive unfunded coal subsidy
2 We are still living in the fossil fuel age. Many people still do not realize how serious the problem is or how limited our options are.
Cyril, you favor two debating techniques.
1 The shotgun. Fire off a blast of accusations that would take a small book to address fully. Your 9/27 post is an example of that. I refuse to engage. Pick one issue, write it up and stick with that issue.
2 The insult. Your last two comments contain examples. When I presented a rational analysis of Price Anderson you responded with an insult, not a rational logical argument.
http://climateprogress.org/2008/08/07/how-much-of-a-subsidy-is-the-price...
I do not enjoy debating you, I believe that is your strategy for suppressing points of view you disagree with. I do it for the silent majority of readers looking for the facts.
The topic is energy balance, not economics. You are not stupid so I've concluded some time ago that you're just another propagandist. Exactly what the nuclear industries do not need.
Your strategy is to suppress energy balance issues, and in stead focus on superficial issues like economics, and even those are cherry picked and obsolete (modern levelized cost estimates for new nuclear are >10 cents/kWh, which you omit for reasons of propaganda). You've got to dig deeper Bill. It's about EROEI, and it's about recent levelized cost estimates. Whenever you talk about energy balance, you resort to hand-waving; you never quantify your energy balance arguments. Indeed I have yet to read one objective fully disclosed holistic article from you.
Rest assure that I do not enjoy debating with propagandists like you Bill. It is a waste of time to try to educate you because propagandists are too prejudiced to learn new things. So all I have left is insult them a bit. It's fun because it's justified. And propagandists have to be marked for all those 'silent readers'. It's the least I can do, and perhaps all.
Cyril, how do you fit 20,000+ deaths from air pollution and 500,000 brain damaged children from mercury into your EROEI equation, or is that just another superficial issue?
Your .72 pound number per person per year looks right.
Your breeder number is completely wrong. More like 1.06 pounds(480 gm) / 80 years.
The price of an amount of bituminous coal(at $70/ton) equal to a pound of natural uranium( at $70/ pound) in a LWR reactor would be $644.
A 1 Gwe coal plant would cost about $1 billion dollars and uses 4 million tons of coal per year costing $350 million dollars per year in fuel. Over 30 year life that totals $7 billion dollars.
So your contention that LWR nuclear is cheaper than coal is definitely a major exaggeration. They cost about the same, but nuclear has much larger liabilities.
A nuclear power plant costs about $2.5 billion dollars plus $1.5 billion dollars to decommission and uses 200 tons per year of ENRICHED uranium costing $59 million dollars at $70 per pound per year PLUS another $56 million dollars per year for future waste management. Over the 30 year life of the plant that would cost $7.0 billion dollars.
http://www.nucleartourist.com/basics/costs.htm
The price of an amount of natural gas(at $10 per 1000 scf) equal to a pound of natural uranium in a LWR would be $3690.
In a breeder reactor it would be $24,840.
In a breeder reactor it would be $199,260.
Why you include costs for breeders make it look like you want to mislead people.
Cyril seems to be right about you, Bill.
The Integral Fast Reactor can split over 99% of the uranium mined to fuel it. My number comes from cell G83 of this spread sheet. Let me know if you find an error in my calculation or yours.
http://coal2nuclear.com/ENERGY%20CALCS%20REV%207.xls
Show us your calculation.
Take a look at the first table of this paper.
http://coal2nuclear.com/energy_facts.htm
Using your $70/ton a year’s supply of coal costs $497 vs a years supply of uranium using your $70/lb costs $50.61. Seawater uranium looks even better using your coal price.
$70 X 4E6 ton/yr X 30yr + $1E9 = $9.4 billion not $7 billion
Next generation nuclear plants will last 60 years. Coal prices have roughly doubled in 4 years. Seawater uranium caps the maximum sustainable uranium price at $108, corrected for inflation, for several hundred years. What will the price of coal be in 20, 40, 60 years?
Coal plants provide half our electricity, equivalent to roughly 200 1GWe plants. They kill 20,000+ Americans per year, 100 deaths per year per plant, and injure hundreds of thousands more.
http://www.earth-policy.org/Updates/Update42.htm
http://www.ens-newswire.com/ens/feb2006/2006-02-15-02.asp
http://www.npr.org/templates/story/story.php?storyId=873954
Children are being born with mercury damaged brains.
“Using national blood mercury prevalence data from the Centers for Disease Control and Prevention, we found that between 316,588 and 637,233 children each year have cord blood mercury levels > 5.8 μg/L, a level associated with loss of IQ. The resulting loss of intelligence causes diminished economic productivity that persists over the entire lifetime of these children. This lost productivity is the major cost of methyl mercury toxicity, and it amounts to $8.7 billion annually (range, $2.2–43.8 billion;”
http://www.pubmedcentral.nih.gov/articlerender.fcgi?artid=1257552
A custom hand built next generation nuclear plant costs more than a present generation coal plant. A fully sequestered next generation coal plant will probably cost much more to build and much more to run than a mass produced floating nuclear plant, but nobody knows how to build a sequestered coal plant.
http://www.atomicinsights.com/aug96/Offshore.html
Majorian, please redo your cost estimate and present the result, integrating over your best estimate of coal prices for the next 60 years and include all the other issues raised above. Include your best estimate of what carbon taxes will be on coal and carbon credits for nuclear, and see if this statement is still true.
Your decommissioning cost is way too high and should be spread out over 60 years, not 30. The money is already being set aside.
http://world-nuclear.org/info/inf19.html
I do not understand what you are getting at here, perhaps you could expand.
It is not unusual for people to speculate on technological developments in wind solar geothermal etc., why not fission? As mentioned in previous posts the Russians have had a breeder reactor running several years.
After recalculating the cost do you still think so?
I try to follow the facts wherever they lead. There is an easy way to change my mind.
Show me the error in my facts and logic.
Show me better facts and logic.
Most people are confused about the terms breeder reactor and nuclear fuel reprocessing. This is the reason why there is so much disagreement in numbers.
All reprocessing technologies are not equal :
1) PUREX : 1960s technology which uses just a sliver of the nuclear fuel, produces a lot of bomb-ready plutonium and doesn't reduce the quantity of the radio-active waste by much
2) Pyroprocessing : As developed for the IFR project, burns up all the U-238, produces only fission products (and no transuranics) as waste, and which decay to natural levels within 300 years. This technology produces 100 times more energy from the same quantity of Uranium pellets as LWRs
3) Molten Salt Breeder Reactors : The reprocessing is similar to pyroprocessing, it is inbuilt within the reactor design over a chemical flouride salt. The advantages are similar to pyroprocessing, and if the nuclear fuel is Thorium, no Plutonium is produced to even scare the anti-nukes.
Clearly the technologies (2) and (3) are the way to go. They should be used by the term "breeder reactors" to be distiinguished from the usual "reprocessing" of (1).
Further, amongst breeder reactors there are different varieties with different burn-up ratio. A burn-up ratio close to 1 is preferable (It means that the reactor consumes all the U-238 or Thorium and leaves no transuranics as waste). Examples of such breeder reactors are the Integral Fast Reactor and the Liquid Flouride Thorium Reactor.
The recent book of Tom Blees : Prescription for the Planet explains the breeder reactor technology in much detail.
I think one basic assumption of this calculation is totally in error.
Sure, seawater contains ions, but this ion content is not "from the beginning" but by constant addition (and extraction).
Metals enter the seas in two ways: Erosion from land and by hydrothermal vents (http://en.wikipedia.org/wiki/Hydrothermal_vent) from the upper crust (they leave it by being dried on land -> salt deposits)
In this state the metal solutions are highly concentrated (compared to seawater).
Actually the most concentrated form of dissovled metals occurs in hydrothermal vents - some mineral deposits on land are former hydrothermal vents (http://www.whoi.edu/oceanus/viewArticle.do?id=2400)
So the need to have large membranes to extract homeopathic quantities of ions from seawater can be replaced by the need to go down about 5 km to the bottom of the ocean to find some black smokers and pipe up the mineral-rich slurry
Excellent idea. Hydrothermal vents are also very HOT so could make for effective membrame capture yield.
The great depth sounds like rather a big negative, though.