Will Nuclear Fusion Fill the Gap Left by Peak Oil?
Posted by Chris Vernon on July 24, 2007 - 8:51am in The Oil Drum: Europe
On the 12th. of December 2006 the UK Magnetics Society held its annual commemorative event with an afternoon seminar followed by the Ewing Lecture. This year the focus was on magnetic fusion energy.
Nuclear fusion has evoked opinions in the various energy blogs ranging from “sixty years of failure and a certain dead end”, to “the reason why we do not need to worry about peak oil”. This event was a good opportunity to gain a clearer view of what part, if any, fusion energy could play in filling the gap as oil and then gas production peak and decline.
After many years of half-hearted support there is now a surge of backing for fusion energy. Many will have heard about the agreement to built the International Tokamak Experimental Reactor ITER. Less well publicised have been the European Fast Track program and the bilateral agreement between the EU and Japan called ‘the Broader Approach’ which, amongst other things, will lead to DEMO, the first full electrical power generating reactor. From the UK side this new found enthusiasm has been in large part due to Sir David King, the Chief Scientific Advisor to the government, who may not fully accept the imminence of peak oil, but does see an energy crisis looming and has become convinced that the possibility of fusion energy is promising enough to warrant substantial investment.
The four speakers in the seminar were senior members of the staff of the Culham Division of the United Kingdom Atomic Energy Authority (UKAEA). The lecture was given by Dr. Frank Briscoe, operations director at Culham. Culham has been the centre of fusion research in the UK since 1960 when the first large scale UK fusion experiment, ZETA was transferred there from Harwell. ZETA was still there, but no longer working, when I first visited Culham from Harwell as a student in 1964. I have retained a personal interest in fusion since then.
Of particular interest to this forum were the Ewing lecture itself, entitled ‘Magnetic Fusion Energy: Progress and the Remaining Challenges’ and the seminar presentation by Dr. Derek Stork entitled ‘Scientific and Engineering Challenges of a DEMO Fusion Reactor’. Since the various contributions overlapped somewhat and some of the material was of specialised interest to those involved in magnetics, I have combined those parts of the contributions that I hope are of interest to this forum.
Dr. Briscoe started his lecture with a brief summary of how nuclear fusion works.
Fusion Basics
Atomic energy, both fission and fusion, exploits the fact that atoms weigh less than the sum of their parts. This is because of the energy that binds them together and is released in forming the atom from its constituent protons, neutrons and electrons and would have to be expended to rip the atom apart back to these constituent parts. The energy released in binding relates directly to the drop in mass of the atom by Einstein’s equation E = mc². This loss in mass is called the mass defect and varies between the elements. Energy can be released by either splitting very heavy atoms in two (fission) or joining light atoms together (fusion).
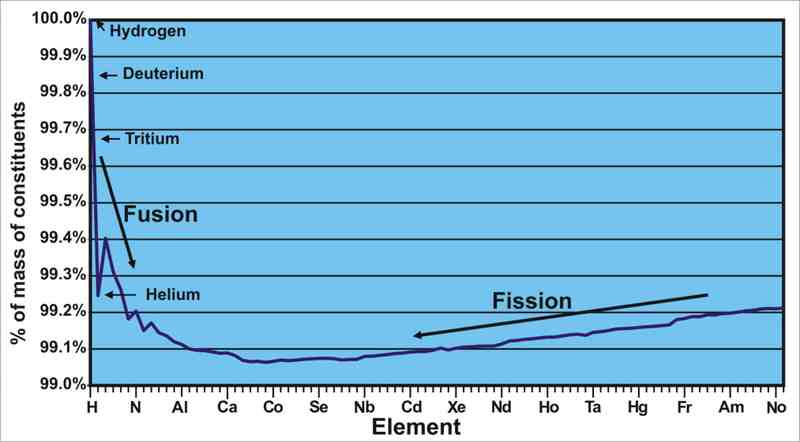
Mass defect: The mass of an atom of each element expressed as a percentage
of the mass of the protons, neutrons and electrons that constitute the atom.
The three isotopes of hydrogen plus the most common or stable isotope of
the other elements are shown. Click to Enlarge
The problem with trying to fuse atoms together is that, although there is a very strong force of attraction between the protons and neutrons in the nucleus when they are very close due to the strong nuclear force, this force drops away very rapidly with distance so that at slightly greater distances the electrostatic repulsion between the positively charged protons becomes dominant. To fuse the nuclei of two atoms, they have to be forced together hard enough to overcome this repulsion until they are close enough together for the force to become attractive.
Many different fusion reactions and many ways of bringing the atoms together have been considered. Far and away the front runner, in terms of progress to achieve a large scale commercial electrical power plant, is the reaction between the hydrogen isotopes deuterium and tritium when heated to a temperature such that thermal collisions between the nuclei carry enough energy to overcome the electrostatic repulsion with magnetic forces being used to confine the reactants. The temperature required to cause the reaction is in the order of a hundred millions degrees. At such a temperature the reactants are completely dissociated into a cloud of nuclei and electrons called a plasma. The plasma is far too hot to confine with material walls but because the electrons and nuclei are charged and moving they can be deflected by magnetic forces and with a suitably shaped magnetic field, confined.
The fusion reaction is deuterium plus tritium gives helium plus a neutron:
2H + 3H —› 4He + n
20% of the energy released by the reaction is carried off by the helium ion as kinetic energy (3.5Mev per ion). Since the helium is ionised it is charged and is confined by the magnetic field. In colliding with the rest of the plasma it gives up its kinetic energy, heating the plasma. If the condition called ignition can be reached this will be the only source of heat needed to maintain the plasma temperature once ignited.
The other 80% of the reaction energy will be carried off by the kinetic energy of the neutrons (14.1MeV per neutron). Since the neutron is not charged it escapes the plasma. In a reactor designed to generate power, a ‘blanket’ surrounds the plasma and the collisions the neutrons make with the material of the blanket transfers the kinetic energy of the neutron to the blanket, heating it up. This heat, plus a little gained from absorbing the hard ultraviolet/soft gamma radiation emitted by the plasma, is transferred out of the chamber by a gas or liquid and used to heat steam, to drive a turbine, which turns an alternator to generate electricity.
Deuterium exists in enormous quantities in sea water where it forms 0.03% by weight of the hydrogen but tritium exists naturally in only tiny amounts generated by cosmic rays, and decays away with a half life of 12.3 years, so that there is only about 3.6kg of naturally generated tritium at any one time distributed all around the planet. All other tritium has to be made artificially. In a commercial reactor the blanket will also perform the function of creating tritium. It will contain lithium in some form and this will react with the neutrons bombarding it to form tritium using the reaction :-
n + 6Li —› 3H + 4He
This tritium will be collected to fuel the continuing fusion reaction. Lithium 6 is a stable isotope and forms 7.5% of natural lithium. Some blanket designs require enrichment of this isotope. The tritium breeding reaction is exothermic and increases the heat production by some 20%.
At first sight these reactions appears to combine to give an overall reaction of:-
2H + 6Li —› 2 4He
However this neat cancellation requires that every neutron from the fusion reaction reacts with a lithium atom to form a tritium atom and that every tritium atom so generated is collected and fed back into the reactor and takes part in a neutron generating fusion reaction. In practice there are many loss mechanisms around the loop that mean that this path alone will not provide sufficient tritium to maintain operation. To augment the tritium production a neutron multiplier is added to the lithium. The main candidates for a neutron multiplier are beryllium and lead. Experiments have shown that tritium self-sufficiency is possible but difficult. To generate much in the way of a surplus is very difficult. Dr. Stork indicated that a tritium breeding ratio of about 1.1 was all that was expected of the designs being considered. This implies that for every 10kg of tritium fed in as fuel for the fusion reaction, 11kg of tritium will be recovered from the blanket. The magnitude of the excess tritium available is probably the limiting factor on how fast fusion energy can spread once a prototype commercial fusion reactor has been demonstrated, as discussed below.
Magnetic Confinement
As part of his seminar presentation, Dr Tom Todd gave a fascinating review of the very varied magnetic confinement systems that have been, and are being, experimented with but again by far and away the front runner in the race to practical power systems are the toriodal systems first developed by the Russians called ‘tokamaks&rsquo, from a Russian acronym. The biggest and most successful tokamak so far is the European run JET system hosted in the UK at Culham. This produced a peak of 16MW of fusion power in 1997 but many other tokamaks have been built across the world.
Critics of fusion power have categorised these experiments as so many failures because none of them have produced more output fusion power than was put in. In truth none of them were built with the intention of achieving energy break-even. All were intended to understand and develop ways of controlling the seething monster that a dense plasma at a temperature of many millions of degrees is. For the most part these experiments have, after a fair bit of modification and adjustment, reached the sort of performance hoped for. Our ability to contain the plasma at high temperature, high density and for long enough to allow a sufficient degree of reaction to take place has increased four orders of magnitude over 40 years.

This shows the progress over the years at confining a hot plasma. The fusion product
is the plasma density in particles/m³ times the time in seconds that the plasma
can be held in these conditions, times the ion temperature in degrees Kelvin.
The requirement for this to be at least 3 x 1028 for ignition to occur in a
deuterium/tritium plasma is one of the Lawson criteria formulated by JD Lawson 1955.
The best results of JET and JT-60U are close to energy break-even, Q = 1. Click to Enlarge
There has also been great progress in predicting the performance of a plasma device as computer power has increased, so there are now fewer surprises in new experiments.
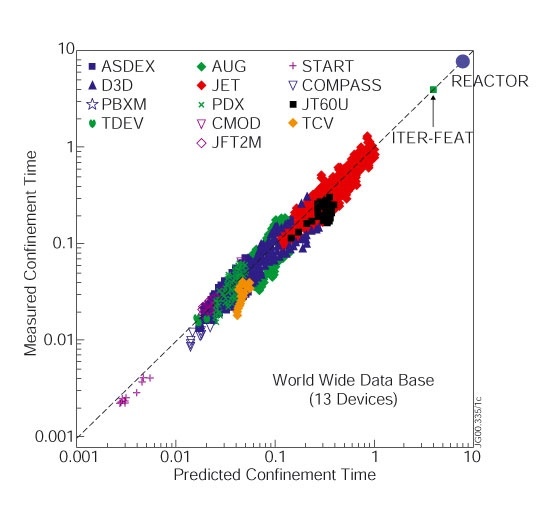
Predicted and measured confinement time for 13 different fusion devices under a great
variety of conditions plus indications of where ITER and a commercial power reactor are
expected to operate by scaling the results of existing machines. Click to enlarge
Although there is still more work to do on plasma control, we have now reached the stage where we can be reasonably confident that just scaling up the size of the reactor will produce substantially more power from fusion than is put in to create the reaction. Such a reactor has been designed in detail and the major parts have already been prototyped. On 21 November 2006, after years of delay, an agreement was signed to build ITER at Cadarache in France financed by China, the EU, India, Japan, Russia, South Korea, and the USA. Together these partners represent over half the population of the planet.
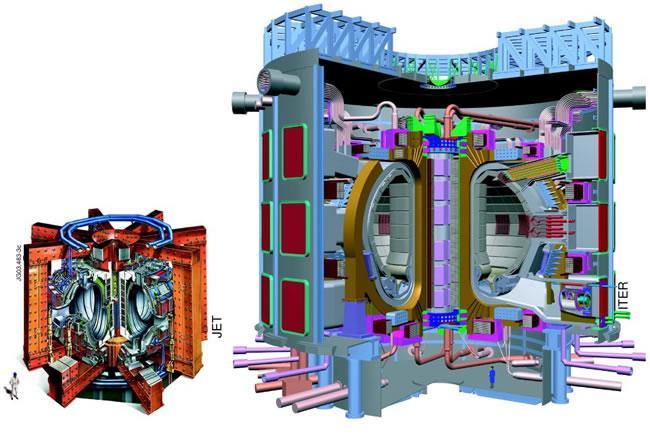
Jet and ITER: The two reactors are shown in cut away diagrams. Human figures give the scale. Click to enlarge.
Power will be feed into the ITER plasma in three main ways: by transformer action causing up to 15 million amps to flow in the plasma; by neutral high energy beams of deuterium and tritium fired into the plasma; and by radio frequency energy fed in from antenna patches in the walls to excite resonances in the plasma, Transformer action is very efficient but necessarily pulsed. The other two forms of heating are less efficient but can be continuous. ITER is expected to generate 500MW of fusion energy output, with less than a tenth of that input power (Q>10) and hold that power for 400 seconds. Also it should generate 500MW output for an hour at an input of one fifth the input energy (Q>5). Although it is not stated as an aim, there is the hope that it might achieve what is called ignition where enough of the fusion energy remains in the plasma to keep the reaction going without the need of external input energy (Q = infinity). This will require higher plasma densities than needed with external energy input.
Although there seems to be reasonable confidence that ITER will come at least close to the target in plasma performance this is just the start of the challenge that needs to be met to build a commercial electrical power generating station.
If all goes well ITER will produce the first plasma before the end of 2016, but, in order to speed the development of commercial fusion power, a ‘Fast Track’ strategy is being adopted and in addition to the ITER agreement there has been a bilateral agreement between the EU and Japan called ‘the Broader Approach’. Studies of the DEMO reactor to follow ITER are part of this agreement.
Beyond ITER
A major hurdle to be jumped is the design the breeding blanket that lines the inside of the reaction chamber and the selection of suitable materials for it. This blanket is required for three purposes; to convert the energy given off by the fusion reaction to heat, to breed more tritium to fuel the reaction and to protect the superconducting coils and chamber wall from neutron irradiation There are a variety of different blanket designs that have been proposed and all of them have some problematical features to them. ITER will not have (certainly not in the early years) a full tritium breeding blanket. Most of the reaction chamber will be lined with a simple cooled neutron and heat absorbing blanket to stop the reactor overheating. There will however be space to fit in and remove small areas of tritium breeding blanket and it is proposed to try, in turn, a variety of different designs.
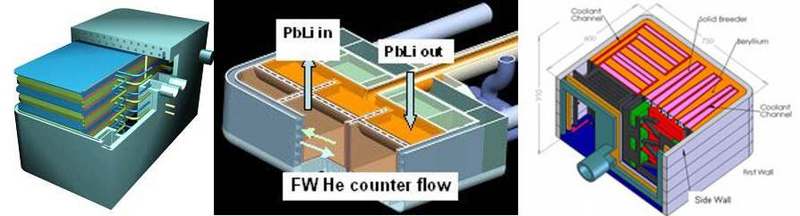
Montage of some of the proposed Test Blanket Modules. Click to enlarge.
DEMO will have a full breeding blanket to achieve tritium self-sufficiency. The materials used to make the breeding blanket and particularly the first wall facing the plasma need to survive an extremely severe combination of conditions and retain adequate strength and other mechanical properties. The heat flux on the first wall of the blanket will 0.1 to 0.3 MW/m² in ITER and rise to 0.5 MW/m² in DEMO. This DEMO figure is about twice that of a PWR type fission reactor and almost the same as a fast breeder fission reactor, The flux of energetic neutrons means that over about 5 years every atom in the first wall will have been knocked out of place an average of about 3 times for ITER and 50-80 times in DEMO and perhaps twice this in a full scale commercial reactor. Each displacement will shift the atom several tens of crystal lattice spaces from its original site. Atomic transmutations caused by the neutron flux will leave hydrogen and helium embedded in the wall. For DEMO this will result in 500-800 parts per million by atom count (appm) of helium and 2300 to 3600 appm of hydrogen. For ITER it will be acceptable for the blanket to be well cooled to keep it at a fairly low temperature but in a reactor trying to generate electricity by a conventional steam cycle, it is important for high thermal efficiency that steam and hence the blanket coolant are run at as high a temperature as possible. It is expected that the blanket structure will operate at 500°C to 800°C
This combination of requirements mean there is almost no chance of a breeder blanket that can survive the full life of the reactor. After a few years the material properties of the blanket structure will have degraded so much that it will have to be replaced. The inside of the chamber will be far too radioactive for a person to go in there, and so a remote handling arm will have reach in through one of the ports, bending around the central pillar where required, and remove the old blanket, section by section and replace it, section by section with a new blanket disconnecting and reconnecting the pipework (probably by cutting and re-welding) without spillage. The sections are likely to weigh several tonnes. The blanket sections will have to have a fairly tight fit to protect all the chamber wall and coils, but the extreme service conditions mean that they will be significantly distorted at the end of their service life. They must not jam in place or the long articulated arm will not be able to pull them out. There is reasonable confidence that a blanket of some sort came be built to operate for some length of time but the economics of a future power station will depend heavily on how hot the blanket can run and how long it can survive before replacement and how fast it can be replaced. The remote handling arm is a major engineering challenge.
The helium generated in the plasma by the fusion reaction and any other contaminants such as material coming off the structure under severe bombardment, need to be removed from the plasma continuously to allow the reaction to continue. To this end, at the bottom of the reaction chamber there is a divertor structure where the magnetic field is reduced so that a small fraction of the plasma separates and is allowed to cool as it circulates to the point where it recombines to form neutral atoms before colliding with the divertor plates. The gases can then be pumped out and the hydrogen isotopes separated for re-injection. Although the plasma has been cooled before hitting the divertor plates the heat flux on them is still enormous. In the DEMO reactor it will be about 15MW/m². This is about 15% of the energy generated by the fusion reaction and this energy will taken away by a coolant (probably helium) and will be used to generate electricity together with the heat from the blanket. 15MW/m² is about 20% of the power density at the surface of the sun. There is even less chance of the divertor plates lasting the lifetime of the reactor. In fact the lifetime may be as short as two years and these will also need to be replaced by remote handling in sections usually called cassettes.
As well as all the other requirements that must be met by the materials of the blanket and divertor, it is important that the amount of radioactivity induced in them by neutron bombardment is minimised. This precludes the use of some elements that might otherwise be useful for alloying. Theoretically there are no net radioactive materials produced by the main reactions of the reactor since the tritium is recycled. However there will be radioactive waste due to side effects such as neutron activation and tritium embedded in the structure. This will affect both the replaced parts during the reactors life time and the reactor itself when it is decommissioned. Predicting the level of radioactivity of the waste is difficult and impurity levels will have a strong influence. However the radioactivity has been estimated to be 2 orders of magnitude less than a fission reactor and to be short lived, so that after 100 years the level of radiotoxicity will be less than the waste from an equivalent-sized coal fired power station, Keeping the radiotoxicity low will require the tritium recovery and recycling to be achieved with extremely low leakage.
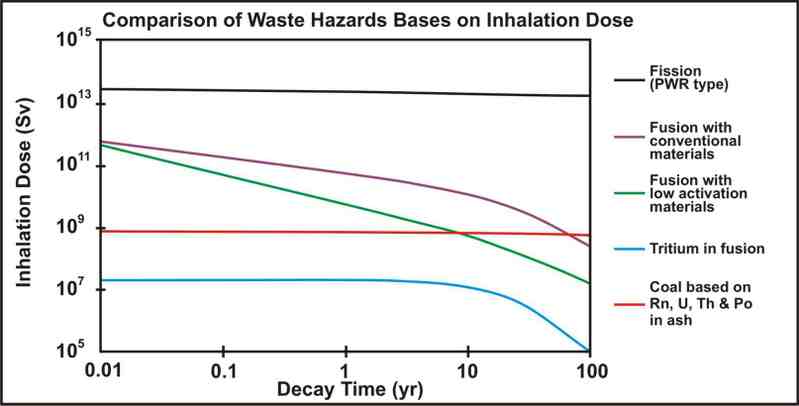
Decay with time of radiotoxicity. Click to enlarge.
The development and testing of materials to meet these very onerous requirements is crucial to the speed of deployment of fusion energy. Because ITER will not produce a plasma for almost 10 years at best and even then will not produce a neutron flux anywhere near as intense as in DEMO and that only intermittently, it has been decided to build a special facility to reproduce, over a small area, the conditions that DEMO and following reactors will have to face. This will be called the International Fusion Materials Irradiation Facility (IFMIF). This will be developed and tested in Japan as part of the Broader Approach agreement, although the final site for the installation has not yet been agreed. At IFMIF two 40MeV linear accelerators will provide 250mA of deuterons which will be targeted on flowing liquid lithium to produce neutrons with an energy spectrum up to 14Mev matching that expected in DEMO. The flux will be sufficient to produce 20 displacements per atom per year in the test samples. Further steps that will be taken to speed up development are that the JET reactor at Culham will have its present carbon chamber lining converted back to a metal wall to provide test data on this material for ITER and DEMO and the JT-60 tokamak will upgraded to have superconducting coils and to act as a satellite control for ITER. A smaller UK Tokamak MAST, which has a toroidal plasma aspect ratio squeezed so tight that it is like a spherical apple with the core cut out, will also provide input to the DEMO design.
Dr. Briscoe summarised the challenges ahead with the following table:
ITEM | Existing Devices |
ITER | IFMIF | DEMO Phase 1 |
DEMO Phase 2 |
Power Plant |
---|---|---|---|---|---|---|
plasma disruption avoidance | 2 | 3 | C | R | R | |
steady-state operation | 1 | 3 | 3 | r | r | |
divertor performance | 2 | 3 | R | R | R | |
burning plasma at Q>10 | 3 | R | R | R | ||
power plant plasma performance | 1 | 3 | C | R | R | |
tritium self-sufficiency | 1 | 3 | R | R | ||
materials characterisation | 3 | R | R | R | ||
plasma-facing surface lifetime | 1 | 2 | 2 | 3 | R | |
facing wall/ blanket/ divertor materials lifetime | 1 | 2 | 2 | 3 | R | |
facing wall/ blanket components lifetime | 1 | 1 | 1 | 3 | R | |
neutral beam/radio frequency heating systems performance | 1 | 3 | R | R | R | |
electricity generation at high availability | 1 | 3 | R | |||
superconducting machine | 2 | 3 | R | R | R | |
tritium issues | 1 | 3 | R | R | R | |
remote handling | 2 | 3 | R | R | R |
KEY
1 | Will help to resolve the issue |
2 | May resolve the issue |
3 | Should resolve the issue |
C | Confirmation of resolution needed |
r | Solution is desirable |
R | Solution is a requirement |
Timetable
A timetable has been proposed for the overlapping development of the various proposed devices. It assumes that the only obstacles to its implementation are technical ones and comes with many caveats, but it sees the first commercial power station operational in 2048. Even if this very compressed time table is met, it does not signify the widespread availability of fusion power. There is a limit to the rate at which the number fusion power stations can be multiplied set by the supplies of tritium.
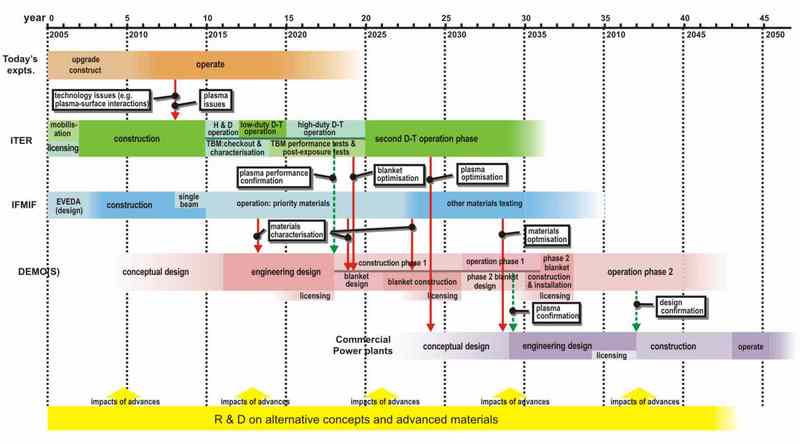
The Fast Track timetable. Click to enlarge.
Tritium Supplies
The large scale adoption of fusion energy will see tritium used on a scale vastly greater than has ever been seen before. Something like a 220kg per year of tritium will be consumed for every 1GW of continuous electrical generation, assuming that 4GW thermal will generate 2GW electrical of which 1GW will be used to provide all the inputs to the system leaving 1GW of output power. At present world-wide electrical consumption averages to a continuous 1700GW
Nearly all the worlds supply of non-military tritium comes from the heavy water used to moderate CANDU reactors and some of these will be closing down in the near future. The supply accumulated over 40 years of operation of CANDU reactors will peak in 2027 at 27kg.
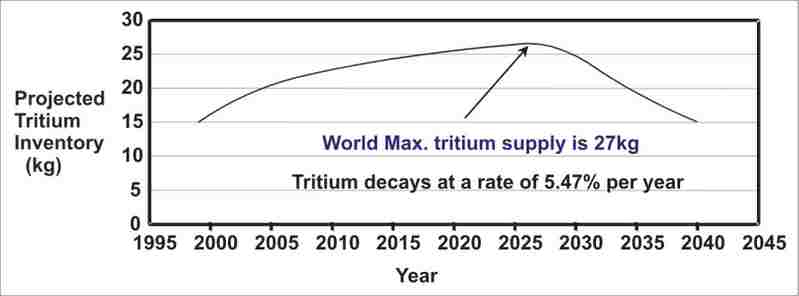
The world’s commercially available supply of tritium before any is removed by fusion programme. Click to enlarge.
Military reactors designed for tritium production produced only a few kilograms a year at a cost of about $200M/kg. Tritium increases the yield thermonuclear warheads (H bombs). It is thought that about 4g is used in each warhead, added in a container just before launch, so that decay of the tritium does not limit the shelf life of the weapon. There have been some hints that the latest warheads being designed will not use tritium. The US had a number of military reactors at its Savannah River site especially designed for tritium production but the last of these was closed down in 1988. It is believed that over 220kg of tritium was produced there over the years but that there was only about 73kg in 1995 which will have now decayed to about 37kg. It is unlikely the US military will release any of this for civil fusion power. One of the speakers said that he believed that at one time the Russians had mentioned the possibility of releasing some of their supply but had no further details. Other civil fission reactors could produce small amounts by placing lithium inside the reactor but some back of envelope calculations that I did for a comment to previous post show that it would take at least 60 tonnes of unenriched uranium to produce 1kg of tritium in a standard reactor and it may be much more. Specially designed accelerators are theoretically capable of producing tritium, and have been considered for military needs, but one to generate a few kilograms per year was estimated to cost $4.8 to 6.1 billion in 1991 prices and would produce vast quantities of radioactive waste.
Since ITER will produce only a tiny proportion of the tritium it uses (at least in the early years) because the experimental test blanket modules will only cover a small area of the chamber, ITER alone will severely deplete the worlds tritium stock. If DEMO is heavily overlapped in time with ITER the tritium supply will be very critical and it will be important to get the full blanket and tritium recovery system going as soon as possible if the programme is not to be delayed.
If fusion reactors are to proliferate, then probably near the start, and certainly after the first few, each new reactor will be relying on the small surplus tritium production from existing reactors to provide the start-up charge of tritium. This is likely to be some tens of kilograms. When I asked him, Dr Briscoe said that it will probably take two-and-a-half to three years from the start of one reactor for it to supply enough surplus tritium to start up another. The estimate he gave was that even if the only obstacles were technical ones it will be 2100 before fusion can supply more than 30% of Europe’s electricity.
The Energy Gap
All but the most dewy-eyed optimists foresee the production of conventional oil severely curtailed by then. If we are to get to 2100 without major economic collapse or using so much coal, tar and oil shale that the carbon dioxide will have risen to disastrous levels, we will have had to have adopted other energy sources on a major scale, as well as substantially cutting our energy consumption and finding some way of replacing liquid hydrocarbons for transport. Fusion will then be competing in a very different environment.
Cost estimates at this stage are obviously uncertain in the extreme but most estimates put the cost of generated electricity as comparable in today’s prices with today’s fossil fuel generated power. Most of the cost is in the capital cost of the plant amortised over the life of the plant. Running, periodic replacements and decommissioning costs coming next with fuel costs less than 1% of these and not likely to rise by depleting the richer ore, as would be the case with very widespread use of thermal fission plants. One estimate puts the capital cost at €14/W electrical for DEMO falling to €4/W for commercial plants in serial production. This link allows you to play with the assumptions and produce an electricity price.
These prices should be compared to today's fission and coal plants at €3 /W and €1.5 /W. respectively for the plant alone. However, the capital costs of coal plants do not include costs to mitigate environmental damage. Wind energy capital costs are now about €1.5/W but rarely have a load factor of more than 30%, whereas fusion plants after initial settling-in could have 85% load factor. Correspondingly more rated wind power plant would need to be installed to meet the same electrical demand. If wind power were to supply more than 30% of the total supply, extensive power storage would be needed and the transmission costs would be greater as many of the turbines would scattered across areas remote from demand.
The carbon dioxide emissions associated with fusion are again very difficult to estimate at this distance, but will also be dominated by that generated in building the reactor and its associated plant and the periodically replaceable parts. The carbon dioxide generated in fuel production and preparation will be constant and low in comparison. Fusion reactors are inherently proof against melt-down or nuclear explosion. There simply is not enough fuel in the reactor at any one time to cause one. Any failure of coolant, magnet supply or other major system will cause the reaction to die in milliseconds. There is not enough heat in the system even without coolant to breach the containment vessel. According to this report even in the worst credible accident with the release of all the tritium on site there would be no need to evacuate anybody beyond the site boundary. They are a very much less tempting a target for attack by violent political or religious groups than thermal fission reactors, still less than fast breeder reactors. If a large part of the energy gap world wide were to be made up by fission reactors using the present system of thermal reactors with once through use of enriched uranium we would rapidly be reduced to the use of ever lower grades of ore requiring more energy input. Some estimates put the point at which there is no energy gain to be 0.02% of uranium and that if nuclear energy were expanded from the present 16% to 50% of electrical generation we would be reduced to using such ores in 50 years. There has been much argument over such estimates but in the long term it is clear that if we are to rely on fission for a major part of our electricity let alone total energy we will need to move to fast breeder reactors or thorium reactors. The long development time of fusion energy may seem disheartening but that of fast breeders is not much better.
In 1946, the year I was born, the Nobel prize winner, Sir George Thomson, working at Imperial College London applied for a patent for using a gas discharge to generate controlled thermonuclear fusion. If the proposed timetable is kept to and if I live to be 102 I may just, in my dotage, see his dream brought to commercial reality. I do not know which of the two is more improbable.
There is an easier way to make fusion: Inertial Electrostatic Confinement.
Here is one promising fusion technology that is not getting the backing it needs to make or break it:
Bussard Fusion Reactor
Easy Low Cost No Radiation Fusion
So I have decided to do an end run around the government by designing an open source fusion test reactor.
Any one care to help? You can start here:
IEC Fusion Newsgroup
IEC Fusion Technology blog
I have read about that bussard fusion reactor, very interesting stuff. The Navy is still involved with it I believe. Best hopes for the bussard fusion reactor!
Great article, Nick. Thanks!
With respect to wind energy, earlier today in another forum I was discussing ways Canadian utilities could add additional wind resources to their generation portfolios without adversely impacting system stability. I thought I might share my thoughts with you in the context of this discussion:
Cheers,
Paul
Paul,
Load shifting can be a pretty big part of making renewables a larger part of the energy mix. This can be pretty cost effective and reduce energy prices for some of our largest loads. The very rapidly falling cost of solar and its anticipated trajectory make me think that storage is going to just fall out of the mix because the renewable sources are going to be so much cheaper than current sources. Wind also has a ways still to come down in price. Monbiot, for example, points out that going further offshore reduces costs because of the increased wind speeds there. You don't need as much equipment for the same power generated and delivered.
Utlities are already using power storage in a number of applications so there is already a "storage sector" that has developed. The US has about 24 GW of hydro capacity that can run backwards and most new thermal solar plants have thermal storage at least as an upgrade option. I look at some of the storage options at the Real Energy blog. Comments are welcome there.
Thanks, Chris, for the link to your energy blog and for the invitation to join you in these discussions; much appreciated.
As you might guess, I see tremendous opportunities to reduce peak demand and to better manage customer loads, especially as our electrical grid becomes increasingly "smarter". My hope is that we can use this same technology to "push" energy to end-users, so that the system is equally responsive to supply conditions as it is to demand (which, of course, allows us to better integrate various renewable resources such as solar and wind). I don't think we fully appreciate the range of opportunities available to us in terms of this push-pull dynamic. Intelligent controls combined with real time pricing could very well take us in directions previously unimagined.
Best regards,
Paul
Great post.
Don't forget plug-in hybrids (PHEV's) and EV's. Owners will pay for storage for their transportation needs, and buffering of wind generation intermittency would be a bonus. Plus, there's the possibility of Vehicle to Grid.
In the long run I think PHEV's will be the main answer to handling renewable intermittency.
Thanks, Nick. As you say, EV/PHEVs could help utilities dramatically expand their renewable energy portfolios, certainly well beyond what is possible today.
I have an oil-fired boiler and I really don't care when my service provider fills my tank, provided I don't run out of fuel. It could be today, tomorrow or sometime next week; it really doesn't matter, again, so long as I have sufficient heat whenever I need it. Now imagine if electrical utilities could operate the same way -- electricity delivered not strictly on the basis of customer demand, but whenever conditions were optimal and the cost of service was low.
In a sense, electric vehicles are a step in this direction. I get home at 17h30 and plug in the Chrysler. The next morning when I'm ready to head back to work, my batteries are fully recharged. It really makes no difference to me if charging began right away or at 02h00 in the morning, or if the flow of power were continuous or intermittent; I'm happy, so long as I'm fully charged when I'm ready to hit the road. Ideally, I would have the option of immediate, continuous charging if I needed a fast boost, but if the vehicle won't be used for another twelve or fourteen hours I could care less (a discounted rate for overnight/interruptible charging would offer suitable financial incentive).
So to recap, smart controls would allow utilities to provide consumers with the same level of service they enjoy now, but in a more efficient and cost-effective manner; any complexity would be hidden from the user and their patterns of usage would remain unchanged (e.g., they would still plug in their car for overnight charging but the utility would determine when and how power would be delivered over that timeframe). The second component is real-time pricing. In this case, utilities would charge consumers rates that vary day-by-day or hour-by-hour according to the true cost of supply. Providing this information to consumers (by way of a household display or internet website) would encourage a shift in discretionary demand to periods most favourable to both parties. Together, these two things would allow us to dramatically increase the amount of renewable energy we can incorporate into our power systems.
Best regards,
Paul
Absolutely.
For an innovative program for time of day pricing, see Thewattspot.com .
What a fascinating esoteric human folly - for something that nature does trivially with massive gravity.
Why cant these people research room temperature tea and coffee - and save us a zillion tokamaks worth of power........?
[Edited after a while..]
Apologies for not first thanking Nick for the excellent information.
Interesting to hear that even with a magic wand making fusion on earth feasible, there is a slow start to the scale up due to the need for tritium.....lets get the tidal lagoons and barrages built first, then build stars afterwards.
tritium seems to be the fav for Tokamaks, not IEC or polywell devices, many whose goal is to fuse P-B11, boron. This type of fusion creates extremely low numbers of neutrons. And there is a lot of boron.
People pushing p + B11 tend not to notice the losses due to bremsstrahlung from all those electrons.
(basically, electrons "collide" with each other at distance via electric forces, the collisions are largely elastic, but at high temperatures and densities the radiation emitted when they all change their random directions of motion becomes significant)
("bremsstrahlung" is German for "braking radiation")
(when a charged particle is deflected due to electric forces, photons are emitted)
ciao,
Bruce
The current statistics on the availability of Tritium are skewed to Earth Bound sources only.
Tritium is actually more plentiful on the moon, and in fact, there is speculation that the Race for a moon base has been given new life because of this fact.
Amongst space enthusiasts, it has been the guess that once a product/resource in space can be found at more abundance and for lower cost/lower risk than on earth, that we would then see a push for space colonies, refineries, mines, and other industrial activities which would require a human presence in space.
Tritium to fuel Fusion might be the very product that kicks this off.
Bussard wants a measly 200 million USD for his startup. $150 million if you want a bit of radiation on the side. Without a doubt someone will explore this when blackouts become more the norm. He's an exceptionally brainy guy - some say the world's foremost expert on fusing atoms. It is unfortunate that at the moment he displays much of the typical baggage of the pseduoscientist - just ran out of funding, on the verge of a breakthrough, crudely drawn graphs...
For me the crucial excerpts were:
"The Fast Track timetable" - shows the first commercial reactor producing power 40 years from now.
Dr. Briscoe: "... even if the only obstacles were technical ones it will be 2100 before fusion can supply more than 30% of Europe’s electricity."
"All but the most dewy-eyed optimists foresee the production of conventional oil severely curtailed by then [2100 - in fact that occurrence by 2025 is much more likely]. If we are to get to 2100 without major economic collapse or using so much coal, tar and oil shale that the carbon dioxide will have risen to disastrous levels, we will have had to have adopted other energy sources on a major scale, as well as substantially cutting our energy consumption and finding some way of replacing liquid hydrocarbons for transport. Fusion will then be competing in a very different environment."
In other words, the development of fusion under the schedule shown will be so slow that as a solution, it will be entirely irrelevant by the time it can produce any meaningful amount of energy. Why?
A) If we find no usable alternatives to oil and gas, our present society will transition - peacefully or otherwise - to appropriate technology and sustainability based mainly on renewables. Expensive high-tech fusion approaches will then be irrelevant.
B) If we "burn everything" the most likely outcome is massive climate change, large-scale melting of the Greenland and West Antarctic ice sheets (paper of James Hansen et al., published May '07). Results - catastrophic environmental damage leading to economic collapse, and fusion irrelevant as no organised effort will be able to be made to complete development.
A massive fission programme may be what politicians are hoping will bridge the decades-long gap from fossil fuels to fusion. Maybe others will discuss this in detail, but it seems doubtful that there are the will or resources to build enough in time. Otherwise, IMO, we should either have a Apollo/Manhattan Project scale of effort to develop fusion over a timescale of a decade or two, or forget it. It seems to me that the current level and pace of effort is little more than a political sop to keep fusion proponents happy and quiet.
If your into science, and into earning money. going into fusion research is the best thing you can do, all you need to do to increases your paycheck is tell the people who give the money(governments) that you can 'solve' the current issues, but it can't be done without a increases in funding.
Bottom line: Time's up!
It was an interesting idea well worth researching, but we've lost our window for development and deployment.
Would a larger and more intensive crash program have achieved more timely results? We can speculate, but we'll never know.
I agree, it's a case of too little, too late..
I remember a lecture by my old nuclear physics prof. back in 1993, when we studied fusion, and he basically said that if it weren't for vested interests (ie. Big Oil & car manufacturers, and even governments) we would already have fusion reactors, but funding was almost nonexistant for so long that they never made much progress.
Great article though, I really appreciated the update!
I would like to point out that the time-scale for fusion development was set back in the seventies and was based on the idea that we would run out of fossil fuels at the end of this century. Two things seem to have gone wrong: 1) we've used more energy than anticipated back then, and 2) the coal resource was probably over estimated. But, it is pretty hard to speed things up because you need to first ramp up physics departments to train students who can go to work on an expanded program. The other areas where plasma physics is used a lot, weapons research and astrophysics, probably can't supply an immediate workforce large enough to make a big difference.
I agree that it is hard to see an economic home for large-scale fusion. It's pricing will look like fossil fuel pricing, but renewables are competitive now with fossil fuels and headed much lower so fusion will have to come in much cheaper than anticipated in the article to be considered feasible.
The only role that fusion might play with regard to global warming seems to me to be in a situation where we are faced with a natural runaway and are willing to pay quite a lot to remove CO2 from the atmosphere quickly. An extra energy source might be handy for that. I am intrigued by Dave Rutledge's idea that remaining hydrocarbons may be so few that we won't reach "dangerous" climate change as described at Exeter. I've linked him in at the third paragraph here. (The linked article is about making cellulosic ethanol without having to hassle with existing patents.)
Since, there is not a good way to know really how expensive fusion will be, the current rate of progress should be maintained I think. Just knowing if it can be done is worth pursuing.
Research wise there is more out there than just the ITER and the Tokamaks, as M. Simon points out. A few notes:
1) Bussard needs 5 million to continue work, 2 to 3 yrs.
2) 200 million funds work thru a proof of concept 100 MW reactor, 5 yrs.
3) P-B11 fusion is preferable, far cleaner than D-D or D-T.
4) I know of one Foundation director who has contacted Dr Bussard concerning possible funding per item #1.
IEC fusion vs Tokamak fusion:
http://www.youtube.com/watch?v=rBfsq80EgOs
I'm not qualified to comment on Bussard's work. All I would say that if we are serious about fusion, there should be a massive project to look at ALL reasonable means of progress and take them forward. The Manhattan Project included many dead ends - Bussard's ideas may be one or maybe not. I think we are looking at a timescale of 15 - 20 years max. for a first commercial reactor on-line. Later than that nobody will be interested.
Yes, Yes, Yes, I agree completely, its just dumb that the Doc has to look for 5 million in VC just to build a few more devices on the way to proof of concept, or that any other reasonable fusion research program has to scour the woods for scraps.
Compared to the cathedral that the Tokamak program has become whats a few tens of millions to be sure these other areas of research are truly dead ends?
Without wishing to sounding too picky - the Manhattan project colleagues had a working reactor within a year, that could produce heat continuously [with fuel] although it probably had no adequate safety design [other than buckets of heavy water]. Doesnt that tell you something?
Ummm. No. What's your point? Fusion is one helluva lot harder to achieve than fission.
The first IEC fusion device was producing fusion in 1959.
However, losses were too high for power production.
Bussard looks to have solved the losses problem.
Fusion is easy. Net power is hard.
ITER is doing fusion the hard way with net power 40 years away or more.
There are many problems with Inertial Electrostatic Confinement devices such as Robert Bussard's polywell.
Even D-T fusion is problem and p-11B very much more of a problen. These problems have been analyzed by Todd H. Rider amongst others.
The Lawson criteria, the triple product of plasma density, confinement time and temperature at the optimum temperature is about 500 times as stringent for p-11B as it is for D-T if the system is thermalised. The ion temperature needs to be about 132Kev (about 1.43 billion degrees K in a thermalised system against 13.6Kev for D-T(158 million degrees).
The crucial point is whether the ions, after they have been injected at high energy can be kept at a significantly higher energy than the electrons, (i.e. not thermalised) not just in an experimental set up, but at the density and confinement time needed for a commercial reactor. If the electrons get anywhere near this temperature they will start emitting huge amounts of 'bremsstrahlung' radiation. Focus fusion hopes to use the magnetic field effect due to fields of 1.5 megatesla to prevent thermalisation. At such fields the cyclotron orbit diameter is less than the DeBroglie wavelength and energy transfer is prevented. The polywell will not have such fields. Todd H. Rider believes that even at the best configuration bremsstrahlung losses in a p-11B system will be 1.7 times the fusion output thus preventing any hope of energy gain.
Trying to extract the energy will be difficult in a commercial reactor. There is the theoretical possibility of retarding the alpha particles and electrons against an electric field and get direct conversion but in a system that already relies on a complex electromagnetic field to create the fusion it is difficult to imagine how the retarding fields could be arranged.
If the energy of the reaction products is thermalised in a blanket most of the energy will be carried by the alpha particles which will be absorbed in a very short distance of the blanket wall. In commercial scale tokamaks the much more dispersed absorption of the energy of the neutrons give a power loading that causes severe problems. The near surface absorption of a blanket polywell system will, I suspect be a much greater problem.
The magnetic mirrors of polywell devices also sound a caution to me as I remember the problems of magnetic fusion devices using mirrors where losses were much greater than first predicted. Ions or electrons moving towards the mirror exactly on the axis are not mirrored and escape. The mirror can be designed so that the cone of trajectories that can escape is a small fraction of all possible trajectories so that for low particle densities the losses are manageable but as the density builds up and the thermalisation time drops the cone of trajectories that escapes (and is therefore missing in the distribution of trajectories) is rapidly refilled by thermalisation and the fraction of particles that escape the mirror rises. To achieve commercial power densities the plasma density will need to be high.
It has been pointed out that Bussard's claim of 109 fusion reactions a second is based on just three detected neutrons. There are at the least wide error bands on that claim.
As with magnetic confinement systems I suspect there is a massive gulf, and decades of work, between demonstrating fusion and even demonstrating energy break-even and producing an economically viable commercial system. I support giving it a chance but I think it is a long shot at best.
1) Read page 3 of Riders thesis to see who paid him to write it.
http://dspace.mit.edu/bitstream/1721...1/31763419.pdf
2) Rider now works in........ biology.
3) Rider never disputes the possibility of an electrostatic potential well forming.
Isotropic velocity distribution in a plasma will always mean rapid Maxwellization. But particles approaching the focus from all sides =/= "isotropic velocity distribution" any more than a linear accelerator has an isotropic velocity distribution.
True enough, once you prove Maxwellianization will occur, isotropic velocity distribution will be an aspect of the resulting plasma. But starting with that assumption is rather circular.
4) His "maximally efficient system for maintaining a non-equilibrium distribution", the basis of his model, (page 69 if you're looking at his dissertation) is an unnecessary application of heat thermodynamics that leads to the search for "recirculating power," a nonsense concept for an accelerator.
5) Bremmstrahlung Radiation Losses in Polywell Systems", shows Riders thesis is wrong under the HEPS device results. contracted under DARPA. Notice the dot mil suffix.
http://stinet.dtic.mil/cgi-bin/GetTR...c=GetTRDoc.pdf
6) Focus Fusion is in the same area, they refute Riders thesis.
7) Tri Alpha Energy just got 10 mill on a total of 40 mill of VC based on...
refuting Riders thesis.
Rider wrote in 1984, there has been too much research in Urbana, Univ of Wisconsin, as well these private ventures since then all based on rider being not exactly right. Based on IEC devices not creating maxwellianized thermalization.
Low to No Bremmstrahlung Radiation Losses, Low to No Maxwellian thermalization.
P-B11 fusion should start around 50 KEv to 65KEv.
Oh come on; This shameless boosterism is a transparent illustration of hope following hype. Dead simple polywell devices would change the world dramatically in huge ways. So you have people constructing these attacks on Riders sound thesis with every plausible counterargument, because then there's hope of this techno-utopia brought by this disruptive technology. Along with lack of experimental evidence (save three whole neutrons!) coupled with with a convenient destruction of the only test device. This reads like a wonderfully self-deceptive scam from the previous booster of the Riggatron.
Paul Dietz summerized it in sci.energy not too long ago.
1) Bremmstrahlung losses happen whenever you have electron-ion interaction so the interaction zone can't be a near neutral plasma. Fusion power would be eclipsed by losses.
2) Non neutral plasma current density is limited by the space charge limit.
3) Focusing the ions to the high density central peak to overcome the space charge limit is unstable. When they miss, they're taken out of the game.
What I expect to see from the polywell configuration is yet another plug-in neutron source that happens to be better than the grid based fusor but still very far from being able to provide net power. One can dream though. It would be nice. So would Woodwards impulse engine, nonlocal quantum communicators, or magic pixie dust.
@Nick
That was a pretty good review of the problems. Plasma physics is a complicated subject, on which any independent thinkers have to do, really a lot of, homework. Most people need several years past PhD to gain real breadth in this subject.
@the other guy
With that argument pretty much all of scientific research would have to pack up and go home. Of course DOE funds the stuff. In the US, no one else is interested, because the horizons are too far off. In other countries it's the same.
ciao,
Bruce
Is an encouraging potential idea, but its really long term thinking. Its likely we will make an advance sometime in the future but would be much better to be concentrating on using carbon nano-tech to create long distance super conducting power lines and improved materials for marine energy systems, light weight rail, insulation and electrical energy storage.
Oh yeah a fair amount of birth control wouldn't go amiss either.
The Mother of all Technofixes, born 1952 I believe. My gut feeling is that if an innovation has legs it moves fairly quickly. If that intuition is correct it suggests that plug-in hybrids will be prime time in 10 years but fusion will have barely progressed.
An excellent piece. The key is again illustrated by the author. That proton charge barrier is the issue. I admire that the piece offers some hard details on what would be required to get the tokamak running. 15 million amps without even mentioning the voltage makes one sit right up. The problems of compressing identical elements with thermal energy is a monumental challenge. A lot will be learned over the course of the project.
Bussard on the other hand seems to attack the proton charge barrier with much more elegance and human ingenuity. He proposes using the high velocity from the device's use of the fuel's own properties rather than brute heat to combine a free proton to a nuclei of boron, thus changing the "rules" to get a fusion going from a nucleus/nucleus fusion to proton/nucleus fusion. But the changing the "rules" is what confuses observers.
Its a fascinating competition. Raw power vs. ingenuity and elegance. But the game will be decided by the cost per kilowatt output, and if Bussard is right, he'll win not only coming out first, but on the merits of the financial analysis.
Right now Dr. Bussard is in the "tantalizing tidbit" mode of getting information out, and that seriously handicaps his funding effort. And by no means has the challenge of getting a fusion going been exhausted of ideas.
But for a serious discussion, the Bussard process needs the details and the skill of presentation offered here. A fine job, thanks for the posting.
Inasmuch as there's really no time or energy to build out ITER-style tokamaks as any kind of global solution, alternate fusion schemes should be looked at in much greater detail.
I have for decades been interested in the inherent elegance of IEC-type designs, and agree that there is little to lose in a crash program to establish or negate their feasibility. If there is a "magic" solution - dubious - then this is one of the few places it might be hiding.
Coincidentally, I am experienced at securing significant funding for projects and have communicated directly with Dr. Bussard over the last week specifically regarding a coordinated international plan to pull together the necessary funding for the work he has proposed doing. Unfortunately, it turns out he's not willing to do much to participate; from helping create a lucid lay refutation of his critics to actually meeting with potential angel investors; he just wants them to find him and give him money or say the hell with it. I hope they do so, and I hope they can get past his attitude, because I am predisposed both towards the technology and to his work and his attitude was entirely dismissive. I'd quote his text here if not for privacy concerns.
Indeed, I was left wondering whether he doesn't have more unsolved technical problems than he has admitted. I'm afraid that I came away from the exchange thinking that he's entirely fine with not being funded and not being involved in proving his contentions.
I would still be interested in helping obtain funding and popularizing this approach if connected with highly credible fusion researchers. It seems that will not be Dr. Bussard.
DJ, thats a shame. That should be overcome. I'll have to twist his arm.
YO! I assume by the comment's position you mean to reply to greenish's comment. I hope you know Dr. Bussard and can talk candidly with him. But I hope everyone respects his wishes and look to find a solution that satisfies him as well as the rest of humanity. I would like to help, too. But I could only move forward with Dr Bussard knowing I and others who I associate with will have his back covered as everyone works to go into the future. This goal is going to require a lot more than money to get to commercial. And somehow I can't imagine that Dr Bussard having spent 12 years in the scratch mode of research, getting this far and having the journey just stop on him has a solid number of "$2.0 million" Having said that I'm thinking $8 to $10 million would be more on point.
MANAGER-BW,
Dr Bussard indicated to me that 5 million buys the next 2 test devices. About the size of the last device:WB6, 30cm on a side. A truncated cube=WB7 and then a truncated dodecahedron=WB8. This is w/o any cooling, so pulse operation is mandated. 200 million equals research thru proof of concept, net power.
LN2 cooling would likely add several+ millions to a single device, but would allow for continious running vs pulse. And would possibly allow for drive levels in the 50-65KeV area, where theory says P-B11 will fuse.
Proof of P-B11 fusion would blow the whole issue wide open. This is the goal of M. Simons open source engineering project.
I was very pleased to see that someone has corresponded with Dr. Bussard. And with great sympathy I hope that greenish can get past the first impression and assess what is necessary to bring Dr. Bussard's creation into practical use. For those of you who haven't followed Dr. Bussard we haven't a man eager to build a new empire through entrepreneurship, rather Dr. Bussard is advanced in age, whose health is of considerable concern and brings forward a set of principles perhaps more old world than new age. But, never the less, no one understands the concept more thoroughly than he. The loss of Dr. Bussard's knowledge and insight is more likely critical than instant funding, and should we as a culture postpone adapting ourselves to his abilities expressed within his needs rather than requiring him to adapt to ours may well lead to a tragedy of incredible proportions. Those of us who hope to bring his work to fruition may best spend our first efforts in seeking the path that works out a compromise with the needs of one with the needs of the many.
Manager-BW says:
The loss of Dr. Bussard's knowledge and insight is more likely critical than instant funding, and should we as a culture postpone adapting ourselves to his abilities expressed within his needs rather than requiring him to adapt to ours may well lead to a tragedy of incredible proportions.
Six months ago I would have agreed with that assessment. At this point I do not believe it is true. There are in my estimation at least 3 engineers and possibly as many as 30 who could design a test reactor based on information currently available from open sources.
The test reactor will fill the holes the Dr. has left in his information output.
A lot of this increase in understanding is due to the work of many in the blog/newsgroup I have mentioned above and the work done at the NASA Spaceflight blog. There are at least 50 to 100 people now working to drive this forward with 10 to 20 providing significant efforts in terms of commitment.
What has been published and discussed is sufficient that even if the Dr. would die tomorrow the work could go forward without significant loss.
Earth Trust has been around since 1977 and is well known in Conservation & wildlife circles. I thought it would be a perfect match with the Doc, but what do I know.
Very likely spot on. Well said.
greenish,
I have designed an open source version of the Bussard reactor. See the IEC Fusion Tech blog link above. If you can secure an option on Bussard's patents I'm more than willing to go forward with a test reactor. The design outline is sufficient for doing presentations. It will require a fair amount of effort to bring it to a level where a test reactor can be built. Mostly mechanical engineering. I can do the electronics.
I am confident of the physics (all of the Drs. claims I was sceptical about have been confirmed by the research of others). I am absolutely sure of the engineering (my field of expertise).
I'm sure Foger and I would be willing to make a presentation.
BTW should it be of any interest Foger and I are on the opposite ends of a lot of political questions yet we have both buried that in our interactions to work on Bussard's design.
This really transcends politics.
Also: Foger has done an excellent job of explaining and countering objections here. His work on this is first rate.
Also note that the Monkton/Rostoker design has much less open and critiqued material available than Dr. Bussard's work.
In addition it is very possible to get Tom Ligon on board. He actually worked for Dr. Bussard for 5 years and has significant insight as well as experience in solving the problems in Dr. B's design.
I have been lead engineer in a number of projects in the $1 to $10 million range from design to schedule(logistics) to implementation. I am also used to working with a small support system i.e. subject to financial controls I have done my own purchasing for various projects. Vendor negotiations etc.
Plus I have a reputation for driving projects to completion including doing miracles with schedules on other people's projects that were terribly out of whack. I can give details and references if you want more information.
Let me also say that I have a rep for being "difficult" because I do not tolerate stupidity or fools gladly. However, that is also the reason I get things done. Also note that I have no problem with ignorance. That can be cured with education.
My main field of expertise is aircraft electrical systems. With a side of fission reactions thanks to the US Navy. (I'm nuclear qualified) I understand the heat transfer problems and can do design in that area or at least supervise it.
Thanks for the comments. Anyone wishing to contact me about this may do so by clicking on my user name.
The reality is that there needs to be a breakthrough in power technology. Efficiency is the name of the game. It doesn't necessarily need to be fusion; an 80% efficient solar panel would do fine. There are other fusion projects beside Bussard that look like they can actually produce fusion. Look at www.freeenerynews.com.
I don’t think that combustion energy has much of a future, the non-breakthrough cards are all on the table and they don’t look too good. You look at the recent advances in solar power and see it’s coming from the private sector, the entrepreneurs smell profit! This needs to happen in fusion power, developments that can come from private financing, this will push everything forward at an accelerated pace
One thing I've always wondered about is if a combined fission/fusion device is possible. Nuclear bombs work on this concept but they are just using the pressure of the blast.
In the case of a combined system it would also include energy transfer to the field and by thermal collisions.
Just wondering.
http://www.ippe.obninsk.ru/podr/tpl/pub/html/1/ref1a.html
http://www.lbl.gov/Science-Articles/Archive/sb/May-2004/01-AFRD-HIF.html
Nick,
Thanks for a great post. I particularly appreciated the clear discussion of the severe requirements for the blanket. It seems extremely challenging!
Thanks (again) for the excellent, extremely informative article.
However, there was no discussion in the article or comments about helium, which is crucial for low temperature superconductivity. Current high-temperature superconductors do not have the crucial combination of strength and high current capacity needed for confinement fields and so all magnetic confinement fusion reactor designs require a large amount of helium. Helium is an element produced by radioactive decay of thorium and uranium in the crust and has been concentrated in a small number of gas wells (e.g., in Texas). It is on a similar depletion curve to natural gas (probably already past peak).
Helium could theoretically be produced by a working fusion reactor. Given the depletion rate of non-renewable helium, however, one wonders whether there will be any left to start the first continuous fusion reaction. When helium escapes from pressurized dewars (like the one in the MRI magnet in front of me), it actually diffuses out of the atmosphere into space to be lost forever.
Many years ago I went to see JET being constructed at Culham, and we had a small presentation. I don't recall if they mentioned the huge technical problems facing fusion power, but your article covers them well.
I would be interested in seeing some estimates on the economics of operation. In particular, high capital costs would seem to be a major obstacle, and if you throw in the need to essentially rebuild part of the reactor every few years, that would surely rule out a viable commercial prospect.
Certainly the hype of "clean, free, unlimited" fusion power will never become a reality.
We have had a large working nuclear fusion reactor for years. Every large population area is about the same distance from it. Approximately 93 million miles, in fact.
In view of this and the very high probability that nuclear fusion will never be viable on Planet Earth, all work on man-made fusion should cease immediately.
The funding should be re-directed to the mass production of the most important technology for the 21st century, electricity storage devices based on physics, not chemistry.
Glad to see some one promoting flywheel technology.
I had an interview with a company with a SBIR grant (small business innovative research) to make flywheels with magnetic bearings. Didn't get the job. I have to ask promoting flywheels for what?
Their specific energy isn't much better than batteries. And that doesn't count the containment vessel if they shatter. Batteries have safety issues too of course. They have much better specific power than batteries, but they are usually competing against capacitors for applications wanting a short pulse of energy. Flywheels have found use in smoothing the output of a multi-stroke piston engine. Initial capital outlay much higher than batteries. They last forever, but if they outlast the car or whatever they are powering, so what.
Interestingly, JET at Culham uses flywheel storage to extract energy from the grid at a steady rate and to supply it in pulses for the fusion shots. There were described at the meeting I was reporting in the post.
There are two flywheels formed from spare multipole motor/generator units from that other energy storage scheme, Dinorwig pumped storage scheme. The rotors of each generator is 9 metres in diameter and weighs 775 tonnes
Each JET pulse consumes around 10 GJ of energy, with the peak power requirements exceeding 1000 MW. This amount of power is two big for the connection to the UK National Grid
Before each pulse the flywheel is accelerated by its 8.8 MW electric motor Each flywheel can be spun up to 225 rpm so that the edge of the flywheel rotates at the speed of 380 km/h That is where the rotor carries the pole windings positioned close to these rotating windings are the stationary pole windings mounted on the stator.
When power is needed for a JET pulse, the rotor pole windings are energised. Magnetic forces slow the flywheel down to approx. 112 rpm.One generator supplies the toroidal field coils, another the poloidal field coils.
Flywheel storage may not have better storage density than batteries but it has a much better cycle life, especially under high power pulse duty such as this.
That same system is used here too (ASDEX Upgrade), practically on every tokamak as far as I know. As you note, the short term power needs are extreme and therefore we store up the needed energy before doing a series of discharges. I don't know when this idea originated but my impression is that it is decades old.
ciao,
Bruce
Neat. I didn't know that. Thanks. Flywheels have an order of magnitude or two better specific POWER than batteries besides lasting forever. I though >1000hp electric motors used banks of capacitors. Maybe power factor is important.
Fermilab is Commonwealth Edison's third largest customer. Which is why all the experiments are run at two in the morning. I don't know who the first two are, but my guesses are an integrated steel mill and the Metropolitan Sanitary District pumping water all over.
IIT did some work on mass drivers. They generate megaamp currents to produce megaguass magnetic fields. To get sufficient specific power, they use high explosives. Reusability is a big problem with mass drivers.
Nick, thanks very much for a very informative and interesting article.
My one major problem is you quoting that site that claims a light fission "break-even" at a mine grade of 0.02%.
The Rossing mine in Nambia operates at an Ore grade of 0.035%.
It produces around 3000 tonnes of uranium per year. A 1 GW light water reactor requires around 200 tonnes of natural Uranium per year, so Rossing supplies 15 GW of electrical power per year. On an annual basis this is around 450 PetaJoules of energy. The energy cost of operating Rossing is 1 PJ per year. So the energy gain from Rossing is over a factor of 400. This is well over 2 orders of magnitude above "break even". You get similar results from analysing other low grade Uranium mines.
Please, lets move on from that 0.02% Ore grade meme. It is demonstrably incorrect from a simple back of the envelope calculation and a bit of googling.
Why are you only taking into consideration the energy of running the mine? That's like saying the energy cost of bringing food to your table is equal to the energy required to plow the ground it came from.
"You can never solve a problem on the level on which it was created."
Albert Einstein
Using gaseous diffusion based enrichment might get the energy cost of running an entire program up to 10% of generated energy, but the remaining 90% is available for useful stuff. This demonstrably true. Nuclear energy supplies 80% of France's electricity which has a totally integrated nuclear cycle within it's own borders, except for Uranium mines.
The essence of the argument that Light Water reactors will not be profitable at Ore grades below 0.02% is that the energy cost of running the mine is meant to exceed the net energy generated from the Uranium.
I'm pointing out that Ore grades of 0.035% represent around 0.2% of the energy output of Light Water Fission reactors. The argument is wrong by over an order of magnitude.
Perhaps I'm biased having been trained as a physicist, but the problem with modern fusion programs is that they have moved into the engineering phase too quickly and this has caused basic research to be neglected.
There may still be physical effects that have yet to be discovered that could offer alternatives to magnetic confinement and laser-based fusion. There was a flurry of interest in sono-fusion a couple of years ago, the shoestring sized project is a perfect example of the type of neglect I'm referring to.
In my opinion what is needed is a worldwide coordinated effort to explore all types of fusion reaction mechanisms. I estimate a good effort would cost about $3 billion per year. This may sound like a lot, but people in the US spend over $1 billion just on vitamins.
Even if 99% of the projects turn out to be dead ends we only need one winner. Why the world persists in being so shortsighted and thus ignoring meaningful fusion research remains a puzzle to me.
The energy crisis will be the defining issue of the 21st Century. Shouldn't it be getting a full court press?
Absolutely, and I'm glad you made this point.
Sonoluminescence hasn't worked to create fusion of course, but before that was demonstrated, who the heck would have thought that you could get that kind of temperature that easily & cheaply in this universe?
Early man probably first got fire from lightning and kept it going. Only much later was it discovered that you could accomplish the same thing by rubbing sticks together in a specific way.
Fusion energy is "in there", and its a pity our civilization has grown like yeast instead of maintaining a sane size for long enough to understand the subtle workings of the real world a bit better. There may well be a cheap and easy route to fusion that we simply never find. I suspect that's the case.
Of course, giving unlimited energy the the culture which exists now would not make it wiser, and humans would only come up against different Liebig limits a bit further along. But it might prevent tipping the global methane positive feedbacks into 'anoxic ocean' phase.
I wish I had more time for this forum.
Thank you for bringing this up. A well researched article, to a point. The alternatives and the physical questions are out there for anyone who chooses to look for them. Unfortunately, there is a certain grant-based mindset that depends upon the MIT version of physics for fusion money.
The hot fusion trail is a dead end down a long road. Anything that creates radioactive waste (the reactor, for instance?) over the LONG term is a dead end.
Fast tracking it is just digging a bigger hole with money that should be spent on other things, or not spent at all.
There are holes in physics, and they usually involve things which are miraculously 'found' when someone actually stops looking in the usual places for the same old solutions.
I distrust the hot fusion plan mostly because it involves too much money (I was a big fan of it in the '70's). Anything that costs a lot of money cannot be a viable solution in a future where there won't be an economy to support it. Our current economy IS oil. Our future economy will be food and water. Can we skim enough overhead money from our agriculture system to pay for hot fusion reactors which won't improve organic agriculture?
http://www.infinite-energy.com
http://www.acresusa.com
http://www.newworldeconomics.com
This is a great article and the 2nd time I have read it from start to finish.
If we assume that the energy crisis will impact during the next 10 year then its likely that ITER/DEMO will be overlapped/fastracked (along with probably hundreds of other energy ideas -I really hope the IEC thing doesn't turn out to be a turkey, it's a wonderfully simple concept).
Anyway, this Tritium problem is the spanner in the Fusion works -why hasn't this issue been made more widely known?
Any stop-gap energy 'alternative' big enough to bridge the oil/gas>Fusion gulf is likely to be big enough to solve the problem outright (unless it's fission/coal).
Consider, once we start rolling out thin film PV on scales needed to make a serious impact on Electricity supply manufacturing costs will plummet to the point at which Fission/Fusion/Etc. look like grand folies...
Nick.
Cadarache, the site for ITER, has a problem. It sits atop a fault zone in the Durance valley in Provence that had a force 8 earthquake in 1938. Cadarache currently contains a plutonium recycling centre built to withstand only a force 7 earthquakes. Another big earthquake and ITER (and Provence) will be history.
Then it is a good idea to build more CANDU:s and collect the tritium in case it all works out.
How much helium will a running plant produce compared to its inventory and leakage?
California to fund Dr. Bussard's research.
What does Schwarzenegger know that the wikipedia does not?
The physics.
Schwartzenegger must know it works since he comes from the future.
Wonderful, IF true. You just couldn't make this stuff up.
(unless someone did, of course)
One-upped by the Terminator, and glad to be, again IF it's real.
On the other hand, Dr. B didn't know about it on Sunday, he was ready to tell the world to take a hike and send all his work to the Chinese.
Girly-men finish last. Go FUSION!
On to the next Liebig limit! To infinity and beyond!
California is making direct investments in stem cells, why not fusion or any other promising technology? The companies that come from this would most likely be located in California, creating high paying jobs and tax revenues well into the future.
It is up to the voters to determine whether or not they want their tax dollars used for this. The stem cell program was funded with bonds, but so was the $15 billion dollar deficit to cover the state payroll that Arnold authorized during the downturn.
drupal hiccup created double posting; now deleted
greenish,
Back to the real world.
I'm still (more than ever) interested in putting together a program.
Tri Alpha Energy has gotten funding for a similar type device with a different configuration. They cite Dr. Bussard's work.
False Alarm
Nick, Thanks so much for the excellent writeup.
I think an increased effort to do R&D on terrestrial fusion is warrented. However, from my understanding, gravitation confinement reactors (like our sun) solve all of the confinement issues. When the reaction gets too hot, the reactor expands thus reducing plasma density and temperature. Also, the thermal blanket is 93 million miles away and thus avoids the intense neutron threat.
Given that, I also believe an intensified effort to research solar energy collection schemes in all of its forms, photovoltaic, solar thermal, wind and biomass should be seriously undertaken. At the same time, efforts to deploy today's technology on all of these fronts should also be stepped up.
We've got the thermal blanket, we just need to extract the energy impinging upon it, and figure out how best to distribute it both temporally and spatially.
It turns out that the announcement was a false alarm:
http://www.classicalvalues.com/archives/2007/07/fusion_false_al.html
A great summary of current state of fusion science and engineering. Thank you so much.
Looks like I made the right choice back in 1972 on entering nuclear engineering school - fission rather than fusion.
A comparison of human energy needs and fusion design paths continues to show a gross mismatch. Even if a tokamak burning tritium worked, who would or could invest in it?
Essentially, we are on a path to produce a design that costs too much to build and operate that uses unobtainable fuel. A ratchet of design conditions to exploit real fuel supplies (deuterium, helium-3, etc)is ORDERS of MAGNITUDE more difficult yet.
Claims of alternative design approaches flourish with perceived crisis. I wish them well but will require hard facts, full disclosure, and intense scrutiny before I endorse any of them.
The bottom line remains - the fissioning of uranium and thorium is the only large scale energy source we can plan on for the foreseeable future and must be the foundation of our energy policies.