Low energy return on investment (EROI) need not limit oil sands extraction
Posted by Rembrandt on June 10, 2013 - 4:27am
This is a guest post by Adam Brandt, Assistant Professor from Stanford University, Department of Energy Resources Engineering.
1. Introduction
Low energetic returns (e.g., EROI, NER) from oil sands extraction and upgrading have been noted as a potential limit to the development of the oil sands as a substitute for depleting conventional oil resources (e.g., Herweyer and Gupta, 2008). In this article we will examine this claim from a variety of perspectives. Specifically, we will examine the following questions:
- Are the energetic returns from oil sands extraction lower than conventional oil?
- How have the energy returns from oil sands extraction varied over time?
- What energy sources are used in oil sands extraction, and what are the implications of this sourcing for net energy availability from the oil sands?
- Will low energy returns limit the net output of energy from the oil sands industry?
This article is based on the peer-reviewed journal article: Brandt A.R., J. Englander and S. Bharadwaj (2013). The energy efficiency of oil sands extraction: Energy return ratios from 1970 to 2010. Energy.
2. Are energy returns from oil sands extraction lower than conventional oil?
Previous works have calculated the energy returns from oil sands production using a variety of methods (Peter 2010; Herweyer and Gupta 2008; Rapier 2008; Peter 2012; DOE 2006). These studies estimate EROI from the oil sands at values ranging from 2.5 GJ/GJ to 7 GJ/GJ. Most of these estimates were generated quite simply, relying on a limited amount of data. Because of the different system boundaries used in each study, as well as other methodological differences, determining the exact reasons for variation in these estimates is challenging.
Our recent work (Brandt et al. 2013) utilized detailed energy production and consumption data reported by oil sands producers from 1970 to 2010 to examine trends in historical energy returns from oil sands extraction. The system diagram and flows considered are shown in Figures 1 and 2. Data are available on a monthly basis for most flows, with limited interpolation required in mining datasets, and some back-extrapolation required for in-situ energy intensities (see Brandt et al. (2013) for methodological details). The resulting energy inputs and outputs from mining and in situ production are shown in Figures 3 and 4. Mine mouth (e.g., extraction only, excluding refining) net energy returns (NER) for the entire industry were found to be 5.23 GJ/GJ in 2010. In situ NERs were approximately 3.5 to 4 GJ/GJ in 2010 while mining NERs were approximately 5.5 to 6 GJ/GJ.
Conventional oil EROI metrics commonly show energy returns of order 10-20 GJ/GJ (Gagnon 2009 et al.; Guilford et al., 2011; Dale et al. 2011). Therefore, oil sands projects exhibit demonstrably lower energy returns than conventional oil operations.
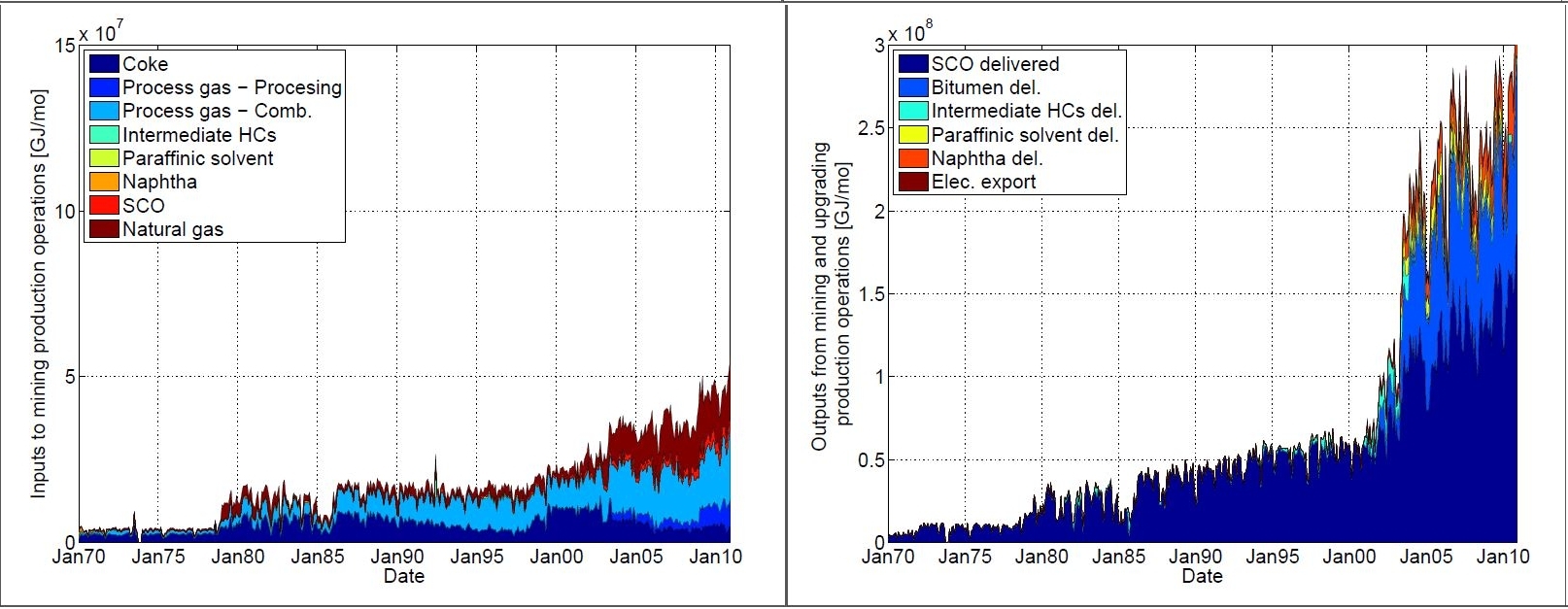
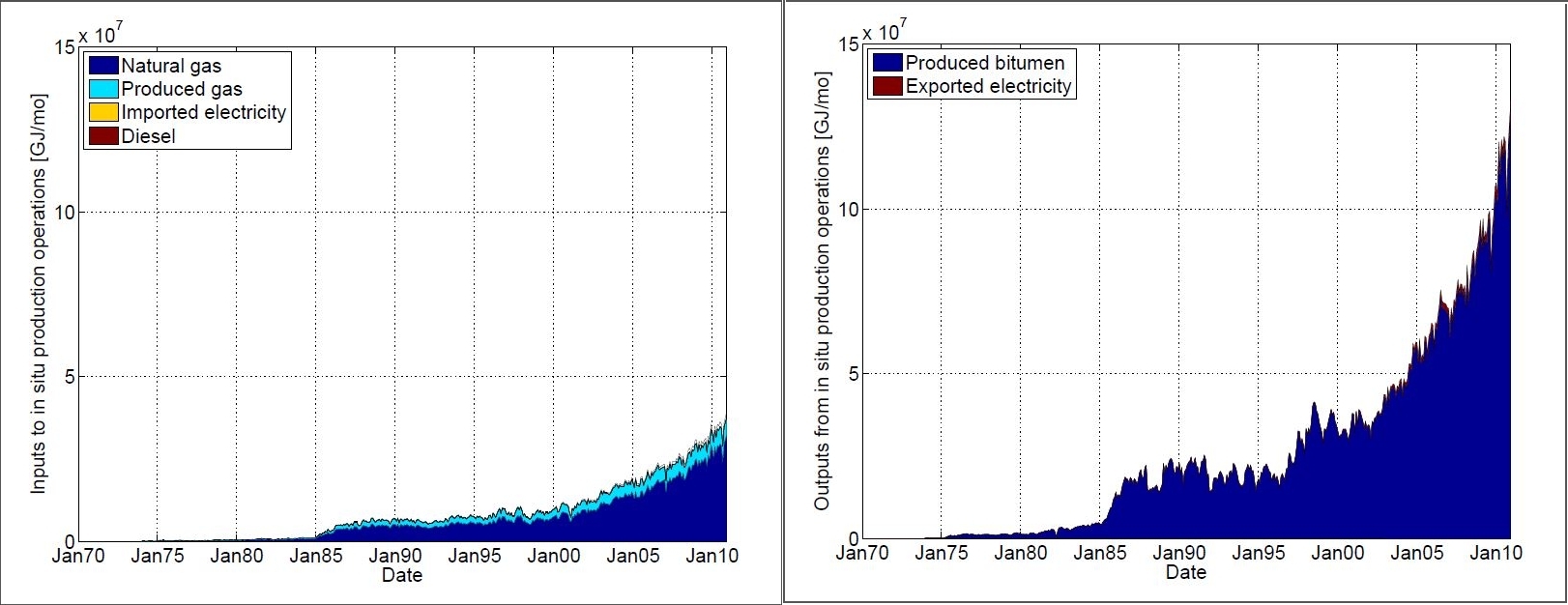
3. How have energy returns from oil sands extraction changed over time?
Net energy returns from mining and in situ operations (again, measured at a mine-mouth system boundary) have increased steadily over time, growing from 1.0 GJ/GJ in 1970 (entirely from the Suncor mining operation) to 2.95 GJ/GJ in 1990 and then to 5.23 GJ/GJ in 2010. As shown in Figure 5, significant improvements in mining energy returns have been realized.
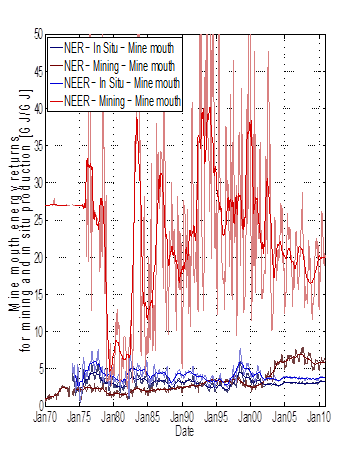
4. What are the implications of oil sands energy sourcing for limitations due to low energy returns?
The net energy returns reported above are just one metric to assess the energy returns from oil sands operations. Using the key provided in Figure 1 for mining operations (top), a mine-mouth NER compares the net output from the mining and upgrading operation to the total energy inputs into the mining and upgrading stage. Symbolically, this can be written as:

where B, k…q are defined for the mining pathway shown in Figure 1. However, this might not be the main point of interest from a societal net energy perspective. If one is concerned about the ability of the oil sands to provide net energy to society relative to the energy that they consume from other sectors, then one may actually more concerned with a ratio that we define as the net external energy return (NEER). Symbolically, we can state that:
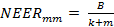
That is, if we are concerned about the ability of the oil sands to provide energy to society, what we are concerned about is parasitic consumption of natural gas (flow k) and electricity (flow m) by the operations, relative to how much unrefined energy they provide to society (flow B). Energy consumed on site that is produced as by-products from the production process (e.g., coke or still gas) may not be a concern from the perspective of energetic availability (although may be a major concern from an environmental perspective).
Measured in this way, the NEER from mining operations was approximately 20 GJ/GJ in 2010, significantly higher than the corresponding NER (see Figure 5).
5. Will low energy returns limit the output of net energy from the oil sands?
These data suggest that oil sands processes exist that have reasonably high energetic returns relative to external energy provided by other energy sectors. That is, relative to the amount of energy that they consumed from the rest of society (e.g., natural gas, imported diesel, and electricity), these processes produce a significant amount of net energy output. This is partly a result of historical development and geographic considerations: the oil sands mining operations developed in a remote and poorly-integrated part of Alberta, and therefore were designed to be largely energy self-sufficient. Importantly, these conclusions are not just limited to mining operations. In situ operations such as the Nexen Long Lake project produce steam using upgrader by-products (asphaltene residues).
Our results suggest that it is not realistic to expect oil sands extraction to be limited by their calls on natural gas and other resources. If natural gas becomes expensive, processes can be adopted to use byproducts of the processing of bitumen to fuel extraction (e.g., integrated operations). However, these integrated processes have implications for the amount of oil sands resource available (e.g., not all barrels able to be produced will be available as “net” barrels of output) and can have important climate implications (e.g., using coke for fueling bitumen separation or steam production is more GHG intensive than using natural gas).
6. Works cited
Peter CJ. Enbridge northern gateway pipeline project energy return on investment. In the matter of NEB file: OF-Fac-Oil-N304- 2010-01 01 Northern Gateway Pipelines Inc. Application for Enbridge northern gateway project, certificate of public convenience and necessity 2011. http://www.ceaa-acee.gc.ca/050/documents/54603/54603E.pdf.
Herweyer M, Gupta A. Unconventional oil: tar sands and shale oil e EROI on the web, part 3 of 6. Technical Report. The Oil Drum, http://www.theoildrum.com/node/3839; 2008.
Rapier R. The energy return of tar sands. 2008. http://robertrapier.wordpress.com/2008/11/14/the-energy-return-of-tar-sa....
Peter C, Jacob N. Alberta to China: what’s the energy return? C.J. Peter Associates Engineering; 2012. http://www.bcsea.org/sites/default/files/webinars/20120522-Peter-Jacob-G...
DOE. Fact sheet: energy efficiency of strategic unconventional resources. DOE Office of Petroleum Reserves e Strategic Unconventional Fuels; 2006. http://fossil.energy.gov/programs/reserves/npr/Energy_Efficiency_Fact_Sh....
Brandt A.R., J. Englander and S. Bharadwaj. The energy efficiency of oil sands extraction: Energy return ratios from 1970 to 2010. Energy. 2013. DOI: 10.1016/j.energy.2013.03.080
Gagnon N, Hall CA, Brinker L. A Preliminary Investigation of Energy Return on Energy Investment for Global Oil and Gas Production. Energies 2009; 2: 490-503.
Guilford MC, Hall CA, O’Connor P, Cleveland CJ. A New Long Term Assessment of Energy Return on Investment (EROI) for U.S. Oil and Gas Discovery and Production.Sustainability 2011;3: 1866-87.
Dale M, Krumdieck S. Bodger P. Net energy yield from production of conventional oil Energy Policy 2011; 39: 7095-7102.
On an earlier post I suggested that barrels of each type liquid fuel be discounted to a 'useful fraction' by multiplying by (e-1)/e where e is EROEI. An EROEI of 4 for example gives a multiplier of 3/4 or 75%. Thus if tar sands got to 4 mbpd with an average EROEI of 4 then 4 X 3/4 = 3 mpbd the useful fraction. Note this metric is abysmal for corn ethanol of EROEI 1.25 the mulitplier being .25/1.25 or 20%.
The energy cliff theory espoused by Euan Mearns and others suggest that major energy sources should have EROEI > 8. That seems plausible to me since primitive hunter gather societies barely generated enough calories to survive. They may have been somewhat contented but they died young; a minor cut for example could be fatal since they lacked broad spectrum antibiotics. EROEI increased with the age of steam and not long after the world economy exploded when conventional oil had an EROEI of 30 or more. My guess is that the kind of world people want with a high protein diet, personal mobility, thermal comfort, affordable medical care and push button entertainment still needs an EROEI of at least 8.
I believe that the implications of such metrics are going to differ significantly depending on whether the energy used in producing an energy source is "internal" or "external".
For example, let's take an offshore rig with no economic means to get gas to market. This happens in offshore Nigeria for example. In these cases, associated gas is generally flared, as any means of getting it to market is so expensive that economic loss would occur. If this associated gas is instead used to power lifting, separations, compression, etc. (which it often is in practice) then what effect does using this "internal" energy have on the net output to society? I would argue that this self consumption of otherwise low value produced product is not consequential to the net energy returns to society.
In contrast: a field that is tied in with the electricity and natural gas grids -- and uses these products to fuel production operations -- can be seen as consuming energy that otherwise would have been able to be used elsewhere in society. This is a more clear case of low EROI having a "parasitic" impact on net availability to society.
With respect to the oil sands, the same thing occurs with mining and upgrading operations. A lot of the energy is provided by coke and still gas, which are otherwise very low value products that never make it to market (stockpiled or flared). Therefore, the energy return ratio if you count all energy inputs (NER) is ~ 6-7, while the ratio if you only count "external" inputs (NEER) is more like ~20-25.
Feel free to send me an email (abrandt "at" stanford "dot" edu) if you would like a copy of the full paper.
In a casual conversation this morning the idea of regretted investment came up which could be another metric. If major river valleys were to be dammed for hydro now deep greens would be hysterical. However we're now grateful for the low carbon power and long plant lifetimes that were instigated by mid 20th century hydro. That investment was 'low regret' compared to say buying the latest military equipment.
On the EROEI 'cliff' there is nothing special about the value 8 as it is not a turning point, just an empirical value. (the usual graph is y = (x-1)/x for 50>x>1, 0.98>y>0) However some aliens from another galaxy might turn up one day and tell us 8 is the lowest value to aim for as the other galaxies didn't make it.
Re gas flaring/onsite powering so long as it is carbon taxed at the CO2 rate if burned, the CH4 rate if vented. Now if carbon tax got really serious it might work out cheaper to inject it back underground which will demolish the previous EROEI.
Alberta heavy industry, including the oil sands, are subject to CO2 regulation which imposes an (effective) carbon tax of 15 $/tonne CO2. They do have to pay such a tax on the fuel gas consumed, as well as any imported natural gas. If they had to reinject the gas, the work required would go up and they would need to get the energy for processing from somwhere else. Not sure that this would make sense.
This misunderstnading often repeats itself.
The average age of hunter gatherers may have been low, but this is mostly due to infant mortality. Hunter gatherers who lived till their 20s had a life expectancy similar to a modern human, and probably died after a short illness rather than a prolonged period of deterioration moderns experience in our hospitals and old people's homes.
How very true. But that's not the only inaccuracy.
Not just primitive hunter gather societies. My grandfather died in 1954 at the age of 96, having reached adulthood when medicine had reached the stage where doctors could make a good estimate of how long a disease or injury would take to kill a patient, but had not yet developed much to cure him, and was well on in his seventies when the first broad spectrum antibiotics were developed.
Also, consider the following quote:
It seems that primitive societies had little difficulty generating calories most of the time.
That last point is reassuring. My nearest supermarket is 40 km away. If there is no black gold to power the car to get store bought calories I'll starve. Then again the shelves may be empty if farmers can't use tractors to grow food and delivery trucks can't get it to the store.
I think it depends on where you are lucky (or unlucky) enough to live. Here in New Zealand some pre contact Maori had a a range of health issues stemming from a restricted diet. Nutrition was mostly adequate but sometimes lacking in protein (New Zealand has no native mammals. Protein would have been entirely from fish, birds, and introduced domestic pigs and dogs). Their teeth were also not in very good shape due to fibrous and gritty staples such as taro, kumaru and fern roots which could lead to malnutrition and exposure to disease. Also note:
"Distilling his careful review of research evidence, historical demographer Ian Pool concluded that, at the time of contact 'birth rates might have been around 38-40 per 1000, death rates 30-35, and life expectation at birth of the order of 28-30 years', with gradual long-term population growth. Although very low by today's standards, this figure matches life expectancies in many European cities and countries at that time"
PDF http://www.waitangi-tribunal.govt.nz/scripts/reports/reports/692/FB92C82...
After a quick search I came upon this paper from Johns Hopkins circa 1990. The dubious sources (like burial counts) are sorted from the fairly sparse better data, but there are still some fairly large assumptions and a wide range for the calculated numbers. However, it is clear that infant and child mortality is a key parameter in life-expectancy.
The Decline of Childhood Mortality
Kenneth Hill
I do not know enough about demographics but model life tables seem a useful tool.
British aristocracy (reasonably documented) provide a case study over several centuries, and the unfavourable effects (a dip in child survival rates) during early urbanisation are discussed. The other largest variable, which appears not to have been discussed adequately in this paper, is Fertility Rate for women. This rate was very high for example in Britain during the 19thC but started its significant drop well before the advent of modern barrier or chemical contraception. Similar falls are noted worldwide today. If we want a more complete picture, I guess some attention is probably needed to be given to the data for survival of women in childbirth.
All the big changes affecting mortality across the age range in the urbanised West and elsewhere followed public health measures and pre-dated the use of effective antibiotics.
FYI, there is demographic information on this here (pdf).
Figure 3 in particular is informative. Modern Western people at age 20 can expect to live about 60 more years, vs. 40-45 years for modern hunter-gatherers, and vs. 20 years for prehistoric hunter-gatherers. (The authors of this paper argue, however, that 20 years is not an appropriate estimate for prehistoric populations, and the figure should be closer to that of modern hunter-gatherers.)
Actually, civilization is a long series of transitions to lower EROEI.
Hunter-gatherers only needed to work for a couple of hours per day - agriculture was much lower EREOEI.
Coal was a big step down from local wood.
Fortunately, wind and solar are much higher EROEI than "newer" oil.
Your observation raises an important point: that EROEI is only comparable within societies of similar population density.
Local wood, hunting, and gathering may have had high EROEI in a world with a population of a few million, but if 7 billion of us try to do it the EROEI will plummet.
Similarly, a thousand years from now we may say "fossil fuels may have had high EROEI for a world with a few billion people, but if all trillion of us try to do it today it won't work."
Yes.
I'm not sure it's useful to think in terms of EROEI falling dramatically when the resource runs out. At a certain point, no amount of work will find additional fruit, or wood.
Probably more helpful to simply introduce a separate metric - scalability. Or, possibly, "maximum net energy".
The evidence that I have encountered does not support this idea. For sources, you might try Vaclav Smil's books, which discuss energetics of historical energy transitions.
The transition from wood to coal in the UK was hugely net energy positive. Early oilfields had EROIs in the 100s to 1000s. For example, the Lakeview Gusher had an EROI of at least 35,000 (calculation I did with Charlie Hall for the Discovery Channel but did not publish yet).
Adam... great work. That is amazing that the Lakeview Gusher had an EROI of 35,000. Here is a question. I just read a new paper from Hall et al about a new EROI study of US Oil.
Looks like the EROI of early exploration was 1,200/1 in the 1920's but has moved down to 5/1 currently. My question is this. Shale Oil in the Bakken was calculated at approximately 5/1 EROI...but that was early on.
With all the new increased drilling, lower production and higher declines in newer wells, has the EROI fallen or has it increased similar to your study here on Tar Sands?
thanks...
The transition from wood to coal in the UK was hugely net energy positive.
Yes, but volume made up for lower returns.
The EROEI of local wood is very high. Coal was necessary because wood was limited. Coal was hard work to dig and transport, and was lethally dirty.
Agriculture was a big step down, but it was scalable where hunting/gathering was not.
I agree that early oil had very high returns - that was an exception to the rule, and I didn't include it.
Local wood perhaps (have not done the math myself). At the point of the coal transition, England's forests were pushed back such that wood became expensive and difficult to transport (over muddy and often impassable roads). Perlin talks about this transition and wood depletion in detail in "A Forest Journey".
Coal was accessible at scale (especially after development of Newcomen Engine to dewater mines) and was transported over water. Therefore it was much cheaper and easier to access (which caused the strange name of "sea coal" to develop). Of course, it also made London an unlivable smelly hell for a few centuries!
Yeah, at the time of the coal transition England had badly depleted their forests centuries before, and were working on depleting the forests of the new world. Wood had a scarcity premium built into the price, and was transported over long distances.
The only sustainable wood is local - many pre-industrial empires were Ponzi schemes that took wood (and food) from a rim that expanded until they collapsed: Athens, Rome, etc. England was saved from that fate by coal - of course, energy dynamics aren't everything: coal couldn't ultimately save the empire...
IIRC, the name "sea coal" predated mining, and came from coal that washed up on the beaches.
many pre-industrial empires were Ponzi schemes that took wood (and food) from a rim that expanded until they collapsed: Athens, Rome, etc
Got a peer-reviewed source for this? It's an attractive idea, I'd like to see the evidence.
As you know, the collapse of the Roman Empire was complex, and is controversial. The "depleting wood and food" theory is a strong competitor, and one I like (though I wouldn't suggest that it was the only element - for instance, one has to explain how the Eastern Empire was able to dial back it's exploitation, and survive another 9 centures).
Off the cuff, I don't remember seeing the collapse of Athens framed quite that way. But, Athens depleted it's wood, began drawing on distant sources, and the need to (and failure to) protect those imports was an important element in it's wars with Sparta.
This has been discussed in a qualitative sense in most of the energy history books, as well as "Collapse of complex societies" by Tainter, "A forest journey" by Perlin, and numerous other places. I don't know how much actual hard empirical data there is on it, but it is reasonably well accepted as a common story with early societies.
I have yet to see the math worked out in a peer-reviewed place, but it would be easy to do with basic calculus and idealized shapes (e.g., expanding circles). Add this to the near-infinite list of papers that I would write if I had the time!
Agreed. That could be the source of the "sea coal" name. I recall that london was fueled with coal from ships (from newcastle south then up the thames) for a long time (centuries?)
Agreed that energy dynamics are not everything.
"The only sustainable wood is local"
That doesn't pass a simple logic test.
The sustainability of wood must be judged by whether the removal of biomass from a forest area is greater or lesser than the growth rate of that forest each year. If the forest is growing (adding more biomass) at a higher rate each year than is being extracted, it can keep going indefinitely and it is sustainable (at least as a resource) no matter where in the world it is located.
However if removals are greater than the growth rate, it doesn't matter if the forest is in your back garden - it is NOT sustainable.
Of course if the wood is harvested then transported it will have a reduced EROI (or IRER!) and if the EROI falls below 1 (or the IRER falls negative), then it would also be fair and logical to deem it unsustainable. It really depends on the mode of transport. Ocean transport is by far the most efficient - in the time you are talking about ships may have been wind powered in which case it wouldn't matter. These days with very large efficient bulk carriers you would have to ship the wood several times around the world to consume as much as energy as you are carrying. At the other end of the scale is road transport where the break-even distance is much smaller (but still well over 1,000 miles if using a large articulated truck).
There are other complexities - for instance how do you set the boundaries of your "forest"? Too small and removals will not be fairly balanced with growth elsewhere, too big and you may be missing localised effects. Of course replanting/regeneration is also essential and in your appraisal you need to take account of your age class - a changing age class is a sign that the forest is changing - this may or may not be sustainable. One of the most interesting features of American commercial forests - especially in the South East states from LA up to VA - is that planting stock is genentically selected to be faster growing and this is a process of continuous improvement, therefore in theory the sustainable removals rate is actually slightly higher than annual growth - although you would never get away with that in practise.
I didn't mention nutrients. Actually, it really depends on the forest and the soil. In the beest cases nutrient removal is tiny if you only take out stem wood and leave behine leaves/needles, small wood and bark. Eventually it would be a constraint though (whether local or not).
Lastly of course I have only discussed resource sustainability here. The issue of sustainability of bio-diversity is incredibly important in relation to forests and is a major constraint for those looking to remain sustainable in a broader sense, but here is not the place to go into those issues.
I agree: being local was not a sufficient condition for sustainability, just a necessary one.
Not necessary, as wood can be transported on bulk ocean vessels at very low energy cost. For instance the fuel consumed in a 4,000 mile journey from the US South to the UK moving wood chips in panamax class wood chip carrier is about 2% of the energy in the wood being transported. The discussion was in refernce to a historical period in which sail ships could have been used - making it even more sustainable.
In modern day, even including some trucking of the wood at both ends, the rate of energy return (and greenhouse gas emissions) is far superior to PV, even for trans-Atlantic wood, but if the forest removals are not sustainable then there is no point (no matter how far it is transported).
Let me clarify - I'm not talking about the current dynamics of wood usage. I'm talking about the historical transition from wood to coal. My point: before the demand for wood exceeded supply, supplies came from local sources, and the E-ROI was very good.
Historically, imported wood was only used because local wood had been exhausted. That's kind of the definition of unsustainable.
A quibble: don't forget to include energy in the form of labor in the calculation of E-ROI for imported wood – sailing ships were *very* labor-intensive. As a practical matter, I believe the British imported wood (before the advent of coal) not primarily for fuel, but for applications where wood was essential: building warships (especially sailing masts, for which domestic logs just wouldn't suffice), charcoal for iron smelting, etc.
Another proof by example is ethanol production, which arguably has an EROI < 1.
The key point regarding tar sands and EROEI vs ROI (in $) is that the natural gas used for much of the process can be said to be "stranded" for other usage, no ?
For mining operations, much of the energy used in the process is in the form of upgrader byproducts, including still gas and coke. These products have little economic value, due to poor quality and remote location. Therefore, if they are not consumed in the upgraders, they are generally "wasted" by stockpiling (in the case of coke) or flaring (in the gas of gas). This will have a big impact on any ROI vs EROI comparison.
The natural gas used in the operations should likely be considered fungible with other gas in Alberta.
The natural gas in the oil sands area is not really "stranded", but it is expensive to transport to far-away markets in Eastern Canada and the US. With the current glut of domestically produced shale gas in the US and resulting low natural gas prices, gas from Northern Alberta and BC (particularly Canadian shale gas) is uneconomic to deliver to those Eastern and US markets. Selling it to the local oil sands plants is the gas producer's best option.
Under Alberta's strict flaring regulations, burning the process gas would not be an option, but it is a perfectly adequate fuel for the upgraders so almost all of it goes for fuel. Coke is burned for fuel as well.
If there was a local market for the coke - e.g. steel mills or power plants in Northern Alberta - it might be more salable, but there are no steel mills around and the power plants are mostly mine-mouth units sitting on top of big deposits of low-sulfur coal. They are not much interested in high-sulfur coke. If it was closer to China there would be a huge market for the coke.
Oil sands coke has found an interesting market recently. A big heavy oil refinery near Detroit has been processing bitumen and building up huge piles of coke on the water's edge. However, Nova Scotia Power has started buying it and burning it in a power plant in Nova Scotia. The power plant was built to burn Nova Scotia's local high-sulfur coal, but the coal mines have shut down as uneconomic, and the cheapest alternative is coke produced in Michigan from Alberta bitumen. Too bad they can't get the bitumen to Nova Scotia to reduce the oil costs.
http://www.desmogblog.com/2013/05/21/greenwashing-tar-sands-part-3-where...
This says that if EROEI is 20 for crude, tar sands has an EROEI of from 5 to 7.
Only rough congruence exists between GHGs and EROI. We are using this same dataset to build a time-varying GHG analysis. This is forthcoming (in review). Our detailed energy consumption dataset is a much more rigorous way of getting at EROI (or whatever metric you are interested in) than a GHG comparison (which can be stymied by shifts in fuel shares from coke to still gas, etc.)
Agreed. There is certainly interest in coke if the price is right. Power generation is what will happen if raw bitumen is sent to a less remote market (as dilbit or synbit) and coking occurs in an accessible area. The Detroit case is of interest, and has made the papers. If refining occurs on the gulf coast, there will be ready markets for the coke.
I believe that what most people who are concerned about EROI are actually concerned about is something more like the NEER. It has little impact on energy availability if some fraction of the bbl is consumed as coke or still gas, except possible reductions in net resource availability from a truly enormous resources base.
Tanks a lot for the info, RMG and arbrandt
Hi, Adam.
I am wondering about one of your result. From a simple back of the envelop EIO-LCA analysis, and EROI of 20 in the Oil industry, should translate in a exploitation cost around 30-50 $/bbl. However, there is indication that the exploitation cost are more around 70-80$ $/bbl. This would mean that the NEER is closer to 10. Nevertheless, I have used rough societal average. It might be possible that in a remoter region la northern Alberta higher EROI are needed to be sustainable, like for an off shore platform.
Adam Brandt here. The translation between dollars and energy is challenging, and will depend on the nature of the operation. For example, some operations are more capital intensive (e.g., mines and upgrading) while others are more energy intensive (e.g., in situ projects). These data are direct energy use, as reported to regulators, and so should be more accurate than an EIO-based number.
An interesting thing with the oil sands is that a significant amount of the energy consumed is not "commercial" energy that otherwise has much market value. For example, the integrated mining and upgrading operations use a lot of by-product coke and still gas (upgrader gas) that has little other economic value due to location and quality.
Adam, actually people in Calgary have done all those calculation, they had a supporting grant for the oil industry. However, once the oilers found that the results were not moving in the right direction, they just cut off the funding ;) I tried to find out for over a year before giving up.
The operating costs of the existing oil sands plants actually is around $30-$50/bbl. It is the costs of NEW oil sands production which is more like $70-$80/bbl. The difference is the cost of labor and capital to build the plants which are the biggest factors in new oil sands development.
The primary factors of production in traditional economic theory are Land, Labor, and Capital, while Energy is only a secondary factor of production. This is true of oil sands development today. The big constraints in Northern Alberta are the shortages of Labor and Capital. Availability of Energy is not a significant constraint in Northern Alberta - there is as much Energy available there as is needed.
This perfectly illustrates the difference between the marginal cost of production and the average cost of production.
While it might not make sense to add more oil sands capacity if the price drops below 70-80 $/bbl, producers will keep operating well below that price. On the margin, they can make money at quite low prices (especially if the gas prices is low at the same time).
The existing oil sands plants can get their marginal cost of production very low if they have to. During the low point in world oil prices around 2000, they managed to reduce their avoidable costs to around $12/bbl. They did this by cutting staff, putting new development on hold, and generally operating with very sharp pencils. The cost of fuel gas was also very low because gas prices tend to follow oil prices.
The owners of these plants have very deep pockets and are not going to shut them down even if they are losing money. The reason is, if they shut down the operation, they would have to surrender their leases to the government, the government would re-lease them to someone else, and when prices went back up they wouldn't be able to get back in the business because all the good land was leased by someone else.
They know the conventional oil is running out and the oil sands are the last big reserves left they have access to, so smart players don't want to give them up. Dumb players? Well I won't name names, but I know of one giant multinational which sold its leases back when oil prices were low, and then ended up buying back the same leases for billion$$$ more than they sold them for after prices went up in recent years.
Conventional oil involves a lot of risk, and the risk offsets the nominally better rates of return. There is no geological risk in the oil sands, only the economic risk of prices being too low. Example: Shell's recent Arctic offshore drilling fiasco, which discovered nothing and ended with them running the drilling rig onto the rocks and damaging it, with full press coverage. Meanwhile, Shell's oil sands plant kept humming away generating money - not as much money as a big Arctic offshore discovery would make, but certainly much better than having an Arctic offshore disaster.
Am I right that there is no time element in EROI calculations? I have never been able to understand this, why should EROI be any different from financial IRR in its fundamental methodology? I'm sure I must be missing something, because this seems so obvious. Please don't feel patronised by this point, I'm sure it is down to my ignorance, but I would appreciate it if someone could comment as I've so often wondered about it.
Take the following two examples, the first is an oversimplified oil sands operation and the second is an oversimplified solar PV plant, both have an EROI (as I understand it) of 2 GJ/GJ.
Example 1: Oil sands:
On day 1 the oil sands producer is forced to expend 100 units of energy (which he has "borrowed" from society), the process is not at all capital intensive (negligibly so in this case) but requires 100 units of diesel fuel to dig up, wash and refine the sands back into diesel (in this theoretical example). At the end of the day he makes 200 units of energy. On day 2, he has to start all over again and invest his 100 units of energy, again he gets back 200 units at the end of the day. And so on. The process has an EROI of 2 GJ/GJ. However at the end of the 1st day, the oil sands producer is a able to completely pay back his "loan" of energy from society and still have enough left over to operate again the next day. At this point, as far as society is concerned they are even and from then on he produces a surplus energy amount of 100 units every day. So even though the EROI is very low, as far as society is concerned the INITIAL energy investment was paid back in a single day and from then on it receives 100 units every day without any further investment.
Example 2: Solar PV:
A solar PV developer invests 1 thousand energy units in the form of electricity (which again it "borrows" from society) to make the high grade crystalline silicon, turn it into photovoltaic cells and install them for electricity production. The operating life of the PV plant is 50 years and it takes very little energy to maintain the plant (negligible in this case). It produces 40 units of energy per year for 50 years, overall producing 2 thousand units of energy, an EROI of 2 GJ/GJ, the same as the oil sands operation. In this case however, society has had to wait 25 years before it gets paid back the energy it invested! After that, as with the oil sands case it gets surplus energy every day.
Both cases have an EROI of 2 GJ/GJ but the difference in impact on society of the 2 options is huge. In the first case society gets surplus energy almost straight away - after 1 day, in the second case it takes 25 years to make any surplus energy during which time that energy debt could have been spent on something else. This difference between upfront capital investment and on-going operational expenditure, and the different results in terms of payback time and return on investment is obvious to anyone who has ever done a day's corporate level work in their lives. It is crucial in making financial investment decisions, again for completely obvious reasons, and in the two examples above, the time difference would be just as meaningful to an energy constrained society. But as far as I can understand there is no time component whatsoever in EROI, so how can it be a useful measure of net energy production?
Can this possibly be right? Is my understanding of EROI wrong? If not - I am sure this must have been debated and discussed before, what is the rational for it? I can see no possible justification, what am I missing?
The 2:1 return from oil sands presumably is over the lifetime production, so just as with PV you don't get it back the first day. And it does not consider that the return from the oil sands depletes the reserve while the return from the PV uses renewable fuel.
The difference is made possible by the indiscriminate use of the term EROEI. Replace the oil sands by a fuel tank with a spigot. No matter how efficient the pump, the EROEI from a fuel tank is always less than 1 if you consider the potential energy in the tank as one of the energy inputs. But the PV case generates additional energy that would not have been available otherwise, so it can truly have a positive EROEI.
However if you simply regard the oil sands net output as energy that would not otherwise be available to society, it wins if you can extract it more rapidly than using PV.
The numbers for cash flow are not all that different; the oil sands tie up capital during the build up of infrastructure, but the PV can return a positive cash flow in the first month of operation. Apply your own discount rate before investing!
This is an interesting problem. Michael Dale at Stanford University has started to look at returns as a function of time, rather than integrated over the whole project life. He has a recent publication that looks at rates of energy return over different times of project life.
Also, energy returns of 2 GJ/GJ are too low for both oil sands and solar. Given what we have seen for oil sands operations, the industry as a whole is closer to 5-7 GJ/GJ. Most recent solar analyses are closer to 5-10 GJ/GJ, rather than 2 GJ/GJ.
Yes, I agree. Energy payback time is more important that EROI for long lifetime project due to the non stationary of the system boundaries. I have a paper waiting for an internal review before submission, that as an extensive discussion on this topic. This aspect is very important in sustainable building practices.
Sustainable building would have huge effects. Lots of embodied energy and time effects.
You are right Adam. The problem is that promoter of sustainable building have a tendency to support the most extreme measure to save a kWh even if this makes no sense.
Perhaps energy has a Net Present Value curve which is sloped in the opposite direction from an NPV curve for money. A dollar now is more valuable than a dollar 5 years from now, but with respect to energy, as efficiency increases one can argue that the "True Value Curve" (just made that up) is actually upsloping. A gallon of gas would have allowed you to travel 15 miles 10 years ago, now that same gallon allows you to travel 25 miles (not actual numbers of course). Although the quantity of energy is the same now and 10 years ago the utility derived from that gallon has increased.
So in a way applying anything resembling a (financial) NPV type of analysis to physical energy, not the dollars associated with lead you to make the opposite decisions of what ought to be done.
abrandt: thanks for sending me the paper. It seems quite well researched and within the boundaries of energy efficiency analysis of bitumen production a welcome and needed contribution.
Rgds
WP
An interesting concept. I think that Mik Dale at Stanford might have looked at these sorts of issues, so you might contact him.
I would wonder if such a curve would slope differently than a financial NPV curve. NPV and discounting arises because of human "impatience". A trip to Hawaii is more desirable this summer than next summer (weather in terms of monetary or energetic costs and benefits) because I may be unable to travel next summer. I wonder if this same impatience applies, and acts to offset the increased efficiency effect that you are discussing.
There is indeed some depreciation due to energy basket change, efficiency improvement. In building, climate change has also to be factored as the unknown lifetime of a building.
This article is useful in that it brings out the fact that the energy return on oil sands is a lot higher than the 2:1 or 3:1 ratio that is commonly bandied around. Those are based on old data. There has been a lot of research and development done to bring the efficiency of the oil sands plants up, and the ratio is over 5:1 today and still rising. This renders a lot of the skepticism obsolete - these plants really do work to generate more energy.
The distinction between internal and external energy consumption is also useful, because if energy cannot be used, it doesn't matter how much of it there is. If it is used to produce more energy, then it is the external consumption and production that matters, not the internal processes.
One point not brought out is that the oil sands plants are burning a low-cost form of energy which is available in excess supply (natural gas) and in effect converting it into a higher-cost form of energy which is much less readily available (oil). If you did this with a gas-to-liquids plant, you would have an EROEI of about 0.6:1, but it still might be cost-effective. If you do it with an oil sands plant, you achieve an EROEI of 5+:1, which is much better.
The economics of it are better than the energy balance. If you take 1 GJ of gas, which costs about $3 at an oil sands plant, and use it to produce 1 barrel of syncrude, which is worth $100, you have achieved a ROI of about 33:1. Of course it's not nearly that simple because it ignores the very important costs of labor and capital, but it does illustrate that you are making a significant gain in value.
One way to view internal consumption is by changing the system boundaries. If you draw your boundaries to envelop the internal consumption, you can assess the net inputs and outputs to society (e.g., you "black box" the process and disregard internal flows). This, to my mind, gives a better sense of the scarcity implications of energy returns. However, total energy consumption (e.g., NER) will be more proportional to environmental impact.
We discuss these mathematical aspects in a few recent papers:
Brandt, A.R., M. Dale, C. Barnhart. (2013) Systems-scale energy efficiency with multiple energy pathways and non-energy processes: a bottom-up matrix-based approach. In review, Energy.
Brandt, A.R., M. Dale. A general mathematical framework for systems-scale efficiency of energy extraction and conversion: Energy return on investment (EROI) and other energy return ratios. Energies 2011(4): 1211-1245. DOI:10.3390/en408121
Thanks, RMG, for a very clear summary of this whole discussion, but I worry about what is being done ...
I wonder if a case can be made that any resource that is now in excess supply may some day become more in demand. For example, as global warming progresses, human population will likely migrate toward polar regions, and these future generations of Canadians may rue their forefathers decision to strip the region of its once abundant energy resources.
Thomas Jefferson once opined that the Earth and its wealth belongs to the living, unfettered by concerns of past or future generations. When I first read it, I was impressed at his wisdom, but now not so much. There is. also, a Psalm about being good stewards of the Earth, which is hardly what is happening in the case of so called 'stranded' resources.
I don't accuse you of advocating what some might see as vandalism. But your summary did clarify my thinking.
It's highly likely that fossil hydrocarbons will be used less and less for fuel. Even petrochemical feedstock will likely be phased out in favor of pulling carbon from air and water, and hydrogen from water.
Renewables will get cheaper and cheaper, and FF will eventually be seen as a very, very odd, very very dirty anachronism.
I can't really believe in your vision of the future. The laws of physics, energy conservation, etc. will still apply, IMHO.
Of course. Wind and solar power obey all of the laws of physics, energy conservation, etc. For instance, Conservation of Energy is obeyed: the Earth is an open system with 100,000 terawatts of continuous input (and not quite as much output, lately!).
Which laws were you worried about?
Well, if human beings ever decide to settle on Melville Island, in the Canadian Archipelago, they can exploit its oil sands, which rival the three oil sands in Alberta (Athabasca, Peace River, and Cold Lake). Few people even know about the Melville Island oil sands, even fewer care, and nobody lives there despite the fact it's slightly bigger than Switzerland. It also has huge reserves of stranded natural gas, and not inconsiderable reserves of conventional crude oil. There are also huge amounts of coal in the Arctic Islands. It's just all frozen in place.
I don't think future generations of Canadians will really remember the historical oil deposits of Southern Canada once they are gone, any more than they remember the huge white pine trees of Toronto and Southern Ontario or the once vast rain forests of Vancouver and the Lower Mainland of BC - or even the dense, dark, trackless oak forests of Southern England. They are gone now, and the people living there now don't remember they were there. Who even thinks about the ferocious wolves, lions, and bears that stalked the ancient English forests, now that they are seen only on heraldic crests?
Future generations will look at the hydroelectric dams and wind farms and think they always were there. In fact they will probably be nostalgic about them when they are replaced by quantum zero-point diffusers or some other Star-Trek-like energy source.
Nicely put.
I'm really amused by people being nostalgic about Dutch and Spanish windmills, while others are complaining about modern windmills...
"Am I right that there is no time element in EROI calculations?"
I have mentioned this before as a weakness of EROEI; that without a time element one can't really say that you can't run society on a low EROEI. If I returned 1.5 units for every 1 unit I put in -- but it returns that every day -- then that would be a fantastic process. The other weakness is the issue of unlike energy inputs. It may make perfect economic sense to turn 2 BTUs of coal into 1 BTU of liquid fuel -- even though the EROEI is less than 1. What the EROEI tells you in this case is that this process would accelerated depletion of fossil fuels.
Many thanks for your time and replies, especially Professor Brandt.
As I thought, I am hardly the first to have thought about the importance of timing in energy investment returns. It's also good to know that there are people doing formal work on the subject.
On the other hand, I was afraid of the answers that were given, and I find this very disturbing. For years I have read blogs and articles about EROI and been troubled by the thought that time effects were being ignored - rendering the value of the discussion (i.e. as a metric for the usefulness to society of an energy source) almost worthless - but I've always sort of assumed that I was missing something somewhere, since lots of obviously intelligent people don't usually sit around wasting their time. What I think I'm reading is that actually I'm not missing anything at all and that EROI is indeed oblivious to time.
The analogy with financial investing is quite obvious. We have been talking about unit margins while all the time acting like (or at least giving the impression to me, and therefore surely others) we have been talking about project level IRRs.
I've spent my career in UK power and more than half of it as a project developer, of course unit margins are interesting but the first and only metric of importance that an investor wants to know is IRR. Later he might look at unit margins in order to better understand the mechanics of the business but the investment decision is going to be taken based on IRR. That is also clearly what society needs to do when evaluating the net energy returns of an energy project, it needs to know at what rate (rate = amount / time) any energy that it has to invest will be returned to it (along with the additional energy profits) so that it can go on being used to human ends.
Please somebody help me. What is the reason for unit margin EROIs being the main metric of discussion and not Internal Rate of Return of Energy?
P.S. Final point: Sorry to those who thought I was saying that oil sands (and/or PV) EROI is 2. I was just trying to make a point about the timing of energy returns and used the number 2 for simplicity of arithmetic. It was supposed to be purely illustrative - sorry for the confusion.
Hoover, You have conveniently forgotten that the time constant involved in fossil fuel EROEI is several hundred thousand years. That is the amount of time it takes to transform ancient biota into a convenient energy store. How do you like them apples?
Very droll, but not very constructive.
Or have I forgotten some reason why EROI/IRER doesn't matter for renewable (or nuclear) energy?
In fact I seem to remember reading blogs on TOD about an energy "hump" (or was it bump?) referring to the difficulty of transitioning to renewable energy because of the sheer amount of upfront energy investment required. A bizarrely qualitative and inelegant approach to describing the issue of payback times and rates of return that is so much more easily captured and quantified by using an internal rate of energy return calculation - but at least one that recognises that the challenges for society of IRER are probably greatest of all for renewable energy.
The time scales for formation of fossil fuels from biomass material would not be material to any calculation of IRR, EROI, etc. Fossil fuels have essentially been "gifted" to us by geologic processes, and the time scales involved are so long as to be effectively infinite.
EROI can be whatever you want it to be.
Take your oil sands example, run it for 100 days.
Output is clearly 99 GJ higher than input, but there's 100 GJ that can either be ignored as an internal recycle, or counted as both output and input.
Counting it as internal recycle EROI = 100/1 = 100
Counting it as output and input EROI = 200/101 = 2
The analysis can be interesting, but the EROI result is useless once divorced from the analysis that led to it because it is completely dependent on hidden assumptions made in drawing the boundary. Is the process with the EROI of 100 fifty times better than the process with the EROI of 2, or is the boundary just drawn in a different place? Unless you see the detail of the analysis, you don't know.
a post on eroei boundary issues (a second one is coming)
http://connectrandomdots.blogspot.com/2012/07/test-post.html
Rgds
WeekendPeak
I'm utterly confused by that. You could apply exactly the same thinking to finance and investment - you could, but why on earth would you? And indeed no one does.
There are only really a discreet number of variables that are worth considering, the science and mathematics of this stuff was long ago created and refined to dreadful detail by accountants and economists. That's why I can't understand why people don't just apply the same thinking to energy.
Ultimately the one that matters the most is the one that must be considered before embarking on any investment (whether of finance or energy) which is the rate of return over the lifetime of the project.
Hi Hoover,
Very interesting discussion. It's pretty obvious that investments today in Solar, Wind farms or Oil rigs are done by investors who look at IRR. But is it the right measure to talk about society's future?
My take would be that EROI's avoidance of the time parameter is also a strength. It leaves out of the picture the messy details. In other words: EROI lets you take a birds eye view, and IRR gives you more details (together with many more dubious assumptions and errors). Take MIRR, with cost of capital included. It looks good on the books, doesn't it? Now forward to 2045, increase global temperature 2 degrees, reduce global oil production by 40%, assume a deep financial crisis with 20% interest rates, demand falling, war ... did you predict the cashflow in your IRR calculation? Probably not.
What I have seen in the industry are business as usual assumptions: growth, constant inflation, average interest rates, same demand, typical risk premiums (construction delay, contract problems, maintenance problems). But reality in 2033 might look a bit different. From that point of view, talking about 2040 in terms of IRR is probably pure fantasy. And EROI might be a more cautious measure to guide us.
There is another question, and it's the definition of "useful to society". Is it better to give society 100GJ/day to burn today? Or is it better to give a small stream of 2GJ/day and buy time to figure out a more sustainable future for Humanity? The question has been phrased in a different way by asking: ... if we suddenly had cheap unlimited fusion ... would it help us as a society ... or would it accelerate our destruction?
I think those two factors: 1. Defining useful, and 2. getting too detailed may have something to do with the use of EROI. If you're investing your money in a well defined 10 year project ... definitely IRR. If you're deciding Humanity's fate for the next 50 years ... then it's not so clear which metric is best to guide you.
Thanks for your reply Massagran.
I agree with you completely that there are a wide number of important factors that need to be taken into account when deciding whether or not an energy source is good for society. Clearly the impact on the environment, climate and long term resource depletion are all factors. BUT we should not try to confuse all those things into one metric; we should test them each independently. The energy return on investment metric helps us to decide if, in developing a primary energy source, we are actually producing enough energy for society’s use to justify the energy that will have to be invested. This is especially relevant when subsidies are involved (i.e. almost everything). It shouldn't be confused with resource depletion or social/climate/environment issues, rather it is a test that should stand alone and alongside those other tests.
I'm going to risk suggesting another example now, one that will probably be even more controversial than the oil sands, and it is corn ethanol. Corn ethanol is usually cited as having EROI in the range of 1.1 to 1.3, which is widely condemned by energy pundits as conclusive evidence that at best corn ethanol is a waste of time and money. However ON ENERGY RETURN GROUNDS (but not environmental or social) this needs to be challenged.
Firstly there is the fact that much of the energy input is natural gas and most of the output is transport fuel. Others have just pointed out the difficulties in assessing the relative "value" of different types of energy, this is clearly a difficult problem as not all energy carriers are equal (as a power station developer I have been involved in many energy projects, but never one with an EROI of more than 0.7!). But I want to deliberately ignore that for now and just purely focus on whether or not we are right to judge corn ethanol as useless by its low EROI.
Corn ethanol is an annual crop and the vast majority of the energy needed to produce it is spent in the same year as the ethanol is consumed. Let's assume an EROI of 1.2 for the sake of argument (PLEASE note, this figure is purely illustrative - I have no axe to grind on what the correct number is). The EROI does indeed look terrible, but because the returns are annual, in 5 years enough surplus energy produced by corn ethanol has been generated to offset the energy that will have to be spent the next year to make more. At this point "society" has already broken even, from then on every year the surplus 20% is effectively provided scot free to society. The Internal Rate of Energy Return is 20%. Resurrecting my earlier 50 year PV project with the EROI of 2 (far better than the corn ethanol) the IRER on that is only 3% because all of the investment is up front and takes a long time to pay back.
How is all of this relevant to your comments Massagran? Well firstly, corn ethanol is a great example of where those other social considerations should come into play. While I disagree that corn ethanol should be ruled out on energy grounds, I am still personally against subsidising it because I feel the environmental and social costs are too high, something I judge by applying tests that are completely independent to energy returns.
But now to answer your question about timing; is it better to produce energy now than later? For two reasons the answer is a conditional, but resounding yes! Conditional on other environmental and social factors. Energy is used to improve people's quality of life. Honestly, my quality of life is already so great that any marginal increase in my use of energy only has a small additional benefit to me. In fact I actively look for ways to consume less energy. However I am part of the lucky 10 or 20% or so of the global population for whom that might be somewhat true. For everybody else access to more (cheaper) energy would improve their lives quite meaningfully and I passionately see that as goal in itself.
How does the rate of energy production affect this? Well I think the easiest way to visualise the answer is to consider our ability to re-invest the energy "profits" to produce yet more energy for society in the future. Let's take another example:
Say "society" happens to have a spare 100 energy units that are not currently required for essential human needs. In this example it (society) already has an excellent and steady supply of energy for the next 50 years but that it is known that this source will dry up suddenly in 50 years’ time. The President comes to you and says this: "Please take these 100 energy units and invest them. Whatever energy you produce you must re-invest for the next 50 years, but on the 50th year, and every year from then on, you must produce as much NET energy for society as you possibly can, sustainably, for ever".
If we invest in the solar PV scheme with the EROI of 2, and each year use the electricity produced to keep expanding the array, then on the 50th year, when society's original energy source finally dries up, it works out that on a sustainable basis we are able to supply roughly 13.5 units per year of energy to society indefinitely (i.e. net of the energy that will have to be constantly re-invested to replace expiring cells - a different amount each year on a 50 year cycle meaning some will have to be stored through the cycle).
However If instead we had invested in corn ethanol with the pitiful EROI of 1.2, after 50 years we would be supplying a massive 151,674 units each year on a sustainable basis – 4 orders of magnitude more! Roughly 910k units would be produced each year and roughly 758k would have to be held over to invest in the next year’s production. Thus we can see that society was far better off by investing in the technology with the highest RATE of return even though it had a much lower UNIT return.
Finally, I beg my fellow commenters to understand and recognise the limitations of this model; it is for illustration purposes only. I fully realise that in the real world I would have had to consider whether there was enough agricultural land available for the corn crops; or if high food prices might have created appalling poverty; or if silicon for the PV or potash for corn fertiliser might have run out; or if terrible environmental damage caused by intensive agriculture might have been worse for society than a lack of energy. These factors MUST, of course, be taken into account, and in many/most/all cases over-ride the benefit of higher energy returns. But that does not mean energy returns should not also be properly tested and understood - they should! And the correct metric for proper evaluation is the internal RATE of energy return (IRER), NOT the marginal expenditure per unit of return (EROI).
Sorry for the long one, I feel strongly.
Sure, society gets more energy back at 1:1.2 per year compared to 1:2 over 10 years. And in your example the energy is extracted from the Sun by way of photosynthesis, so all other things being equal it is as sustainable as PV.
However the theoretical maximum efficiency of photosynthesis is 11% and in practice half that (sugar cane can do 8% in a good year). So the hectares used in energy production could be generating twice the amount if the crop were replaced by garden-variety 15% efficient PV. Production would ramp up more slowly as the area fills with panels but in the long term the highest EROEI process will extract the most energy from the sunlight. Lifecycle EROEI analysis is essential to make that decision; the initial rate of energy return is a consideration only during the ramping up phase.
Hi dak664, that is a very useful argument.
You have thrown an additional variable into the mix - that of land availability. Your conclusion is that, because land is constrained, PV is a better investment than corn ethanol, despite its much lower IRER, because in the end more energy will be extracted from the constrained resource (land in this case). You may very well be right, in fact I'm sure you are, but you are naughty because you are subverting my argument away from the pure comparison between RATE of energy return and UNIT energy return (IRER v EROI). I deliberately went out of my way to exclude additional variables such as resource constraints, demanding instead that they be tested and considered separately.
If we once more exclude land availability as a variable then the reason why corn ethanol is superior in terms of RATE of energy returns becomes clear. PV - although it overall requires less energy investment per unit of energy eventually produced - requires ALL of that energy to be invested UP FRONT - the production of crystalline silicon is a tremendously energy intensive thing, and so is the energy required to install the module relative to the electricity it produces. Once it's installed it sits there producing electricity all by itself (for 50 years in my illustration) without any further inputs. The planting of corn on the other hand is not as energy intensive as crystalline silicon production, but unlike PV it has to be re-invested every year. Yes, there is a lot of energy embedded in the nitrogen fertiliser, the harvesting, transport and distillation - however that energy is all paid back in the same year with profits.
As before I want to emphasise that real world constraints have to be properly considered when evaluating energy investments - environmental, social and resource scarcity constraints. In your case where land is the greatest constraint then yes, that would over-ride the IRER comparison (although I don't know if land for solar PV really is constrained). However, this does not mean that energy returns should not also be properly evaluated by considering the relative RATE of energy returns (not EROI).
Ethanol production from sugarcane is hoped to increase to 9,000l/ha, or somewhat under 1,000 gal/acre. Each gallon of ethanol has about 22kWh of energy, so each acre is expected to produce about 22,000 kWh/yr, or 5.75kWh/m^2/year.
By contrast, a square meter of solar PV will produce approximately 0.75 kWh/day, or nearly 50 times as much energy.
(For comparison, corn produces about 400 gallons per acre, or 40% as much as sugarcane, meaning it produces 0.8% as much energy per acre as solar PV.)
I appreciate that these numbers are chosen to illustrate your point, but it's worth noting that your input numbers are grossly unrealistic, so the example you discuss is unfortunately highly misleading.
The EROEI of solar PV is 6-12 (and increasing) for a 30-year lifespan. Using the minimum value of 6, we find that after 5 years, when ethanol has generated enough surplus energy to pay for one year's production, solar PV has generated enough energy to entirely pay back its construction cost up to twice over.
Sorry if I've come over negative about PV, that really isn't the case. I did my Master’s thesis on thin film PV (back contact effects of CdTe) and I wanted a career in PV at the time - there were simply no jobs in those days and I needed a reasonable income due to some hefty debts. I ended up going in a slightly different direction but still in power and mostly renewables.
I'm in awe of the progress made by PV recently, even if I suspect some of the cost reductions are not sustainable (i.e. from Chinese production subsidies). I'm sure they will be replaced by other more fundamental cost improvements, and it seems inevitable to me now that grid parity will happen, with or without Chinese dumping, and some places are probably there already.
For me, I just can't compute the lack of understanding about the importance of rates of return. PV does suffer relative to other renewables technologies when considering rates of returns instead of unit returns, albeit clearly nowhere near as much as in my simple example. It doesn't mean that PV cannot or should not be built out, but it is an important consideration because it means the faster we try to build it out, the more scarce energy for investment will become which will limit the rate of deployment relative to other technologies.
In reality other constraints are more important than energy returns for all the technologies - load profile is the biggest one for PV, wind sites for wind (and grid flexibility), appropriate land for biomass etc etc. But anyway, while we're talking about energy returns, we may as well use the right metric, otherwise the conversation becomes irrelevant.
otherwise the conversation becomes irrelevant.
Really, this conversation has already become irrelevant. Both wind and solar have E-ROI and E-IRR which are "good enough". Those metrics don't really matter any more for those two sources. And, I think Liquid Fuel Return on Liquid Fuel Invested is more important for an analysis of ethanol.
Cost is more important - both out of pocket and total (which includes externalities like CO2, other pollution, and energy security). That still puts wind well ahead of solar.
Of course, other things matter too: solar is on the consumer side, it provides power that is peak or close-to-peak, etc.
If the E-IRR of PV really is already around 40% now (or even half of that) then, yes, I have to agree with you. Growth is exponential (although limits are not) so that becomes a huge number in no time.
Oil sands and corn ethanol, which have poor EROIs, have pretty reasonable E-IRRs.
Thanks for the conversation. The conclusion is that it has been a waste of time .... but of course it hasn't been.
I'll turn my thoughts now to limiting factors and real terms delivery. My instincts about intercontinental transmission lines are that there will be far more optimal solutions than that, unless superconductors jump out of the box. Hmm, interesting.
"EROI can be whatever you want it to be."
This is a major challenge with the term "EROI" or "EROEI". It is used in such widely varying ways as to be very difficult to compare. I have argued mathematically that this is the case in two papers. Feel free to write to ask for either paper:
Brandt, A.R., M. Dale, C. Barnhart. Systems-scale energy efficiency with multiple energy pathways and non-energy processes: a bottom-up matrix-based approach. In review, Energy.
Brandt, A.R., M. Dale. A general mathematical framework for systems-scale efficiency of energy extraction and conversion: Energy return on investment (EROI) and other energy return ratios. Energies 2011(4): 1211-1245. DOI:10.3390/en408121
For this reason, I don't compute "EROI" per se, and in this oil sands paper compute 8 metrics of interest, which have different boundaries. People have been looking at and comparing different metrics for computing energy returns since at least 1976. We go over the history in the earlier (2011) paper. Unfortunately, many people who do EROI analysis are not careful with defining boundaries or being clear about exactly which metric is being computed. This area is in dire need of rigor and standardization of terminology. Baring that, much more transparency is needed in system boundary definitions.
+1 for standardization of terminology, with the first distinction being that between the energy returned from a fixed reservoir which caps the total amount no matter how fast it is extracted, and the energy extracted from an entropy stream or renewing reservoir, with no upper limit to total but limited in rate (wind, hydropower, PV, Dyson sphere)
Reliance on the former would explain the Fermi paradox, but we can fondly hope that happy civilizations enclosed in Dyson spheres are just hard to detect.
+1 for referencing the Dyson sphere in a post about oil sands! ;)
Where can I find a description of the 8 metrics and their relative importance?
Briefly in this paper, but more thoroughly in my mathematical papers:
Brandt, A.R., M. Dale, C. Barnhart. (2013) Systems-scale energy efficiency with multiple energy pathways and non-energy processes: a bottom-up matrix-based approach. In review, Energy.
Brandt, A.R., M. Dale. A general mathematical framework for systems-scale efficiency of energy extraction and conversion: Energy return on investment (EROI) and other energy return ratios. Energies 2011(4): 1211-1245. DOI:10.3390/en408121
Feel free to write for any of them: abrandt "at" stanford "dot" edu
Hey hey Hoover,
There was a TOD post on this a few years ago: http://www.theoildrum.com/node/7147
I haven't read all the replies to your post. It's late and I have to work in the morning so I apologize if any of this has already been addressed.
There are at least three good reasons why we use time in financial IRR. 1) Interest rates, both charged and received, that represent the opportunity costs in an exponentially growing economy. 2) Discount rates from human psychology. We discount the future in a hyperbolic, not exponential, way and there are evolutionary reasons for this. 3) Entropy and or physical wear. There are stock flow issues that need to be addressed for any dynamic system with built infrastructure. Biomass of mammal populations (humans), leaves on trees, business equipment, roads and grids, etc.
I don't know how to segue into this but there was an economist named Pierro Sraffa who wrote a paper called 'the production of commodities by means of commodities' that sparked a big debate in economics called the Cambridge Capital Controversy (University of Cambridge in England vs MIT in Cambridge Massachusetts). The gist of it (for our time quandary) was that there is a trade off between capital and labor that should be governed by interest rates in one, and only one, direction. Sraffa didn't like this at all and he provided a counter example where the production of some good would switch from one capital to labor ratio/technology to another (which is supposed to happen) as interest rates increased and then switch back (which is not supposed to happen) as interest rates increased further.
The Cambridge Capital Controversy died a quiet death. It posed a challenge that neoclassical economics could not answer (which is a bad thing for the neoclassical school of thought) but it failed to advance the field (which is a bad thing for economics at large). Sraffa didn't know diddly about energetics. He was only concerned about economic theory and so he missed the stock/flow angle. The relationship between stocks and flows is time. The stock of fossil fuels that we have was a long time in the making and the flow from renewables could replenish those stocks over sufficient timescales.
As I said, it's late and I have to work in the morning, so I am stopping here. But, applying time to EROI or applying energetics to the financial IRR is going to offer insight.
Thanks,
Tim
Thanks for your reply Tim, and for that interesting info.
I didn't think for a minute I would have been the only one to wonder why time was not included as a variable in assessing energy returns from energy investment, but it does seem that for now most are happy to invest their effort (and in some cases their careers) in working on EROI as if the time factor was of no relevance at all.
I'm not saying EROI isn't a valuable metric in itself, but for the most part - especially when discussing the transition pathways as we gradually leave behind the old world of conventional fossil fuels and embrace the next phase of unconventional and renewable resources - internal rate of energy return should be the most important metric.
This has been a fascinating couple of days of discussion for me. I started off not being quite sure whether or not there was some time component to EROI. I've learned that there isn't, and I've developed my own thinking about rates of return considerably, as well as picking up several ideas from others. Maybe one or two other people have thought a bit more about it as well.
I'd like to thank Professor Brandt for his original article, even though it was only about EROI it was very good, and his participation in the discussion that followed was very constructive.
internal rate of energy return should be the most important metric.
Well, it's certainly useful. OTOH, like E-ROI, it's less important than the financial IRR/ROI, and is most important in the rare cases where it's very low (as seen in your examples).
Both wind and PV return energy sufficiently quickly that E-IRR isn't really that important.
Why?
The developed world is not particularly short on energy at the moment, so we're not constrained to use the system that can grow the fastest. I would argue that a better model -- all else being equal -- would be to choose the option that requires the lowest gross input, as that will roughly correspond to the smallest diversion of society's productive capacity to achieve the goal of providing enough energy. This corresponds to EROEI.
For example, providing 20 units of surplus energy via an EROEI 1.2 system will require society to do the work to provide 100 units of energy as input every year, or 3,000 units of energy provided as input over 30 years. By contrast, an EROEI 6 system with a lifespan of 30 years will require the input of only 100 units of energy, meaning it requires only around 3% as much of society's effort.
Even an EROEI 2 system will require just 600 units of energy to be input, or 80% less than the first system. Given that our society has enough of a productive surplus to choose either system right now, why would the system which requires 5-30x as much input effort be preferred?
A great thought, but I think energy has a negative discount rate. The economist's idea of net present value boils down to this: it's better to spend money in the future than today, because there will be more money in the future. Leaving aside the question of whether this is really true for money, it's certainly not true for fossil energy. Quite the opposite.
"Leaving aside the question of whether this is really true for money, it's certainly not true for fossil energy. Quite the opposite."
For as long as we have the ability to invest in energy production with a positive Internal Rate of Energy Return (which is still true for most renewable energy) that should fundamentally and inherently be untrue, and discount rates should remain positive - albeit one would expect them to fall to a considerably lower number as we transition from fossil to renewable energy, a trend which in both cases has already started.
It's not true that if we invest in energy production with an EROI > 1 (which is what I think you mean), we will always have more in the future than we do now, because we're not just "reinvesting" our energy profits, we're using them to run our society, whereupon they disappear.
Well if EROI > 1 then the internal rate of energy return would mathematically be expected to be positive, but IRER is the useful term here (not EROI) for several reasons but not least because discount rates are related to rates of return expectations – even if the nature of the relationship is somewhat controversial. The importance of using the internal RATE of energy return (IRER) rather than EROI is exactly what I have been going on about, possibly at too much length for you to bother with, which would be quite understandable.
Anyway, I would like to try to break down what I think you are saying. You can tell me if I've got it right or not. There are two parts to it:
1. You are saying that we will consider energy in the future to be more valuable to us than energy in the present (i.e. that discount rates would be negative)
2. You are also saying that we will not be prepared to invest present energy to create future energy even though - not only do we think future energy is more valuable - but we would create more of it (an energy profit) by doing so
Well, I'm sorry, but if that really is what you are saying, then I think it's a bag of old nonsense. Anyway, if energy discount rates were negative, why wouldn't we just hoard energy (assuming for some reason we wouldn’t invest it) in the same way that people hoard money when achievable returns are very low?
if energy discount rates were negative, why wouldn't we just hoard energy (assuming for some reason we wouldn’t invest it) in the same way that people hoard money when achievable returns are very low?
The distinction is between what "we" as a society believe, and what I and other Oil Drum readers believe. Society believes, based on past experience, that fossil energy supply will continue to increase indefinitely. It is wrong, but it's that attitude that shapes the energy economy.
If you believe in peak oil, hoarding is absolutely the right response. That's why I'm opposed to expanded offshore and Alaskan oil drilling: not just because I'm an environmentalist (though I am), but because I want to hoard that stuff to be used in an energy-scarce future. Burn someone else's oil now, and keep the stuff in our own backyard for later.
Another reason to encourage fossil fuel hoarding: it creates scarcity now, forcing us to switch to renewables sooner rather than later.
Well, I still contend that theoretically the "rational" thing for you to do given your beliefs would not be to hoard energy but to invest it by turning into renewable energy projects if you can get a good rate of energy return. Then, when the scarcity starts to bite you will have more of it to use for yourself or sell. Also, if everybody started hoarding energy then it would become scarce in the present meaning discount rates would still be unlikely to be negative (you might then expect them to trend to zero or a very small positive number).
However, I do fully recognise that you are talking about your own personal real world concerns and as such there are real world constraints to deal with that are not covered by my very simplistic and theoretical treatment of energy returns - not least access to financial capital which in the real world is what you actually need to build anything (not just "energy"), but also that renewable energy mostly produces electricity which is difficult to store or use for transport while you are probably most concerned about liquid transport fuels.
Hoarding is not rational, it is something people do when they are concerned about the future. For a place like Alberta which (unbeknownst to most people) has vast energy resource alternatives of numerous different types, it doesn't make any sense at all. Alberta is not particularly dependent on its own oil - it is mainly a source of jobs and income. The conventional oil is nearly gone, but the oil sands are coming on stream at a sufficient rate to continue to increase employment, so that is not a big problem from Alberta's perspective.
The Alberta government does have measures in place to conserve the natural gas supply because everyone heats their homes and cooks their food with natural gas, but it doesn't consider oil critically important. After all, people can always run their cars on NG instead of gasoline with a conversion kit, and diesel trucks and locomotives can easily run on a mix of 10% diesel, 90% NG. The electricity production is migrating from mostly coal to a mix of NG and wind power, and Alberta has lots of all of them. The oil sands operators build big cogen plants that can deliver a large surplus of electricity to the Alberta grid as a profitable by-product of delivering process steam to the operations. There are even major undeveloped hydroelectric sites in the far North.
For some place like the UK, which has long since mined out its cheap coal, and whose oil and gas production is now in steep decline, hoarding some of it might have made more sense. It would be better than relying on Middle Eastern oil and Russian natural gas. However, that's just fossil fuels under the bridge so they have to think of something quick because they are getting into deep trouble. So far they don't seem to have done much thinking about it.
Too true about the UK. We have not begun to shift our previous massive reliance on the 'Financial Industry', which seems these days an ongoing liability rather than an asset. The EU as a whole must import oil and NG - and I guess we negotiate within that context and hope we are too big to fail. UK still imports a lot of coal for power generation - a lot of it from Russia. Actually we do not get much if any of that Russian NG - we were topping up with LNG from Quatar etc. when storage ran low back in the cold spell.
ERoEI = Eg/Ep; where Eg is the exergy of the petroleum fraction (assumed as 35.7 deg API for conventional crude, 140,000 BTU/gal) and Ep is the work required at the well head to extract the oil. ERoEI has no time variable attached to it because it is extracted from a Second Law statement (which has no time function associated with it). It is derived from the entropy production of the oil production system.
If the API of a fraction is known, the exergy for that fraction comes handily from an exponential function: Exergy (BTU/gal) = exp(-0.0045*API + 12.01). Reservoir engineers use a WOR (water, oil, ratio) of 40:1 to determine the economic life span of a well. A WOR of 40:1 determines when a well will be shut-in. A WOR of 40:1 translates directly for API 35.7 crude to an ERoEI of 7.2:1.
The mine-mouth ERoEI of 5.23:1 mentioned above would no longer be considered economical to produce for a conventional well. It is the low cost NG that makes Syn Crude production possible. The statement that without NG Syn Crude production could continue from bitumen combustion is flatly false!
I am not sure what you mean here, and cannot understand your method. Rapid expansion of oil sands output was occurring when gas prices were much higher in the past.
"I am not sure what you mean here, and cannot understand your method. Rapid expansion of oil sands output was occurring when gas prices were much higher in the past."
The method can be found in any Engineering Thermodynamics textbook. The meaning is that the utility of a fuel is dependent on the quantity of energy that it is capable of delivering to the end consumer. The premise being used here is that to act as an energy source any fuel must be able to provide more energy than it takes to produce it. If not, it becomes an energy sink, and is no longer economically viable unless it is subsidized by other energy sources.
The author's assertion that bitumen production can be accomplished with a mine mouth ERoEI of 5.23:1 using NG as a supplemental energy source allows for the determination of an energy budget for bitumen production. If the author is correct, (his data looks pretty messy) and Production Energy is calculated from the equation above (ERoEI = Eg/Ep), and Production & Distribution, and Waste heat included (calculations are not be shown here for lack of space) it gives:
Production energy --------- 18,614 BTU/gal
Processing & Distribution -- 75,023
Waste heat ----------------- 45,884
Total Production energy 139,520
Exergy of API 8.5 bitumen 158,220
Total Production energy 139,520
Energy to end consumer 18,700 BTU/gal
As a comparison the energy delivered to the end consumer from a gallon of conventional crude (API 35.7) in 2012 was 28,490 BTU/gal. It is obvious that Syn Crude without an external energy source would not be an economically viable product. If Syn Crude was produced only from the energy acquired by the combustion of bitumen, it would become an energy sink!
I am the study author. You are making claims that are entirely at odds with what I (and many others) have found, and are offering no evidence. I would argue that your conclusion is not "obvious" at all, since it contradicts the historical record (others have done in the past what you claim is impossible, and have done it at large scale).
As I have offered elsewhere in this thread, you can email me for the study: abrandt "at" stanford "dot" edu. If you have questions after reading the study, I would be interested to reply to them.
It is obvious because according to Syn Crude it requires an energy input of 1.0 - 1.25 giga-joules to process one barrel of bitumen. That converts @1 Gj to 22,567 BTU/gal, which is more than the SynCrude delivers to the end consumer.
The Processing & Distribution calculation (although we employee a different methodology) agrees closely with the EIA's determination of 16.3 K BTU/$ of finished product. Our result is within 5K BTU/gal of theirs. For a final number we are assuming that 1/3 of the product must be further reprocessed by vacuum distillation.
The Waste Heat determination is derived from the calculation of the maximum Second Law thermal efficiency, which says that any hydrocarbon with a molecular weight greater than heptane has a SLE of no more than 71% (29% waste heat production).
To do a complete analysis it is necessary to look at the entire process (extraction, processing and distribution). To do that you can not use dollars equivalent terms without employing their time correlation function. This entire exercise is a direct application of the Entropy Rate Balance Equation for control volumes. Which is a Second Law statement.
Shortonoil, it's obvious you haven't read the study very closely, and that you yourself are not working with real-world data.
The NER number of 5.23 the author calculates is in GJ/GJ and is determined at outlet of the plant or separation facilities (not really the "mine mouth"). The output would be bitumen (8° to 10° API) if the project had no upgrader, or syncrude (30° to 32° API) if it did. The consumption energy in NER includes internally produced solid coke and/or process gas and/or syngas produced by the upgrader, if there is one, and/or produced gas from the SAGD or CSS wells if it is an in-situ project.
The trouble with this is that the typical oil sands project is producing quite a bit of the energy it consumes itself, and this isn't really relevant to an EROEI calculation. You have to draw the boundaries around the external limits of the project and not consider internal production/consumption.
The NEER number he calculates is a net energy value closer to what most people consider EROEI. It uses only external input and output energy. This apparently has gotten up into the range of 20 GJ/GJ for the mines with upgraders these days. (I had been using 12 GJ/GJ but that might be an obsolete value).
Also, the author is working with original Alberta Energy Resources Conservation Board data, which is as good as it gets. The companies have to report all their production and consumption data in detail to the AERCB monthly, and there are legal penalties for misreporting. The AERCB accepts and reports all its data in metric units, so the energy would be in GJ rather than BTU, volumes in m3 rather than gal or bbl, and density in kg/m3 rather than °API. You can convert if you want to, but there's really no point because your calculations are wacky.
Any form for FF will be depleted. If you can switch society to renewables - solar derivatives that whole game stops. look at the link in my most again.
(yes negative entropy doesn't exist, but think of it as a concept - an energy concentrating activity rather than an energy diffusing activity.)
Rgds
WP
I don't think WOR determines ERoEI, and I don't think ERoEI determines when they shut in the wells. I know of entire oil fields that are operating at a WOR of 100:1. They plug and abandon the wells when they fail to produce enough oil to recover their operating costs, and their operating costs were very low. In one case, the reservoir pressure had fallen so low that the injection wells were sucking the water into the reservoir, and the wellhead pumps were running on electricity because there was almost no NG left, but the whole thing made money so it kept running.
They could run oil sands plants using syngas produced from bitumen, and in fact it is being done, but at this point in time burning residual coke, process gas, and purchased gas is cheaper. If the price of NG goes up, bitumen gasification might become more popular.
Adam, thank you for a very clear and informative article. I think NEER is a valuable insight that makes a lot of sense. I wonder though, if some part of the 'societal opportunity cost' of the employment at the mine should be - somehow - included in NEER. Seems to me there should be some reflection of the fact that as more workers are diverted to energy production there are fewer workers producing other stuff. Problem is you kind of end up back measuring GDP/person instead of GJ...
Anyway, thanks again!
Jon.
Adam
What is your current estimate for EROEI of American kerogen ("oil shale") in
Utah, Colorado, and Wyoming?
Thanks
I looked at oil shale from 2007-2011 or so. I did EROI for ICP, ATP, and a speculative fuel-cell based approach. Here are some sources:
Mulchandani, H., A.R. Brandt (2011). Oil shale as an energy resource in a CO2 constrained world: The concept of electricity production with in-situ carbon capture (EPICC). Energy & Fuels. 25(4): 1633-1641. DOI:10.1021/ef101714x
Brandt, A.R., J. Boak, and A.K. Burnham (2010). Carbon dioxide emissions from oil shale derived liquid fuels, in Oil shale: A solution to the liquid fuels dilemma, O. Ogunsola, Ed. ACS Symposium Series 1032. American Chemical Society: Washington, D.C. DOI: 10.1021/bk-2010-1032.ch011
Brandt, A.R. (2009). Converting oil shale to liquid fuels with the Alberta Taciuk Processor: Energy inputs and greenhouse gas emissions. Energy & Fuels 23(12): 6253-6258. DOI: 10.1021/ef900678d
Brandt, A.R. (2008). Converting oil shale to liquid fuels: Energy inputs and greenhouse gas emissions of the Shell in situ conversion process. Environmental Science & Technology, 42(19): 7489-7495. DOI: 10.1021/es800531f
Get in touch if you want any of these papers: abrandt "at" stanford "dot" edu
Hi Jon,
Thanks for the positive feedback.
I agree that there is opportunity cost of internal energy use. That is, the gross output of the mine has to be larger than it would be otherwise due to the parasitic consumption (per unit of "net" output to society, of course).
This is an interesting area for future research.
Adam
I had a bit of a discussion about this with RMG a few months ago.
It seems these EROEI calculations aren’t simple, and ultimately in my opinion, can’t really be narrowed down to a single definitive EROEI figure.
He points out that much of the internally produced and process energy (eg still gas), as well as the purchased natural gas, used by the upgraders can be used “twice”: they can send it through a gas turbine to produce electricity and then put the exhaust through a HRSG to produce low grade steam via cogeneration to pull more bitumen from the ground. Apparently they sell this excess electricity to the grid. This then begs the question: how do you incorporate that electricity / cogen heat into EROEI calculations? A typical combined cycle gas plant isn't cogen and wastes 35% of its heat, but in the oil sands they recover most of that for further bitumen extraction.
The charts on page 5-54 of this document are revealing: http://www.ercb.ca/sts/ST98/ST98-2012.pdf
From those charts it should be fairly easy to compare with produced bitumen per day and come up with an EROEI for the whole Alberta oil sands sector. The biggest question mark is how you define EROEI and therefore which numbers do you plug in and divide. It’s difficult to compare apples to apples in this case with other energy sectors.
Alberta produced in 2011 637 million barrels of raw bitumen (page 2). Of this, 315 million was upgraded SCO (page 10). This is a bit less that what your Figures 3 + 4 suggest of total production of oil sands stuff of 800 million barrels equivalent (based on my eyeballing of your charts of 270,000,000 + 120,000,000 GJ per month which converts at 5.86 GJ per barrel oil equivalent).
Gas consumption is as follows: About 1/3 of the total gas used by the industry (1.4 or 1.9 Bcf/d depending on what chart you want to use, i.e. if you want to include cogen or not) is from internally produced and process gas. That is a lot.
1.9 Bcf/d corresponds with 120 million barrels of oil a year. Divide into 800 and we get an EROEI of 6.7. If we instead only include the produced oil (I don’t think bitumen itself used for road construction and other things like that is useful as an energy source), then 315 divided by 120 is an EROEI of 2.6.
If we subtract out the internal process gas (I estimate 0.6 from the chart on page 5-54), since it could be argued that this shouldn’t be part of an external EROEI calculation, then we get 1.9 minus 0.6 which equals 1.3 Bcf/d and an EROEI of 3.8. A bit better. These are very low EROEI’s, maybe I missed something. But the numbers are all right there, straight from the Alberta government.
But I argue that this internal process gas should be included in EROEI because as EROEI drops in the future from increasingly poor oil sands deposits, it’s going to eat up an increasingly disproportionate share of the total energy liberated from the produced bitumen, more so than if that gas all came from purchased external gas with a higher independent EROEI. It isn’t possible to have a perpetual motion machine with an EROEI less than 1:1. So assuming no external gas was used and all came from internal process gas, then internally-powered oil sands operations would cease as they approach 1:1. On the other extreme, if the oil sands operation was powered by external natural gas only, then the EROEI of the oil sands itself could indeed go below 1:1, and this would be made possible from the profit due to the price arbitrage between NG and oil on a BTU basis. But the overall EROEI of the NG/oil system would still have to be greater than 1:1, because the energy losses in the oil patch would be more than offset by the positive EROEI of the external NG extraction. In this case, the oil sands would not be a source of energy; merely a carrier, of the net surplus energy from the cheaper external natural gas.
The other issue is that the reason oil sands production can’t be ramped up quickly is because of the very high capital costs associated with building these upgraders, etc. They cost billions. All that infrastructure represents a huge amount of embedded energy that is not required for conventional oil. We need these billion dollar upgraders just to turn the bitumen into oil equivalent to what comes straight out of the ground in North Dakota. Considering how much these things cost, I imagine that this embedded energy in infrastructure would be quite significant, in terms of all the pipes, valves, tanks, pumps, labour, supporting communities, food for the workers, trucks, etc. etc., all of which require energy... from somewhere else. It should really be incorporated into the EROEI calculation if we’re trying to get an idea of society’s overall EROEI so we can predict if and when we’re all going to die. Because, while it may be difficult to single out individual oil “production” industries and peg a definitive EROEI value associated with them when different sectors of the economy are so intertwined; on the whole, collectively, when we put all the energy “production” sectors together, they do indeed need to have a significantly positive EROEI, because you can’t violate the laws of thermodynamics. From what others say, that seems to be around 8:1. It seems we’re pretty close to that. I would guess that coal and natural gas are subsidizing the oil sector right now to keep overall EROEI above 8:1, and that's only possible because of the BTU price arbitrage from liquid oil being more useful than solid coal or gaseous methane.
The other reason we're getting as much investment as we are is because of the ridiculously low interest rates from the US dollar / Wall Street / derivatives ponzi scheme financial system we are all enslaved by. After money hyperinflates and interest rates return to something more sane then we will not have all this investment since money will be more reflective of reality. Because of this, Peak Oil will be a very clearly defined shark fin and oil production will drop like a rock when we cross the wormhole through to the other side.
Null... I actually agree with everything you stated in your comments. It seems as if the Capital invested (in energy) is not taken into consideration. I have seen ROI studies on Wind Farms in the United States that don't pay back the original investment before its 20-25 year depreciated lifespan.
Furthermore, Deborah Rodgers at the Energy Policy Forum puts out some interesting information on how the trucks fracking and transporting the shale oil & gas are destroying the roads and there's only a fraction of tax revenue to maintain the roads.
Basically, the oil and gas companies are off loading the burden to local tax payers. She states, " But the companies who have generated these costs are not paying for those costs. They have off loaded that significant burden onto the tax payers and local businesses. This is true of the oil and gas industry as a whole. In fact, economists estimate that if all oil and gas externalities were included gasoline would cost in excess of $12/gallon. "
I don't know if that $12 a gallon figure is correct, however we can see that we have gone past the ability to pay for the energy we are currently using... if we factor in that we import goods and export inflation.
Just wait until the FED can no longer push on a string and those worthless $trillions of dollars and U.S. Treasuries come back to roost in the USA. Talk about inflation.
Some comments:
"It seems these EROEI calculations aren’t simple, and ultimately in my opinion, can’t really be narrowed down to a single definitive EROEI figure."
- Agreed. See above comment for two papers outlining the mathematics of energy returns and arguing that the term EROI is poorly defined and inconsistently applied.
"He points out that much of the internally produced and process energy (eg still gas), as well as the purchased natural gas, used by the upgraders can be used “twice”: they can send it through a gas turbine to produce electricity and then put the exhaust through a HRSG to produce low grade steam via cogeneration to pull more bitumen from the ground. Apparently they sell this excess electricity to the grid. This then begs the question: how do you incorporate that electricity / cogen heat into EROEI calculations? A typical combined cycle gas plant isn't cogen and wastes 35% of its heat, but in the oil sands they recover most of that for further bitumen extraction."
- You aren't really using energy "twice" because conservation of energy dictates that energy cannot be "consumed" per se. What you are doing with cogeneration is getting more exergetic value (thermodynamic work) from a given energy stream as electricity, and generating lower value steam using the residual lower quality energy stream. Cogeneration is used extensive in California and Alberta heavy oil and bitumen extraction operations that use thermal recovery (e.g., SAGD, steam injection, CSS). We account for all cogeneration operations in this analysis, and count the energy value of electricity exports. This does not end up being a major driver of the results.
"From those charts it should be fairly easy to compare with produced bitumen per day and come up with an EROEI for the whole Alberta oil sands sector. The biggest question mark is how you define EROEI and therefore which numbers do you plug in and divide. It’s difficult to compare apples to apples in this case with other energy sectors."
- We go into this in great detail in the paper and supporting information. We compute 8 different ratios, because there are different ways of looking at the problem. We use all ERCB statistics in conducting our analysis, and spent 18 months compiling, cleaning, and analyzing the data for this paper (and another). Please feel free to email about the paper. Believe me, we have thought about all of these issues in exhaustive detail. Email me at abrandt "at" stanford "dot" edu.
"But I argue that this internal process gas should be included in EROEI because as EROEI drops in the future from increasingly poor oil sands deposits, it’s going to eat up an increasingly disproportionate share of the total energy liberated from the produced bitumen, more so than if that gas all came from purchased external gas with a higher independent EROEI. It isn’t possible to have a perpetual motion machine with an EROEI less than 1:1. So assuming no external gas was used and all came from internal process gas, then internally-powered oil sands operations would cease as they approach 1:1. On the other extreme, if the oil sands operation was powered by external natural gas only, then the EROEI of the oil sands itself could indeed go below 1:1, and this would be made possible from the profit due to the price arbitrage between NG and oil on a BTU basis. But the overall EROEI of the NG/oil system would still have to be greater than 1:1, because the energy losses in the oil patch would be more than offset by the positive EROEI of the external NG extraction. In this case, the oil sands would not be a source of energy; merely a carrier, of the net surplus energy from the cheaper external natural gas."
- Oil sands operations net energy returns (whether including or excluding internal consumption) have generally been improving. We are very far from 1:1 energy returns. Eventually, we will be "scraping the bottom of the barrel" here and quality will decline, but many many billions of barrels will be produced between now and then.
"The other issue is that the reason oil sands production can’t be ramped up quickly is because of the very high capital costs associated with building these upgraders, etc. They cost billions. All that infrastructure represents a huge amount of embedded energy that is not required for conventional oil."
- Conventional oil investments, especially offshore, can represent billions as well. Fossil energy sources typically do not have energy returns driven by capital investment, as the quantities of energy consumed on a day to day basis dwarf the energy embodied in steel etc. Many studies have shown this. Future work could include these flows, but my past experience suggests that they are likely an order 10% increment on the numerator of any of our 8 ratios.
Hi Adam, I agree with most of what you say in the above comments and your paper.
Yes, I know the energy isn't used "twice", but for accounting purposes, if you were to compare the cogen plant to a naked combined cycle plant without cogen then that heat is a "free" energy source.
Thanks, I'll email you for the paper.
My only real quibble with what you are saying, without having yet read your full report, is the title of the post here, "Low energy return on investment (EROI) need not limit oil sands extraction", and the argument along with this that seems to be that when you remove from the EROEI calculation the internally produced and process gas, plus coke and all the other parts of the extracted bitumen that get burned to provide the "invested" part of the EROEI calculation, then the real oil sands EROEI experienced by the rest of society jumps up from 6 to 20 GJ/GJ as you say. I think this is a bit misleading, because if you're trying to tease out "energetic returns relative to external energy provided by other energy sectors. That is, relative to the amount of energy that they consumed from the rest of society", then you really should be incorporating ALL external embedded energy to be consistent, and this is not just diesel and natural gas, but additionally every bit of coal and natural gas that was burned somewhere else on the continent or in China to manufacture every piece of steel that went into the capital investment, every bit of natural gas that went into the agricultural activities needed to support the society up in Ft McMurray, and anything else remotely related to providing for those oil sands operations, because this amount of energy is not available to society for purposes other than for providing for oil sands extraction. You say this amounts to a 10% increment, but I would like to see some more evidence of that, specifically for a typical new facility in the Alberta oil sands.
The title of the piece and its contents imply that the true EROEI of oil sands is 20:1 which would suggest that we still have a ways to go before we get to some lower limit required to maintain society. But this doesn't change the fact that the actual EROEI of the processes that are extracting and converting bitumen to oil (your NER's) have an EROEI of around 4 and 6. This is the number that becomes important for when the quality of the remaining oil sands deposits drops as we cream out the best deposits first, not the 20:1 number. As the required energy to extract bitumen increases (the EROEI "cliff") it will be taking away from the 4:1 and 6:1 ratios, not 20:1. It doesn't take much to send that down into near negative territory. 4:1 or 6:1 are a lot closer to 1:1 than 20:1 is. I guess there will be two opposing forces directing the future progression of overall oil sands EROEI: tending to decrease it will be deteriorating quality of remaining reserves. Tending to increase it will be technological innovations which increase overall efficiency. The problem is, both of these trends are going to look rosy in the beginning, but will conspire to really create problems as they progress. Oil sand quality may be OK for a a few decades, but when it falls, it's going to get progressively worse, especially when we're starting near 5:1 with the good deposits (how soon the quality of the remaining reserves will drop, I don't know -- are we talking 10 years? 20? 50?). Compounding this will be the fact that the technological innovations will be made at the beginning, and I doubt we'll see anything significant beyond 10 years into the future because the biggest and best innovations are made first. This means we may be able to maintain overall EROEI of 5:1 for a few decades and maybe not even need any external energy at some point, but it won't take much in terms of reduced oil sand quality to push us into danger territory.
And of course, the entire oil sands deposit represents 6 years of global oil consumption (based on current reserves), or 10 years (based on projected future recoverable reserves).
"And of course, the entire oil sands deposit represents 6 years of global oil consumption (based on current reserves), or 10 years (based on projected future recoverable reserves)."
Isn't it wonderful, completely beyond any accounting by the science of economics!
But that ratio is irrelevant since oil sands production will never approach current conventional oil production levels. You need to turn that statement around and say, "The entire Alberta oil sands proven reserves represent 186 years of production at current production rates, or 93 years at projected future production rates."
What will really happen is that world conventional oil production rates will fall to less than oil sands production rates. 100 years from now, there won't be much conventional oil left in the world, but there will still be significant oil sands production - both in Canada and in Venezuela. Production rates and consumption will not however be anything like current global oil consumption. Current global oil production rates are unsustainable in the long term.
By the way, the 170 billion barrels of proven oil sands reserves ARE the high grade 10% of the resource. The total resource is around 1700 billion barrels, and as the initial 170 billion barrels are exhausted over the next century, higher prices and improved technology will bring more and more of the lower grade remainder on production. Remember, almost all of the world's conventional oil will be gone by then and the small amount of oil sands production will be almost the only available source of oil worldwide. Prices will be astronomical for those few who can afford it.
You really believe that we will be enjoying "improved technology" in terms of oil sands extraction and processing a century from now? I give it 10 years more, then all the main innovations will have been made (we may be getting close right now; they've already had a decade and many billions of dollars to think about and tinker with these things). We all know they have a world of incentive to innovate, and people these days are pretty smart. I have a hard time believing there's many significant unknown processes hiding under the rocks awaiting discovery. It's not like they're transitioning from internal combustion engines to electric cars -- a totally revolutionary, potentially disruptive shift. Rather, they're transitioning from bitumen and natural gas to ... bitumen and natural gas. With maybe some coal thrown in as well. I mean, really, how many different ways are there to burn stuff, pump it around, and transfer the heat? There's only so many permutations to that theme.
Do you truly believe that these very expensive and complex upgrader facilities, which we currently have a hard time building, will actually be built after the world runs out of regular oil and only Alberta and Venezuelan oil sands remain? Where are all the components going to come from? Who will make the pumps, valves, electrical, pipes? The world will be in chaos; we won't be concerning ourselves with building megaprojects in Alberta. The only oil we'll be burning will be the dribbles from stripper wells and any remaining whales to be killed -- and maybe CTL, but I doubt society will be in much shape to be building them, either. Wars tend to destroy those kinds of complex facilities.
"higher prices and improved technology will bring more and more of the lower grade remainder on production."
But if those lower grade remaining deposits have a NER EROEI approaching 1:1 (not much of a stretch considering we're at 5:1 today), then they won't be a source of energy, and price won't make any difference. If we're running out of natural gas by then, which I fully expect, then where is the energy going to come from to drive the future oil sands production? Coal? Then we will be transitioning to a coal based energy system, not oil.
I agree that oil sands production rates will never ramp up to offset global oil production declines; that's the whole point. The best we can hope for is that it might keep North America going for a few more decades than otherwise, helping to offset the current North American oil trade deficit which won't be an oil trade deficit for much longer after the US dollar dies, and provide an opportunity for N America to move onto other sources of energy. Based on how our treasonous politicians behave, however, I doubt we'll take the opportunity to do so. They'd rather sell our country to Chinese communists.
The other day I got my copy of Process West magazine. In it was an article suggesting how the energy sector needs to "educate" a largely "ignorant" populace about energy; how so much misinformation is being spread by interests opposed to energy development; and of course about about how much prosperity and tax revenue (and wonderful smiley face things like schools and hospitals and daycare centers! -- funny how Alberta still has a major deficit problem to fund these kinds of smiley face things despite its huge recent energy development... I guess we just aren't developing our energy resources fast enough, that must be the problem!) we'll all be enjoying if we'd just open the floodgates to communists and sell off our natural resources! And I got the impression that the (Calgary based) author actually genuinely believes what he wrote. The amount of sheer ignorance and hubris exhibited by Canada's political and corporate leadership wrt to the energy sector is truly staggering. Presumably Stephen Harper, being an oil man himself (and an economist to boot), echoes these views.
I gave the Northern Gateway hearing almost a hundred pages of analysis, along with 10 minutes of extremely fast speaking on my part, laying out the real facts along with supporting data, which seemed to open the eyes of the three NEB panelists. Whether that filters up to the level of the actual decision makers, who knows. Even if it did, the system is so corrupt it probably wouldn't matter anyways. Although, the Northern Gateway propaganda seems to have ended lately, so maybe they realize it's just too much of a lost cause.
I agree that we shouldn't trust fossil fuel companies' claims about ever-increasing innovation. But ... wait a second, there's someone here at the door. Oh, hey, it's an energy expert from 1980. He says:
You really believe that we will be enjoying "improved technology" in terms of natural gas extraction and processing a century from now? I give it 10 years more, then all the main innovations will have been made ... I have a hard time believing there's many significant unknown processes hiding under the rocks awaiting discovery.
Do you take the POV of Patent Office Commissioner Henry Ellsworth in his 1843 report to Congress?
In fact Ellsworth was setting up a straw man argument, and subsequent patents by Edison, Tesla, Ford and others rendered his sarcastic prediction wrong. In 1843 everything that could be invented had not yet been invented and in fact the number of patents exploded after that date.
The same is true of the oil sands, and there are a lot of innovations coming down the line that haven't impinged on the public conscience yet. In fact, a lot of innovations that have already been made haven't become public knowledge yet, hence the large improvement in EROEI unbeknownst to the unwashed masses.
There is a lot of new technology in the prototype or pilot state that few people know about. My favorite is the use of rocket engines to generate steam for in-situ production. This is not a joke, the underemployed ex-space shuttle engineers have build a prototype, and some oil companies are seriously considering spending a few million on a pilot project to see how it works in real life. If it is economic, it could be really, really good.
There is a lot of oil sands stuff under development. Most of it is in prototype, some of it is in pilot. Most of it will come to fruition post 2020, but to stay ahead of the game you have to think outside of the box. Don't think inside the box, don't try to make the box smaller, think outside of the oil sands box. For instance, consider the "carbonate trend", which is outside the oil sands box but where companies are already drilling wells and booking oil reserves. If they missed the oil sands boat, there's always the carbonate trend to fall back on. It just needs more research but is potentially bigger than the oil sands.
RMG, I just can't buy into the argument that humanity will continually improve; this doesn't pass the test of ecology. It's the economists' fantasy that's destroying the world: "Don't worry your pretty little heads about resource depletion or pollution today, because future technology will find and bring forth new resources. Trust us! And just keep consuming! Ben Bernanke knows what he's doing."
It never fails to amaze me how far people will go to convince themselves to deny the fact that we are completely dependent on ecological productivity for our survival and how we have clearly overshot the planet's carrying capacity many fold. Hopium is an addictive drug. Of course, a look through history reveals the complete opposite and the simple undeniable fact that almost all of our technological advances wrt energy have been for the purpose of finding additional deposits of dead things to burn, to extract them more efficiently, and to burn them more efficiently. The only real exceptions to this are nuclear, which seems to be well on its downward spiral, and PV, which is still very small in overall contribution but has great growth rates and is essentially our one and only hope. The chances of it coming online fast enough to save us from major social and economic catastrophe seem about nil, however.
If you look at an animal population, say African elephants, we'd all agree that their populations and survival are totally dependent on the underlying ecological productivity supporting them, which is all a result of the annual rains and sunshine stimulating plants to grow which the elephants and antelope eat, which then provide food for the crocodiles and lions. If you go a few thousand kilometers northwest to the Sahara, you will see that there are no elephants. Why is that? Because there's no rain, so there are no plants and no ecological productivity available to support elephants. Duh! Pretty obvious, how could anyone disagree? We all learn this from science class. Yet somehow, we delude ourselves into believing that people are somehow different than elephants because we gots technology, that somehow we are not dependent on ecological productivity, when this is in fact completely false. 97% of our energy comes form burning dead things that used to be alive (we just managed to additionally access dead things from hundreds of millions of years ago and burn them at a rate 1,000,000 times greater than deposition rates). You'd think the important question should be front and center in the discussion right now: "How much ecological productivity is there available to support our societies? (i.e. how many dead things are there available to burn?" Instead, we seem to be asking the opposite, illogical question: "How can we increase our burning of dead things from a rate of 1,000,000 times greater than deposition rates to 2,000,000 times greater than deposition rates?"
Instead we get Star Trek fantasies about "quantum zero-point diffusers or some other Star-Trek-like energy source.". (Whether you're being facetious here I'm not sure, but the fact remains that most people actually believe in this stuff, and if Stephen Harper can spread his "Jobs, Growth, Prosperity" propaganda along with images of some attractive young person wearing a hard hat, ready to be productive and go to work, then people will tent to re-elect him).
As I say:
The problem with Point 1) is that when it comes to oil, we've moved from a NER of hundreds or thousands down to about 5. I know the NEER is higher, at about 20, but this ignores the underlying processes involved in extracting the oil, and is only a valid metric as long as the NER remains above 3 or 4. Once the NER goes below 3, then the amount of energy needed to be recycled into the process to bring forth more oil overwhelms the energy outputs, eg, if you need to input 1 unit of energy to produce 3 units of bitumen-derived energy, that's still fine if you're using cheap natural gas to do it. But if you instead recycle the bitumen energy (to provide for a NEER of "infinity") then suddenly you have to use up a full 1/3 of the liberated energy. If the NER drops to 2:1 then in order to maintain the oil sands as a net source of energy then you have to burn up 1/2 of the liberated energy. Go to 1:1 and then that's it! So, basically, we now have a factor of 2 or 3 which is enabling the oil sands to remain a net supplier of energy.
The problem with Point 2) is that we're basically at the efficiency limits in terms of how we burn and use hydrocarbons in our societies.
The problem with Point 3) is that renewables have a long way to go to make a dent in the overall energy picture and they generally only produce (intermittent) electricity, which is far less useful than hydrocarbons. Furthermore, our population and economies tend to grow at a rate comparable to increases in renewables, meaning they merely tread water.
These three problems, combined, point to some very difficult times ahead, and in such times I don't think we can count on many of the just-in-time efficiencies we currently rely on to hide energy scarcity to continue. History shows that empires collapse violently, not gracefully -- The problem is, today it's the whole world that is the empire: the human empire that has now dominated all other species. This will not provide an ideal environment bringing forth advances in radical new technologies that increase oil sands NER from 5:1 to 8:1 so we can all continue burning infinite amounts of dead things.
As to the rocket engine steam generators, what specifically is their advantage? The gas turbine and HRSG combinations they're currently using are already pretty efficient, probably like 50% I would guess. We have a Carnot limit of around 70% so what do the rockets do?
In fact, a lot of innovations that have already been made haven't become public knowledge yet, hence the large improvement in EROEI unbeknownst to the unwashed masses.
But, as stated in Adam's article here, EROEI has improved only from "1.0 GJ/GJ in 1970 to 2.95 GJ/GJ in 1990 and then to 5.23 GJ/GJ in 2010". That's not huge by any stretch of the imagination. That's basically just clawing our way back from the energy cliff, but still on the precipice risking falling off again.
It's the economists' fantasy that's destroying the world: "Don't worry your pretty little heads about resource depletion or pollution today
That's not the message from economists, that's the message from the oil, gas and coal companies!
The reality: we have all the tech we need, and it's affordable, scalable, high E-ROI, etc, etc. The tech is being blocked by a small minority who would be hurt by it: employees and investors in FF companies (mostly the investors).
97% of our energy comes form burning dead things that used to be alive
Look around: 99% of our heat, and 95% of our lighting comes from the sun. Without it, the earth would be about 400 below zero, and very, very dark!
"That's not the message from economists"
Have you not listened to anything the Federal Reserve says?
"The reality: we have all the tech we need, and it's affordable, scalable, high E-ROI, etc, etc."
Nick, this constant delusional PV worship is getting beyond absurd. How much of N America's energy comes from PV and wind? How much hydrocarbons are produced from PV and wind? How much of our previous hydrocarbon-burning infrastructure has been converted over to use electricity? How much artificial photosynthesis is there in commercial production converting atmospheric CO2 to hydrocarbons?
I'm all for PV development but we need to be realistic. We have about two years or less to pull it all together and largely replace N America's energy infrastructure.
"99% of our heat, and 95% of our lighting comes from the sun."
Yes, via photosynthesis in plants and the deposition of said carbon molecules in the ground over the last 300 million years. Then we come along and harvest recently dead things (biofuels) or ancient dead things (fossil fuels) and burn them all.
Have you not listened to anything the Federal Reserve says?
I've read quite a lot of research from the Fed, and I haven't seen anything about non-depleting fossil fuel.
How much of N America's energy comes from PV and wind?
Again, almost all of N Am's energy comes from the sun. Look outside: where does the light and heat come from? Human HVAC only adjusts temperatures around the margin, and human lighting is a tiny fraction of the light we take for granted. That's why "Zero-energy" homes are possible: the sun provides all the energy needed.
As far as human-captured/transformed energy goes, I believe wind is at about 4% for the US. It could be 50% in 20 years with a modest effort.
How much hydrocarbons are produced from PV and wind?
??
Who needs hydrocarbons from PV/wind at the moment, given our supply of cheap oil, gas and coal? Obviously, the cheap and easy thing is to reduce hydrocarbon consumption before going to synthetic substitutes.
We have about two years or less to pull it all together and largely replace N America's energy infrastructure.
Where did this number come from????
"Look outside: where does the light and heat come from?"
Thanks for the reminder, I must have missed that observation! I guess you'll be the first one to voluntarily revert to the Middle Ages and rely on fully passive heating and lighting from the sun in a Toronto winter to cook your dinner, illuminate your book at night, keep you warm, and move you down the street!
Your rooftop PV setup would work much better, you say? Yes it would, but that's not passive and I'll again revert back to my original question which you already answered: how much of N America's energy supply comes from wind and PV?
"Who needs hydrocarbons from PV/wind at the moment, given our supply of cheap oil, gas and coal?"
Nick, I'm going to start ignoring you because you are making less and less sense the more you post.
Coal is cheap, for sure, and will probably continue to be (although China seems to want our coal deposits so that may push the price up).
I don't know if you've noticed the price of gas at the pump. It's going up and up. And that's with N America importing 1/3 of its oil. That's with all this short-lived Bakken production that's only possible because of the Wall Street interest rate scam. When US oil imports end and the dollar dies you won't believe how expensive oil will become.
Natural gas supply can't possibly keep up with today's consumption rates and there isn't enough new well drilling going on to maintain production.
"Where did this number come from?"
Any remotely realistic analysis of US debt and gold supplies. After the dollar dies things will be much different. A very brief sampling:
http://www.paulcraigroberts.org/2013/04/11/pcr-interview-with-greg-hunter/
http://truthingold.blogspot.ca/2013/05/must-read-essay-on-gold.html
"My favorite is the use of rocket engines to generate steam for in-situ production. This is not a joke, the underemployed ex-space shuttle engineers have build a prototype,"
Interesting, just last week I was at the California Science Center in LA and got up close and personal with the Space Shuttle Endeavour before opening hours. Just me and the shuttle. They have an engine on display. And you can see the impact gouges in the underside tiles from the last mission, they didn't replace them. Kind of scary.
They are going to build a new building for it where it's standing up vertically rather than horizontal.
That's right Null:
The Enbridge N Gateway is a lost cause and so is the KM TMX expansion pipeline.
So thanks for your effort at the NEB hearings...every little bit helped in the effort to thwart the risk of supertanker spill risk on the entire BC coast.
Any chance I could read your submission on the NEB doc website?
Ward
This may be a double post but I just put the transcript up here:
http://markbc.net/
Prices will be astronomical for those few who can afford it.
Prices will be capped at the cost of producing synthetic liquid fuel, which will probably fall below $5/gallon.
Heck, it's very likely that a world powered by wind and solar will have large amounts of cheap power available during periods of peak production. The sensible thing to do is turn some of that power into synthetic hydrocarbons. Given that the power input will be cheap, synthetic liquid fuel could easily cost in the range of $3.5/gallon. That gives a maximum price of about $150/bbl (and crude oil would need to be discounted to account for refining).
Let's say wind provided 50% of the kWhs needed by the grid, and wind was overbuilt by 50% to reduce the frequency of lulls. That would create surplus power to the tune of about 25% of demand. That might be sold to synthetic fuel producers for, say, 2 cents per kWh.
Diesel has about 40 kWhs, so at 40% efficiency the energy input would cost about $2. The hardware might get 50% utilization if we're using surplus power, and that might raise capital costs to around $1 per gallon. Operating costs might get us in the range of $3.50 per gallon, which seems pretty feasible. Not quite competitive right now, but awfully useful in the long range.
And, of course, if there's a lot of surplus power you might get it for $.01 per kWh. Actually, this demand source would probably set the price for surplus power.
All of the tech needed for this exists right now, even if it's not quite competitive with the current moderate price of "rock oil" (petr-oleum).
I did not save the link, but i was reading this article about the Canadian Tar Sands and this guy involved with it's production said that all the high grade sands have been removed and processed and they are now working on removing the lower grade material which is all that remains. I regret not having saved that article - didn't think i would be commenting on this subject.
He's wrong, they are nowhere near depleting the high-grade reserves in the oil sands. They have generations to go before that happens.
He may have been talking about individual mining leases. Some of the older mines have been operating for over 40 years, and they may have mined out all the high-grade deposits in the particular lease. However, when that happens they can just move the mining and processing equipment to the next lease.
Eventually the mineable portion will be mined out. After 40+ years, they have mined about 715 km2 or 15% of the 4750 km2 of mineable sands. At current rates they will mine out the remainder at a faster rate, probably before the end of the century. However, the portion which is producible by in-situ methods is 10 times the size of the mineable area, and that will take longer to produce - it will last into the next century.
So far, technological improvement has been running ahead of resource depletion and the size of the economically producible resource has increased tremendously as a result, to over 170 billion barrels, despite continuous production. That is illustrated by Adam's paper:
(bold italics mine)
6 GJ/GJ is the number I have been using recently, although I have been getting a lot of pushback from people who are reading studies based on the 1990 number. This is an example of continuous process improvement, and the rate of improvement is not slowing. A century or more of resource remaining gives considerable time for additional technological advances in producing the rest of the oil sands. The pushback is coming from people who don't believe that things can ever get better.
Would definitely agree here. Resources in place are 1.5 to 2 Tbbl. If history is any guide, current reserves of 200 Gbbl or so are are likely to be an underestimate (perhaps a significant underestimate) of what we will be able to get out in the end (if we want it).
I would not hold my breath for depletion effects to raise the price of the oil sands. High gas prices perhaps. Transport constraints perhaps. Climate policy perhaps. But there is a lot of hydrocarbon in place, and lots of smart folks working on getting it out of the ground.
Rate of extraction may be limited (especially if transport constraints continue to bind) but I would be cautious in arguing about depletion of the resource base.
the energy value of electricity exports
Which raises the "energy quality" question - shouldn't such exports by weighted by a quality factor of about 3, given that electricity is about 3x as useful as primary energy, and converts from and to primary energy with the same ratio?
That illustrates one of the fundamental limitations of EROEI - it only measures energy and fails to take into account other valuable products. One of the attractions of oil sands bitumen for some Midwest refineries is that they specialize in producing asphalt for road paving, and the high asphalt cut of oil sands bitumen is highly attractive to them.
The sulfur which is a contaminant in many grades of oil, but is particularly present in the oil sands is stockpiled in big yellow piles and sold to make sulfuric acid, fertilizer, and pharmaceuticals, with the big markets being in Asia. Alberta is one of the world's largest producers of sulfur, partly due to its oil sands plants but mostly due to its large sour gas plants.
The solid coke is generally burned for fuel in the oil sands, but if there were steel mills nearby they could use it to produce steel. It could also be sold to China, which makes half the world's steel, but they are more interested in Alberta's ubiquitous reserves of low-sulfur metallurgical coal.
Even the CO2 from the smokestacks would have a market in CO2 miscible injection projects to produce more oil from conventional fields if there was a sufficiently high carbon tax or government subsidies on it. Basically, the only thing that the oil sands produce that doesn't have a market is the sand.
Well, actually you don't need those billion dollar upgraders at all, and the trend is increasingly toward not building them and selling crude bitumen directly to oil refineries. The difficulty is in getting it down the pipelines to the refineries. And "straight out of the ground" is not an accurate description of what they are doing in ND these days. There's a lot of horizontal drilling and frantic fracking involved.
Much of the world's oil is not much different from bitumen these days. Venezuelan Extra-Heavy, Mexican Mayan Heavy, and even Arabian Heavy is not much different in terms of quality and contaminants. The main difference is that they are shipped to the refineries by tanker so they don't run into pipeline limitations and protest groups.
North Dakota oil has something of a niche market in refineries that get or can't process heavy or extra-heavy oil. Their alternatives are West African and Libyan light oil, which is very, very expensive on the world market. Crude bitumen is considered cheap given the cost of alternatives. Most of the big Midwest and Gulf Coast refineries can input crude bitumen direct if it can be delivered to them - and they account for most of US refining capacity.
The refineries on the East and West coasts are in trouble because of their high feedstock costs, so ND oil is helpful in reducing those costs, although maybe not to the point of making a profit.
"Well, actually you don't need those billion dollar upgraders at all, and the trend is increasingly toward not building them and selling crude bitumen directly to oil refineries."
Somewhere, the energy and infrastructure has to be developed. They can do it onsite in Alberta or instead ship raw bitumen out and do it somewhere else. It's all part of society's overall NER regardless of where it happens. There is still a certain amount of processing that must be done on site to get the bitumen out. And if they do further processing off site then the released process gas won't be available to help make NEER 20 to 1!
Interestingly, the N Dakota horizontal wells may have similar magnitude capital hurdles as the oil sand facilities do. The scale of the N Dakota wells would just be much much smaller than a single Alberta upgrader. Furthermore, the profit return for a N Dakota well happens over only a few years after drilling as production falls off so fast. This makes them ripe for Wall Street ponzi scheme funding. Alberta upgraders, on the other hand, are so huge, and they are in profit mode for so many years after construction, that Wall Street shenanigans don't apply there. But it may be that both types of oil extraction may have similar required capital overhead, considering that both are borderline profitable, highly dependent on which way oil prices go.
"Somewhere, the energy and infrastructure has to be developed. They can do it onsite in Alberta or instead ship raw bitumen out and do it somewhere else."
China. The Chinese have already built the refineries, they have the ability to upgrade bitumen on the front end and process it into products which they will sell world-wide. People have no idea how big or how sophisticated the new Chinese oil refineries are. The Chinese have already bought up a large piece of the Canadian and Venezuelan oil sands. The major logistical issue is getting the crude bitumen to China. After that, it's a done deal.
Per the latest IEA reports the conventional oil refining industry in the developed world is toast. If they don't have technology to match the new Chinese refineries, they will get eaten alive.
It's happened in a lot of other industries, why would you expect oil refining to be any different?
Good series of posts RMG:
The railway to Alaska will solve the logistical issue, in due course.
The Alberta Government have finally figured it out that social license is unavailable for pipelines thru BC.
So they took out an Insurance Policy "premium" and have kicked-in some skin-in-the-game.
However, we could still build a few more NW Redwater refineries here in Canada...maybe even in NEBC, near one of the pending 2 or 3 state-of-art methanol facilities slated for north east BC..
stay tuned.
Aside from the national security issue of selling out our country to communists, a rail line to Alaska is probably the best overall way of exporting our
sovereigntybitumen. It would be solid in the rail cars so in case of derailment there would be no spill. However, the extra gas needed to re-liquify it at the terminal would be a further draw on NER. Furthermore, there's still the risk of tanker spills, but those would presumably be less in Valdez than Kitimat, and that's America's problem.This makes a lot of sense. And it's actually the shortest path: a great circle route from Edmonton to Shanghai passes through Fairbanks.
That said, keeping a train running in the Yukon in February is not my idea of a good time.
You make it sound like a such a win-win situation. This is the Paul Krugman / Ben Bernanke / Federal Reserve approach towards how N America's economy should develop. We should artificially prop up the dollar so as to make Chinese manufactured imports cheap. This, coupled with the artificial suppression of the Yuan, causes N America's non-resource extraction industries to shift over to China. Their lax social and environmental regulations also aid this transition. Then, after this, all we N Americans need to do is open up our natural resources to access from China and let the Chinese suck us dry and manufacture the goods for us to consume back! Isn't it so great? We'll all become rich! All we have to do over here is go to work 1) selling those goods China makes, and 2) typing on our computers and brainstorming to come up with new technological ideas we can sell to the Chinese to actually manufacture. The fact that no one here knows anything about technology anymore since those jobs are long gone doesn't seem to factor into the equation.
There isn't ANYTHING that could go wrong with that plan!
Paul Krugman / Ben Bernanke / Federal Reserve approach towards how N America's economy should develop. We should artificially prop up the dollar so as to make Chinese manufactured imports cheap.
I haven't seen anything from Krugman or the Fed arguing for a strong dollar - quite the opposite.
It has to do with the reserve currency status of the dollar. There are conflicting motivations. On one hand, they want the dollar to be low to help with exports. On the other, they want the rest of the world to demand and buy dollars to prop up the US debt machine. They are reaching the point where they cannot have both and the dollar will die.
What have they said or done that supports a strong dollar?
On the other hand, if it were to collapse what currency would replace it?
One of the basic concerns about these operations is their level of CO2 emissions. Their lower EROEI suggests that these concerns are well founded.
Do you have any related analysis of this, or are you aware of anything you feel is relevant?
I have looked extensively at these issues in previous work and a forthcoming paper. The forthcoming data uses this same dataset to construct GHG emissions estimates. Good work elsewhere is by Bergerson et al. from University of Calgary.
Photo gallery: Aerial view of deforestation at the Tar Sands mine in Alberta, Canada
The oil sands are indeed bigger than England, at 140,000 km2 versus 130,395 km2, but the total area which has been mined so far is 715 km2, which is only about half the area of London. The total mineable area is 4,750 km2, or only 3% of the total oil sands area. That's less than 0.2% of Canada's 3 million km2 of boreal forest. If it were somewhere else, like Europe, it might seem like a lot of forest, but in Canada it's just a small smudge on the map.
The pictures of trees stacked up like matchsticks and ready to be hauled to the sawmill illustrate that this area of the boreal forest is actually commercial forest and all destined to be logged off and replanted to trees, over and over again. The mined area will be replanted as well.
Canada has several wilderness parks which are bigger than Switzerland, but this is not one of them. It is probably destined to become a major industrial area with large cities in the future. There is however a wilderness park bigger than Switzerland a few hundred kilometres directly north of the oil sands area - Wood Buffalo National Park, which is a refuge for the wood buffalo, among other rare species.
Excellent and quiet reasonable response to the standard refrain about oil sands development impacts...
But we still could do better....and as you mention we are trying....just never fast enough....
I expect this will change soon.....as the oil patch have really been shaken by the efforts of NGO enviros focus on their shortcomings...
I sense a certain change in attitude .... but it is slow.
Part 1 of a 6 part series from 2008
http://www.theoildrum.com/node/3786
Charlie Hall comments
http://www.theoildrum.com/node/3800
From: An Empirical Perspective on the Energy Payback Time for Photovoltaic Mocules by Karl E. Knapp and Theresa L. Jester, Solar 2000, Madison, June 2000.
Making a solar panel involved 2742 processes using 2857 materials in 2000, and fewer now. At the time of their study the norm for mono-crystalline panels were 200 micron thick cells sawn from mono-crystalline ingot. Today, proton induced exfoilation produces mono-crystalline cells 20 micron thick, with no sawing, and no kerf loss
In 2000, Knapp and Jester found 5713 kwh (including both materials and processing), were spent making 1 kwe of solar panel at 13% efficiency. Today, with proton induced exfoilation, auto cooling, and streamlined processing 1764 kwh are required to make 1 kwe of solar panel with 21% efficiency.
Today's panel has 62% of the area of one built in 2000 to make the same power, and uses 5% of the silicon required back then. Knapp and Jester found an EROEI for PV panels = 7.6 in 2000, and today PV panels EROEI = 24.6, due to thinner silicon cells, streamlined processing, and less area per Kwe.
In Life Cycle Energy Cost of Gas Turbine Power, P.J. Meier and G.L. Kulcinski, Madison, WI,2000, the authors calculated the EROEI of Gas Turbine power, including the cost of extracting and transporting the gas to the plant, constructing the plant, operating same, and decommissioning same. I added the cost of transmission and distribution of the power to their findings. The results are as follows:
Gas at well head EROEI = 7.8
Gas at plant EROEI = 4.6
Net energy of electricity leaving plant EROEI= 1.75
Net energy delivered to consumer EROEI = 1.18
INDY
Today, with proton induced exfoilation, auto cooling, and streamlined processing 1764 kwh are required to make 1 kwe of solar panel with 21% efficiency. Today's panel has 62% of the area of one built in 2000 to make the same power, and uses 5% of the silicon required back then.
Could you provide sources for this data?
Many thanks Goprisko, I found that really interesting. It's great news that the energy required to make PV cells has come down so much over the years - as I would have expected of course, but it's good to know a bit about how and why it happened. On the other hand I'm trying to make sense of the EROI number of 24.6 that you quoted based on energy required to manufacture the cell of 1764 kWh.
I guess the EROI of PV depends entirely on where and how well the panel is erected, but experience in Germany shows an average of about 7 - 10% load factor for PV i.e. a 1 kW panel running all year (8760 hours) would be expected to generate between 613 and 876 kWh per year of power. I know Germany is not the sunniest place in the world, but it is a place that produces good statistics and has over 32 GW of installations to look at so I think that those are good load factors to use.
So anyway, if the 1kW cell cost 1,764 kW to make and gives back 24.6 times that in energy over its life, at a load factor of 10% (at the optimistic end) it must be operating for 1,764 * 24.6 /0.1 hours of operation or 433,944 hours, which is just under 50 years (49.54 to be precise). Is this realistic?
Also, in order to get to this I have had to assume zero energy cost of installing the PV modules (or maintaining them), are installation effects really negligable? After all you've got men driving around in little white vans burning gallons of diesel fuel at a time, often for installing only a few kW of power at a time. I've also had to assume no degradation in power output, whereas I had understood this was quite significant after the first decade or so of operation.
Is this an idealised or real life EROI for PV?
10% is too low - 15% is reasonable, and there's more than enough land with insolation of 20% to provide all the power needed. Germans are determined to produce their power domestically, but that's not a decision driven by normal engineering economics.
PV apears to decline at about .5% per year, and existing panels are well on their way to 50 year lifespans. "Rocks that produce power".
And, don't forget that much of the input energy is lower quality than the output: electricity (and reasonably near peak demand).
Nick, I am using real statistics from Germany, although I have to admit I took the figures from Wikipedia (http://en.wikipedia.org/wiki/Solar_power_in_Germany) which is not always the most reliable source. If you have more a more reliable source please provide it.
The Germans have installed over 30 GW of PV including a mix of residential scale and large arrays, so we can start get a picture of what real world (not idealised) deployment actually looks like.
I looked at the last 9 years - from the time that installed capacity broke the 1 GW level and found load factors of between 5.74% and 9.79%. However, I was worried that load factors were being skewed because the capacity figures were quoted as of year end whereas the generation was through the year, meaning that arrays installed during the year were not having the chance to fully contribute. Therefore, for the purpose of calculating load factor I adjusted the capacity figure down by 50% of the capacity additions in that year, i.e. assuming that on average capacity additions were made half way through the year. The adjusted results showed a high of 11.08% and a low of 8.24%, an average of 9.72% and a median of 9.81% for the 9 year period.
Actually what I found most interesting about the data (albeit 9 years was not a great sample size) was that there was actually a trend of rising load factors, even on the adjusted basis, fitting to a slope of 0.21% per year with an intercept of 8.24% in year 0 (2004). The correlation coefficient was 0.68 so not a conclusive trend, but still interesting, especially since I would have predicted the opposite - i.e. that the sunniest spots and the most southerly facing roofs would get their PV first and then installation would move on to poorer sites. I wonder whether this reflects a change in weighting of capacity installations over the years from residential roof mounted (not optimally aligned, and sometimes shaded) to dedicated ground based arrays (usually optimally aligned and negligibly shaded) - perhaps driven by the subsidy regime. That is certainly what we're seeing in the UK.
Still 15% load factors look too optimistic for PV right now, but perhaps we will get there in the end with better placed arrays. I'd like to know more about the transport and installation costs, but on a fag packet calc I can believe they might be as low as 100 kWh per kW.
Assuming a 50 year life, and using a 10% LF, 0.5% degradation and 1864 kWh investment cost (including 100 kWh for transport, installation and inverter costs) that gives an internal rate of energy return of 46%, which is pretty good and much better than corn ethanol, but still nowhere near as good as solid biomass energy crop for power generation which has an IRER in the hundreds (and nuclear fission is probably in the thousands).
Land availability constrains biomass and therefore PV should clearly be used as well, but the best outcome is to do both i.e. give land it's most appropriate use - PV when closer to centres of demand (and especially on roofs if well aligned), biomass on appropriate land - usually degraded or poor quality agricultural soils with low biodiversity. The two are also complimentary since biomass can be stored and used to match the demand profile minus the PV production curve (and minus any nuclear baseload).
Nick, I am using real statistics from Germany,
Germany is a worst-case scenario for PV power: high latitude and lots of clouds. Nick seems to have anticipated this by pointing out that Germany is installing PV within its borders for sociopolitical reasons, not because it makes engineering sense.
Not quite worse case, deployment is heavily weighted towards Bavaria and Southern Germany which is getting around 1,300 kWh per m2 per year horizontal irradiation. Also, at least Germany has a daytime peak in demand. UK peak electricity demand is always after dark which means PV contributes exactly zero to capacity, and our annual irradiation is much less as well.
But anyway, I take your point that there are certainly far better countries for PV than Germany. However you can't just choose the best location, work out your energy returns based on that, and then present them as if they were universal. It would be valid to present a range or - if a single number is prefered - then it should be based on a weighted average of installed capacity - which is globally dominated by Germany.
Not quite worse case, deployment is heavily weighted towards Bavaria and Southern Germany which is getting around 1,300 kWh per m2 per year horizontal irradiation.
By comparison, that's roughly the same as Seattle, the worst spot in the continental US.
However you can't just choose the best location, work out your energy returns based on that, and then present them as if they were universal.
You can, though, within reason. Existing long-haul electrical transmission is incredibly efficient -- less than 10% loss per 1000 km with 1960s technology, less than 5% with modern HVDC systems -- and very cheap compared to the panels themselves. There is no engineering reason not to build solar systems where the sun shines, and ship the power where it's needed.
It would be valid to present a range or - if a single number is prefered - then it should be based on a weighted average of installed capacity - which is globally dominated by Germany.
No, when planning for the future, one should consider how resources can reasonably be used in the future, not where they have been developed in the past. We're trying to change the world here: we can't take past as prologue! (But we do have to obey the laws of physics.)
http://www.nrel.gov/gis/images/us_germany_spain/pvmap_usgermanyspain%20p...
http://www.siemens.com/press/pool/de/pressemitteilungen/2012/energy/powe...
Germany had installed 35.6 GW PV end of 2012. According to AG Energiebilanzen, that is the source you use or argue against :-), these 32.6 GW produced around 28 TWh electricity. Therefore, we have at least 860 kWh per kW.
That works out to 9.0 percent, in good agreement with Hoover's numbers.
http://www.wolframalpha.com/input/?i=28+terawatt+hours+%2F+%2835.6+gigwa...
However you can't just choose the best location
Well, what question are we trying to answer with E-ROI calculations?? If the questions is: "Can PV provide a large fraction of our energy needs with a high E-ROI?", then the answer can and should use the best resource that is practically available. For instance, Spain can achieve 20%: it can and should take the lead, and export quite a lot of power to the rest of Europe.
If you're willing to accept continued dependence on imports to Europe (and why not?), then you should use Morocco, which should be able to achieve a 25% capacity factor fairly easily!
"Solar power in Morocco is enabled by one of the highest rates of solar insolation of any country — about 3,000 hours per year of sunshine. Morocco has launched one of the world’s largest solar energy projects costing an estimated $9 billion. The aim of the project is to generate 2,000 megawatts of solar generation capacity by the year 2020.[1] Five solar power stations are to be constructed. The Moroccan Agency for Solar Energy (MASEN), a public-private venture, has been established to lead the project. The first plant will be commissioned in 2015,[2] and the entire project in 2019. Once completed, the solar project will provide 18% of Morocco’s annual electricity generation.
Desertec - Morocco, the only African country to have a power cable link to Europe, aims to benefit from the €400bn ($573.8bn) expected to come from the ambitious pan-continental Desertec Industrial Initiative."
http://en.wikipedia.org/wiki/Solar_power_in_Morocco
In 2000, Kapp and Jester delivered " An Empirical Perspective on the Energy Payback Time for Photovoltaic Mocules " at Solar 2000. If you google the title, you can download their presentation yourself.
They found the EROEI of a PV panel, as delivered to be 7.8.
At that time the efficiency of Mono-crystalline PV was ~ 13% or 130w/m2. Today, manufacturers in
China are delivering PV with an efficiency of 21% or 210 w/m2.
At that time, wafers were made by wire sawing them from mono-crystalline Silicon Ingots.
Since that time, fabricators have learned how to saw thinner wafers, and as I posted elsewhere, Twin Creeks and others developed the proton-induced exfoilation process. Also, as I posted elsewhere, Evergreen discovered a process for making 100 micron ribbon from a Silicon melt, and SRI developed a process for making mono-crystalline Silicon from Phosphate mine waste.
Since Silicon is the largest energy component in a PV panel, with nearly 66% of all energy consumed in providing it, any technique which reduces the amount of silicon in the panel gives marked reductions in the energy content of the panel.
Proton-induced exfoilation permits fabrication of wafers 20 microns thick vs previous 200 micron wafers, and eliminates sawing, kerf loss, cutting oils, carbide, and other materials associated with sawing.
The combination of reduced area/watt and thinner wafers, raises the EROEI of a PV panel FOB plant from 7.8 in 2000 to ~ 25 today, per my own calculations based upon this data.
I think that leveling the playing field for the various energies requires determination of EROEI for petroleum and gas at the wellhead/minemouth and for wind and PV FOB plant. I advocate this because neither of the aforementioned sources are usable at that point. Hydrocarbons must be transported and refined/purified before use, and wind/PV must be transported and erected/installed.
Given most publications mention the EROEI of hydrocarbons at the wellhead/mine mouth, my mentioning the EROEI of PV FOB plant provides a comparable baseline.
That said, other work I posted has shown the EROEI of Natural Gas fired electrical power to be ~1.2 at the consumers meter.
In your planning for doom and gloom, it is imperative that you inculcate current values, in a fast changing industry.
I have other work I would like to post, and I am very interested in collaborating with those serious enough to help me audit the spreadsheets and their calculations, in which it appears the US can use existing technologies to transition from an economy using ~100 Quads/yr to one using ~10 Quads/yr.
And none of it is rocket science!
INDY
I posted a detailed reply,
Which the moderators do not wish you to see.
INDY
Thirteen years ago, at Solar 2000, Knapp and Jester presented: “An Empirical Perspective on the Energy Payback Time for Photovoltaic Modules” at the Madison, WI conference in June of that year.
They presented a rigorous analysis of the 2857 materials and 2742 processes necessary to construct a PV panel at that time.
In 2000, 200 micron thick wafers were wire-sawn from mono-crystalline ingot, then processed into cells, subsequently joined into panels.
They determined that 2,856 Kwh of energy per Kwe of PV panel was represented by the materials used, and 2742 Kwh per Kwe of PV panel was represented by the energy involved in processing, for a total of 5598 Kwh/kwe.
Mono-crystalline PV panel efficiencies were 13% at that time, and power densities were 130w/m2.
They found that mono-crystalline PV panels returned the energy required to make them within 2-4 years, depending upon where emplaced.
I revisited their EROEI calculation using production data from White Co., Indiana, just a few miles north of Purdue University where I earned my BS in 1968. Using a 40 year life, 0.5% per year degradation, and 130 watts rated output per m2, I calculated an EROEI using their data of ~ 10.1. Using a 30 year life gave an EROEI of ~8, FOB plant.
There have been many process improvements in the solar industry since then. Power densities of 210w/m2, can be found in the high performance mono-crystalline PV panels currently available from China, for example.
The SRI process which makes mono-crystalline Silicon Ingots from Phosphate mine waste, the Evergreen process which makes 100 micron ribbon directly from Silicon melt, and Twin Creek's proton-induced exfoilation method which spalls off 20 micron wafers from mono-crystalline Silicon, plus process efficiencies, have all contributed to reductions in energy inputs.
Because Silicon was 66% of material inputs, when 200 micron wire-sawn wafers were the norm at the time of the Knapp and Jester study, any changes which reduce the amount of Silicon in the panel have a very large effect.
Because wire sawing imposes kerf losses, and involves a great deal of material and energy, eliminating wire sawing altogether has a major impact too.
The Twin Creek proton-induced exfoilation process reduces the silicon required by 93%, through elimination of kerf loss, and reduction of thickness from 200 microns to 20 microns.
This new method of making wafers from mono-crystalline Silicon Ingot, plus efficiency gains in panel output from 13% to 21%, reduce inputs to 1,774 kwh/kwe, a 68% reduction since the Knapp study.
Using these values, a PV panel located in White Co., Indiana at a non-shaded location, tilted toward the equator at an angle equal to it's latitude, producing 1564 kwh/kwe-yr, has an EROEI, FOB plant of ~32, given 0.5% per year degradation over a 40 year life, by the end of which it will be producing 82% of its capacity when new.
I am of the opinion that a valid point for EROEI comparison is at wellhead/minemouth for hydrocarbons, and FOB plant for PV/wind. I believe this because, final customers can be anywhere, with concomitant transport energy inputs. Usage efficiencies can vary enormously depending upon the conversion equipment used for hydrocarbons, the wind resource of the wind farm, or the solar resource where the PV array is located.
INDY
Posted in error
There is an error in the caption for Figure 2.
Figure 2. System diagram for
mining and upgradingpathways showing primary product...Figure 2. System diagram for in situ thermal production pathways showing primary product...
Good catch!
An article in an Australian newspaper says the Earth will become too hot for humans in less than 300 years. Search Too hot to live: grim long-term prediction. I guess we'll just have to turn up the air conditioning.
I suggest after 2050 (when today's kids will be middle aged) all fossil fuels with EROEI of 4 or greater will be depleted. Suppose oil, gas and unconventional are effectively gone leaving only inferior or less accessible coal. If low carbon alternatives don't arrive in time up we'll need that coal to make both electricity and liquid fuel, shame about the molten ice caps. However I think negative economic feedback processes could stop low EROEI coal extraction before that whether cleantech arrives or not. Think lack of capital, high unemployment, wars, climate woes, food shortages and the like.
Therefore in the absence of a Venus style runaway greenhouse event I don't think humans will knowingly make the Earth uninhabitable. By mistake maybe. The key reason is declining EROEI coupled to economic degrowth.
Dr. Guy MacPherson did his homework and if his analysis is correct you are an order of magnitude too long.
Given the current 400 ppm of CO2, the equilibrium acidity of the oceans is sufficient to dissolve the shells of molluscs, and the
skeletons of corals, making both groups extinct within decades.
Then there is the small problem of plankton extinction, which will kill all pelagic organisms, which will interfere with oxygenation of the atmosphere, leading to reductions in O2 levels.
Then there is widespread drought in the western states.
A whole host of problems, we are ill adapted for.
BTW, did you read my post?
Do you understand that we could transition to a renewable economy now?
If we had leadership, that is.
INDY